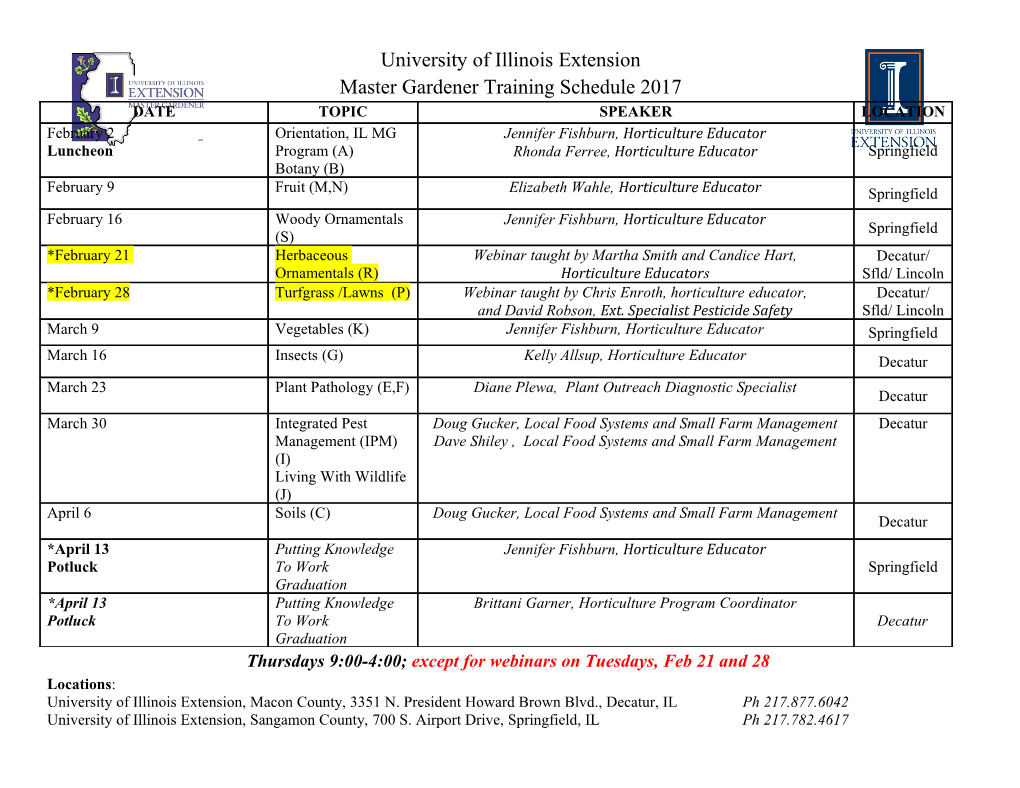
This is an open access article published under a Creative Commons Attribution (CC-BY) License, which permits unrestricted use, distribution and reproduction in any medium, provided the author and source are cited. pubs.acs.org/EF Article Synergistic Effects of Sodium Iodide and Sodium Dodecyl Sulfate at Low Concentrations on Promoting Gas Hydrate Nucleation Fariba Asadi, Ngoc N. Nguyen,* and Anh V. Nguyen* Cite This: Energy Fuels 2020, 34, 9971−9977 Read Online ACCESS Metrics & More Article Recommendations ABSTRACT: Surfactants and/or salts at low concentrations are inherently relevant to gas hydrate formation in nature and industry. However, the combined effects of surfactants and salts at low concentrations on gas hydrate formation are poorly understood. Here, we aim to fill this gap of knowledge by examining the effects of sodium dodecyl sulfate (SDS) and sodium iodide (NaI) at millimolar concentrations on methane hydrate formation. We show that adding NaI to dilute SDS solutions reduces the induction time significantly, promoting gas hydrate nucleation. Meanwhile, adding NaI adversely reduces the growth of methane hydrate. Fundamental studies based on sum frequency generation spectroscopy indicate an electrostatically aligned water layer at the gas− SDS solution interface caused by the charged interface as a result of surface adsorption of dodecyl sulfate anions. This aligned interfacial water layer hinders the nucleation of gas hydrate at the gas−SDS solution interface. Added NaI diminishes the alignment of interfacial water, thereby favoring the nucleation of the gas hydrate. Even though, the adverse (inhibiting) effect of added NaI on the methane hydrate growth remains a puzzling observation that requires further investigations. This study sheds more light on gas hydrate formation in surfactant- and salt-containing systems that are important to many phenomena in nature and applications in the industry. 1. INTRODUCTION formation by a factor greater than 700.28 Meanwhile, inorganic Gas hydrates are crystalline solids composed of water and salts are well-known hydrate formation inhibitors. Fundamen- hydrophobic (nonpolar) gas molecules. Water molecules form tally, it is believed that surfactant micelles are responsible for an ice-like structure via hydrogen bonding called host increasing hydrate formation kinetics because they facilitate gas structure. Gas molecules (guests) are intensely encaged in dissolution and act as mini gas reservoirs (i.e., storing gas 28 the cavities of the host structure and exert a multi-directional molecules in the interior micellar core) in the solution. In force to prevent the host structure from collapsing.1,2 A large contrast, inorganic salts inhibit gas hydrate formation by capacity of storing gases in gas hydrates offers various novel reducing water reactivity and radically perturbing the applications, in such areas as separation and storage of tetrahedral network of water.29,30 With their strong electro- − − gases3 11 and sequestration of carbon dioxide.12 15 The fully static interactions with water molecules, inorganic ions reversible formation of hydrates also provides promising advantageously compete with the dissolved gas molecules for methods for eco-friendly refrigeration16,17 and desalination of water, therefore, reducing the amount of water available for 18,19 Downloaded via MPI POLYMERFORSCHUNG on September 15, 2020 at 07:22:30 (UTC). saline water. However, the formation of gas hydrates in forming gas hydrates. oil/gas pipelines is a well-known hazard to the safety and However, the formation of gas hydrates in dilute solutions of See https://pubs.acs.org/sharingguidelines for options on how to legitimately share published articles. efficiency of the oil and gas industry because it can lead to − surfactants and salts is relatively poorly understood. Under low blocking of the pipelines.20 23 In both situations, the formation concentration regimes, the effects of surfactants are expected to of gas hydrates must be controlled in desired manners to be different