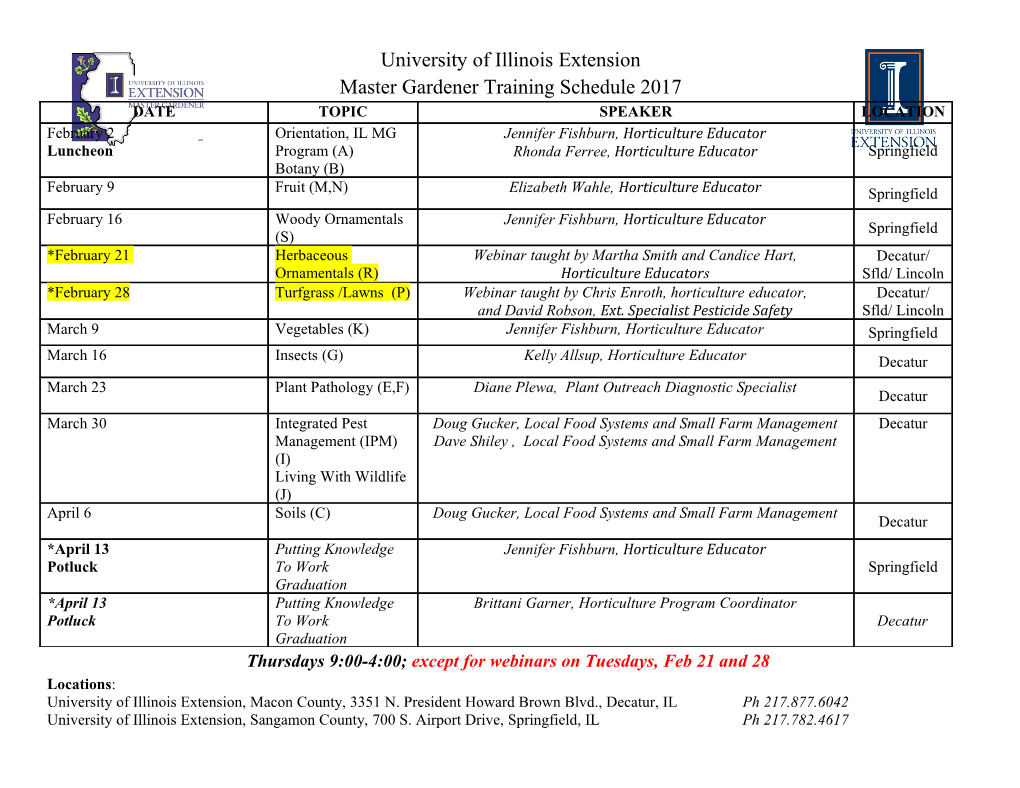
Multivector differentiation and Linear Algebra 17th Santalo´ Summer School 2016, Santander Joan Lasenby Signal Processing Group, Engineering Department, Cambridge, UK and Trinity College Cambridge [email protected], www-sigproc.eng.cam.ac.uk/ s jl 23 August 2016 1 / 78 Examples of differentiation wrt multivectors. Linear Algebra: matrices and tensors as linear functions mapping between elements of the algebra. Functional Differentiation: very briefly... Summary Overview The Multivector Derivative. 2 / 78 Linear Algebra: matrices and tensors as linear functions mapping between elements of the algebra. Functional Differentiation: very briefly... Summary Overview The Multivector Derivative. Examples of differentiation wrt multivectors. 3 / 78 Functional Differentiation: very briefly... Summary Overview The Multivector Derivative. Examples of differentiation wrt multivectors. Linear Algebra: matrices and tensors as linear functions mapping between elements of the algebra. 4 / 78 Summary Overview The Multivector Derivative. Examples of differentiation wrt multivectors. Linear Algebra: matrices and tensors as linear functions mapping between elements of the algebra. Functional Differentiation: very briefly... 5 / 78 Overview The Multivector Derivative. Examples of differentiation wrt multivectors. Linear Algebra: matrices and tensors as linear functions mapping between elements of the algebra. Functional Differentiation: very briefly... Summary 6 / 78 We now want to generalise this idea to enable us to find the derivative of F(X), in the A ‘direction’ – where X is a general mixed grade multivector (so F(X) is a general multivector valued function of X). Let us use ∗ to denote taking the scalar part, ie P ∗ Q ≡ hPQi. Then, provided A has same grades as X, it makes sense to define: F(X + tA) − F(X) A ∗ ¶XF(X) = lim t!0 t The Multivector Derivative Recall our definition of the directional derivative in the a direction F(x + ea) − F(x) a·r F(x) = lim e!0 e 7 / 78 Let us use ∗ to denote taking the scalar part, ie P ∗ Q ≡ hPQi. Then, provided A has same grades as X, it makes sense to define: F(X + tA) − F(X) A ∗ ¶XF(X) = lim t!0 t The Multivector Derivative Recall our definition of the directional derivative in the a direction F(x + ea) − F(x) a·r F(x) = lim e!0 e We now want to generalise this idea to enable us to find the derivative of F(X), in the A ‘direction’ – where X is a general mixed grade multivector (so F(X) is a general multivector valued function of X). 8 / 78 The Multivector Derivative Recall our definition of the directional derivative in the a direction F(x + ea) − F(x) a·r F(x) = lim e!0 e We now want to generalise this idea to enable us to find the derivative of F(X), in the A ‘direction’ – where X is a general mixed grade multivector (so F(X) is a general multivector valued function of X). Let us use ∗ to denote taking the scalar part, ie P ∗ Q ≡ hPQi. Then, provided A has same grades as