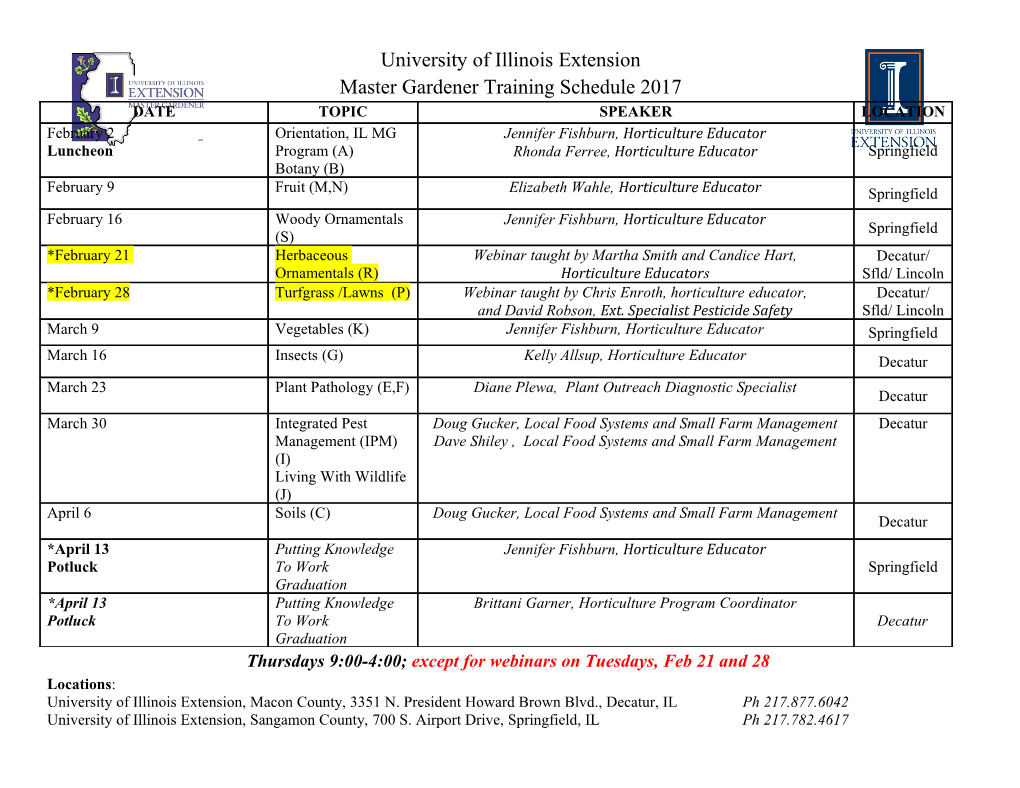
MOLECULAR PHYLOGENETICS AND EVOLUTION Molecular Phylogenetics and Evolution 25 (2002) 361–371 www.academicpress.com Phylogenetic relationships of the dwarf boas and a comparison of Bayesian and bootstrap measures of phylogenetic support Thomas P. Wilcox, Derrick J. Zwickl, Tracy A. Heath, and David M. Hillis* Section of Integrative Biology and Center for Computational Biology and Bioinformatics, The University of Texas at Austin, Austin, TX 78712, USA Received 4 February 2002; received in revised form 18 May 2002 Abstract Four New World genera of dwarf boas (Exiliboa, Trachyboa, Tropidophis, and Ungaliophis) have been placed by many syste- matists in a single group (traditionally called Tropidophiidae). However, the monophyly of this group has been questioned in several studies. Moreover, the overall relationships among basal snake lineages, including the placement of the dwarf boas, are poorly understood. We obtained mtDNAsequence data for 12S, 16S, and intervening tRNA–valgenes from 23 species of snakes repre- senting most major snake lineages, including all four genera of New World dwarf boas. We then examined the phylogenetic position of these species by estimating the phylogeny of the basal snakes. Our phylogenetic analysis suggests that New World dwarf boas are not monophyletic. Instead, we find Exiliboa and Ungaliophis to be most closely related to sand boas (Erycinae), boas (Boinae), and advanced snakes (Caenophidea), whereas Tropidophis and Trachyboa form an independent clade that separated relatively early in snake radiation. Our estimate of snake phylogeny differs significantly in other ways from some previous estimates of snake phy- logeny. For instance, pythons do not cluster with boas and sand boas, but instead show a strong relationship with Loxocemus and Xenopeltis. Additionally, uropeltids cluster strongly with Cylindrophis, and together are embedded in what has previously been considered the macrostomatan radiation. These relationships are supported by both bootstrapping (parametric and nonparametric approaches) and Bayesian analysis, although Bayesian support values are consistently higher than those obtained from nonpara- metric bootstrapping. Simulations show that Bayesian support values represent much better estimates of phylogenetic accuracy than do nonparametric bootstrap support values, at least under the conditions of our study. Ó 2002 Elsevier Science (USA). All rights reserved. Keywords: Bayesian analysis; Bootstrapping; Phylogenetic support; Phylogeny; Dwarf boas; Snakes; Serpentes; Tropidophiidae; Ungaliophiidae; Booidea; Macrostomata; Caenophidea; Exiliboa; Trachyboa; Tropidophis; Ungaliophis 1. Introduction Part of the uncertainty surrounding the relationships of dwarf boas is a result of the poor understanding of Four New World genera of dwarf boas (Exiliboa, basal snake lineages in general. Most recent authors Trachyboa, Tropidophis, and Ungaliophis) traditionally support a primary division in snakes between Scoleco- have been placed in a single group of snakes, the phidea (the blind snakes) and Alethinophidea (all other Tropidophiidae. About 21 species of these small terres- snakes), and most authors also recognize the groups