X, it makes sense to define: F(X + tA) − F(X) A ∗ ¶XF(X) = lim t!0 t 9 / 78 With the definition on the previous slide, eJ ∗ ¶X is therefore the partial derivative in the eJ direction. Giving J ¶X ≡ ∑ e eJ ∗ ¶X J I [since eJ ∗ ¶X ≡ eJ ∗ fe (eI ∗ ¶Xg]. Key to using these definitions of multivector differentiation are several important results: The Multivector Derivative cont... Let feJg be a basis for X – ie if X is a bivector, then feJg will be the basis bivectors. 10 / 78 Key to using these definitions of multivector differentiation are several important results: The Multivector Derivative cont... Let feJg be a basis for X – ie if X is a bivector, then feJg will be the basis bivectors. With the definition on the previous slide, eJ ∗ ¶X is therefore the partial derivative in the eJ direction. Giving J ¶X ≡ ∑ e eJ ∗ ¶X J I [since eJ ∗ ¶X ≡ eJ ∗ fe (eI ∗ ¶Xg]. 11 / 78 The Multivector Derivative cont... Let feJg be a basis for X – ie if X is a bivector, then feJg will be the basis bivectors. With the definition on the previous slide, eJ ∗ ¶X is therefore the partial derivative in the eJ direction. Giving J ¶X ≡ ∑ e eJ ∗ ¶X J I [since eJ ∗ ¶X ≡ eJ ∗ fe (eI ∗ ¶Xg]. Key to using these definitions of multivector differentiation are several important results: 12 / 78 We can see this by going back to our definitions: h(X + teJ)Bi − hXBi hXBi + theJBi − hXBi eJ ∗ ¶XhXBi = lim = lim t!0 t t!0 t theJBi lim = heJBi t!0 t Therefore giving us J J ¶XhXBi = e (eJ ∗ ¶X)hXBi = e heJBi ≡ PX(B) The Multivector Derivative cont... If PX(B) is the projection of B onto the grades of X (ie J PX(B) ≡ e heJBi), then our first important result is ¶XhXBi = PX(B) 13 / 78 theJBi lim = heJBi t!0 t Therefore giving us J J ¶XhXBi = e (eJ ∗ ¶X)hXBi = e heJBi ≡ PX(B) The Multivector Derivative cont... If PX(B) is the projection of B onto the grades of X (ie J PX(B) ≡ e heJBi), then our first important result is ¶XhXBi = PX(B) We can see this by going back to our definitions: h(X + teJ)Bi − hXBi hXBi + theJBi − hXBi eJ ∗ ¶XhXBi = lim = lim t!0 t t!0 t 14 / 78 Therefore giving us J J ¶XhXBi = e (eJ ∗ ¶X)hXBi = e heJBi ≡ PX(B) The Multivector Derivative cont... If PX(B) is the projection of B onto the grades of X (ie J PX(B) ≡ e heJBi), then our first important result is ¶XhXBi = PX(B) We can see this by going back to our definitions: h(X + teJ)Bi − hXBi hXBi + theJBi − hXBi eJ ∗ ¶XhXBi = lim = lim t!0 t t!0 t theJBi lim = heJBi t!0 t 15 / 78 The Multivector Derivative cont... If PX(B) is the projection of B onto the grades of X (ie J PX(B) ≡ e heJBi), then our first important result is ¶XhXBi = PX(B) We can see this by going back to our definitions: h(X + teJ)Bi − hXBi hXBi + theJBi − hXBi eJ ∗ ¶XhXBi = lim = lim t!0 t t!0 t theJBi lim = heJBi t!0 t Therefore giving us J J ¶XhXBi = e (eJ ∗ ¶X)hXBi = e heJBi ≡ PX(B) 16 / 78 ¶XhXBi = PX(B) ¶XhXB˜ i = PX(B˜ ) ˜ ¶X˜ hXBi = PX˜ (B) = PX(B) −1 −1 −1 ¶yhMy i = −y Py(M)y X, B, M, y all general multivectors. Other Key Results Some other useful results are listed here (proofs are similar to that on previous slide and are left as exercises): 17 / 78 Other Key Results Some other useful results are listed here (proofs are similar to that on previous slide and are left as exercises): ¶XhXBi = PX(B) ¶XhXB˜ i = PX(B˜ ) ˜ ¶X˜ hXBi = PX˜ (B) = PX(B) −1 −1 −1 ¶yhMy i = −y Py(M)y X, B, M, y all general multivectors. 18 / 78 Exercises 1 1 By noting that hXBi = h(XB)˜i, show the second key result ¶XhXB˜ i = PX(B˜ ) 2 ˜ Key result 1 tells us that ¶X˜ hXBi = PX˜ (B). Verify that PX˜ (B) = PX(B), to give the 3rd key result. 3 to show the 4th key result −1 −1 −1 ¶yhMy i = −y Py(M)y −1 use the fact that ¶yhMyy i = ¶yhMi = 0. Hint: recall that XAX has the same grades as A. 19 / 78 One possible way forward is to find the plane that minimises the sum of the squared perpendicular distances of the points from the plane. X2 X3 X1 X0 1 X0 0 2 X3 A Simple Example Suppose we wish to fit a set of points fXig to a plane F – where the Xi and F are conformal representations (vector and 4 vector respectively). 20 / 78 A Simple Example Suppose we wish to fit a set of points fXig to a plane F – where the Xi and F are conformal representations (vector and 4 vector respectively). One possible way forward is to find the plane that minimises the sum of the squared perpendicular distances of the points from the plane. X2 X3 X1 0 X1 0 X2 X0 3 21 / 78 Now use the result ¶XhXBi = PX(B) to differentiate this expression wrt F ˙ ˙ ˙ ˙ ¶FS = − ∑ ¶FhXiFXiFi = − ∑ ¶FhXiFXiFi + ¶FhXiFXiFi i i = −2 ∑ PF(XiFXi) = −2 ∑ XiFXi i i =) solve (via linear algebra techniques) ∑i XiFXi = 0. Plane fitting example, cont.... Recall that FXF is the reflection of X in F, so that −X·(FXF) is the distance between the point and the plane. Thus we could take as our cost function: S = − ∑ Xi·(FXiF) i 22 / 78 = −2 ∑ PF(XiFXi) = −2 ∑ XiFXi i i =) solve (via linear algebra techniques) ∑i XiFXi = 0. Plane fitting example, cont.... Recall that FXF is the reflection of X in F, so that −X·(FXF) is the distance between the point and the plane. Thus we could take as our cost function: S = − ∑ Xi·(FXiF) i Now use the result ¶XhXBi = PX(B) to differentiate this expression wrt F ˙ ˙ ˙ ˙ ¶FS = − ∑ ¶FhXiFXiFi = − ∑ ¶FhXiFXiFi + ¶FhXiFXiFi i i 23 / 78 =) solve (via linear algebra techniques) ∑i XiFXi = 0. Plane fitting example, cont.... Recall that FXF is the reflection of X in F, so that −X·(FXF) is the distance between the point and the plane. Thus we could take as our cost function: S = − ∑ Xi·(FXiF) i Now use the result ¶XhXBi = PX(B) to differentiate this expression wrt F ˙ ˙ ˙ ˙ ¶FS = − ∑ ¶FhXiFXiFi = − ∑ ¶FhXiFXiFi + ¶FhXiFXiFi i i = −2 ∑ PF(XiFXi) = −2 ∑ XiFXi i i 24 / 78 Plane fitting example, cont.... Recall that FXF is the reflection of X in F, so that −X·(FXF) is the distance between the point and the plane.
Details
-
File Typepdf
-
Upload Time-
-
Content LanguagesEnglish
-
Upload UserAnonymous/Not logged-in
-
File Pages78 Page
-
File Size-