trial and arboreal snakes are distributed in the West Macrostomata, Caenophidea, and Colubroidea (Fig. 1a). Indies (Greater Antilles and Bahama Islands) and in However, there is considerable disagreement among au- isolated areas of Central and South America. The biol- thors about the relationships within Macrostomata. ogy of species in this group is very poorly known, and Some authors (Cundall et al., 1993; Groombridge, 1979; the phylogenetic relationships of dwarf boas to other Rieppel, 1988) recognize various subsets of Loxocemus, snakes are not well resolved. Xenopeltis, Boinae, Erycinae, Pythonidae, Bolyeriidae, and Tropidophiidae as a monophyletic Booidea (or Henophidia), whereas other evidence (e.g., Kluge, 1991, * Corresponding author. Fax: +1-512-471-3878. 1993) suggests that the ‘‘booids’’ are a paraphyletic group E-mail address: [email protected] (D.M. Hillis). of basal macrostomatans. Several recent summaries of 1055-7903/02/$ - see front matter Ó 2002 Elsevier Science (USA). All rights reserved. PII: S1055-7903(02)00244-0 362 T.P. Wilcox et al. / Molecular Phylogenetics and Evolution 25 (2002) 361–371 Fig. 1. Hypothesized relationships among the major snake Lineages. (a) Traditional phylogeny after Greene (1997). (b) Traditional phylogeny as modified by Zaher (1994). Taxa in bold were traditionally placed in the Tropidophiidae. snake phylogeny have suggested that dwarf boas (all or a Specifically, we wished to test the following three hy- subset) are relatively closely related to Caenophidea—the potheses suggested by Zaher (1994): group that includes most of world’s snakes, including 1. Exiliboa and Ungaliophis form a monophyletic group colubrids, elapids, and viperids (Greene, 1997; Kluge, (Ungaliophiidae), and are not closely related to Trop- 1991; Pough et al., 1998). Nonetheless, as noted by Pough idophiidae sensu Zaher (Trachyboa and Tropidophis). et al. (1998), all alternative phylogenetic hypotheses for 2. Trachyboa and Tropidophis together form the sister- basal snakes are weakly supported at present. group of Caenophidea. Even the monophyly of Tropidophiidae is not well 3. Exiliboa and Ungaliophis are part of a monophyletic supported. Dessauer et al. (1987) presented immuno- Booidea that includes boines, erycines, and pytho- logical data that suggested that tropidophiids were not nids, but not Loxocemus or Xenopeltis. monophyletic. Zaher (1994) argued on the basis of In testing these phylogenetic hypotheses, we com- morphological evidence that Trachyboa and Tropidophis pared several commonly used methods for assessing form the sister-group to Caenophidea, but that Exiliboa phylogenetic support. To test our specific a priori hy- and Ungaliophis are more closely related to other groups potheses, we used parametric bootstrapping to perform that he considered part of Booidea (erycines, boines, likelihood-ratio tests of the alternative hypotheses pythonids, and bolyeriids; Fig. 1b). As a result, Zaher (Goldman et al., 2000; Hillis et al., 1996b; Huelsenbeck (1994) placed Exiliboa and Ungaliophis in Ungaliophii- et al., 1996). However, we also assessed support for dae, and considered Tropidophiidae to contain only other groups that we found during the course of our Trachyboa and Tropidophis (Fig. 1b). study. The two methods that we used for this purpose, To address the relationships of the dwarf boas we nonparametric bootstrapping (Felsenstein, 1985) and obtained sequences from three mitochondrial genes (12S Bayesian analysis (Larget and Simon, 1999; Rannala and 16S ribosomal RNAgenes, and the valine tRNA and Yang, 1996), supported the same groups. However, gene) from representatives of Exiliboa, Ungaliophis, the Bayesian support values were consistently higher Trachyboa, and four species of Tropidophis, as well as than the values obtained from bootstrapping—a pattern from representatives of most of the snake families. that we, and others, have noticed across several phylo- T.P. Wilcox et al. / Molecular Phylogenetics and Evolution 25 (2002) 361–371 363 genetic analyses (e.g., Buckley et al., 2002; Leache and nested primers (Fig. 2), we then amplified approximately Reeder, 2002; Whittingham et al., 2002). Nonparametric 1.9 kb of mitochondrial DNA, spanning portions of the bootstrapping is known to produce highly conservative 12S and 16S genes and the intervening valine–tRNA estimates of phylogenetic accuracy (Hillis and Bull, (corresponding to positions 2475–4574 in the complete 1993). To assess if Bayesian support values are better mitochondrial sequence of Xenopus laevis [GB M10217]). measures of phylogenetic accuracy than those obtained Standard PCR conditions were used (Palumbi, 1996) through nonparametric bootstrapping, we conducted a with the following thermal cycle profile: 2 min at 94 °C, simulation study using our estimated snake phylogeny followed by 35 cycles of: (94 °C for 30 s, 42 °C for 30 s, as a model tree. and 72 °C for 1 min). Amplification products were puri- fied from agarose gel slices using the Qiaquick Gel Ex- traction Kit (Qiagen) and sequenced using fluorescent 2. Materials and methods thermal cycle sequencing and an ABI 377 automated sequencer (Perkin–Elmer). 2.1. Samples, DNA extraction, and sequencing 2.2. Phylogenetic analyses We obtained tissues (liver, muscle, or blood) from 23 species of snakes, representing most of major snake lin- Sequences were aligned using Clustal W (Thompson eages (Greene, 1997): Acrochordus javanicus (UTA et al., 1994) and adjusted to accommodate conserved 17064), Anilius scytale (LSUMNS H-8033), Azemiops secondary structures (Cannone, 2002; http://www.rna. feae (UTA18701), Boa constrictor (UTA24752), Cro- icmb.utexas.edu/). Aligned sequences were analyzed talus polystictus (UTA14514), Cylindrophis ruffus (UTA with PAUP* (version 4.0b8; Swofford, 2000) using 24902), Eryx conicus (TNHC 61448), Exiliboa placata maximum-likelihood and a GTR þ C þ PINVAR (UTA12233), Leptotyphlops dulcis (TNHC 61447), model of sequence evolution with four C-distributed rate Loxocemus bicolor (UTA14494), Morelia boeleni (UTA classes (for parameter values see Appendix B) (Swofford 11396), Pituophis lineaticolis (UTA25116), Rhinophis et al., 1996). This model of sequence evolution was philippinus (LSUMNS H-6179), Trachyboa boulengeri chosen based on results from Modeltest (v3.06, Posada (UTA12958), Tropidophis feicki (POE 89),
Details
-
File Typepdf
-
Upload Time-
-
Content LanguagesEnglish
-
Upload UserAnonymous/Not logged-in
-
File Pages11 Page
-
File Size-