because the micelles are absent. It was found realize the opportunities or eliminate the hazards. recently that SDS at low (sub-millimolar) concentrations A feasible way to achieve these goals is to use additives as inhibits methane hydrate formation.31 Such peculiar inhibition 24−27 promoters or inhibitors for gas hydrate formation. A large was attributed to an aligned water layer underneath the number of additives have been reported as either promoters or charged gas−water interface as a result of the interfacial inhibitors for gas hydrate formation. Especially, surfactants and adsorption of ionic surfactants.31,32 This electrostatic align- salts have been intensively investigated because they are ment of interfacial water hinders the nucleation of gas hydrates directly relevant to gas hydrate formation in nature and the industry. The formation of gas hydrates in nature or pipelines is inevitably affected by natural surfactants and salts. Sodium Received: May 29, 2020 dodecyl sulfate (SDS) has been widely used as a model Revised: July 9, 2020 surfactant in hydrate research. SDS is well-known for its Published: July 20, 2020 effective promoting effect on gas hydrate formation. Adding SDS at concentrations above the critical micellization concentration (CMC) increased the rate of ethane hydrate © 2020 American Chemical Society https://dx.doi.org/10.1021/acs.energyfuels.0c01725 9971 Energy Fuels 2020, 34, 9971−9977 Energy & Fuels pubs.acs.org/EF Article at the gas−water interface because gas hydrate nucleation necessitates a tetrahedral ordering of water molecules.1 Likewise, sodium halides also behave anomalously under low-concentration regimes. While sodium halides at high concentrations are well-known thermodynamic inhibitors for gas hydrate formation, their solutions at low concentrations (between 50 and 70 mM) were found to act as kinetic − promoters.33 35 Nevertheless, to the best of our knowledge, the combined effects of surfactants and salts on gas hydrate formation under low-concentration regimes have never been investigated. Even though, these conditions are inherently relevant to the environments in pipelines or nature, where gas hydrate formation is affected by natural surfactants or inorganic ions (minerals). Oil and gas wells contain mixtures of hydro- carbons, water, and other condensate phases. These mixtures Figure 1. High-pressurecellformethanehydrateformation reside in underground formations and contact with various experiments. Accessory parts include (1) pressure transducer, (2) rocks and geological matters before they are extracted out. The thermocouple, (3) gas supply valve, (4) safety valve, (5) gas- water phases dissolve ions from the minerals and rocks. The discharging valve, and (6) stirrer. The temperature and pressure concentrations of ions in the water phases depend upon the inside the cell were monitored by a computer (not shown). chemistry of the rocks and minerals with which they have been in contact. Previous studies reported considerable concen- trations of iodide ions (I−) in water phases from conventional consumption at time t was calculated using the equation of state of − oil and gas wells.36 43 Harkness et al. also indicated a wide real gas range of iodide concentrations in the fluid from shale PV PV Δ=−= − (unconventional) gases.44 Natural surfactants can arise from nt() n0 nt ZRT tt=0 ZRT (1) similar origins. Here, we selected SDS and NaI as models of where n0 and nt are the amounts (moles) of methane at the beginning surfactants and monovalent ions, respectively. Another reason ji zy ji zy for the selection of NaI for our study is that this salt at low (t = 0) and time t, respectively,j zR is thej universalz gas constant, V is the gas volume in the reactor,k and {Z is thek methane{ compressibility factor concentrations exhibits a most pronounced effect on gas − 27 34 45−47 and calculated using the Brill Beggs equation. The hydrate growth hydrate formation and interfacial water structure rate, r(t), was calculated using eq 2 relative to other salts. Consequently, any potential effects of dΔn Δ+Δ−Δnt()() t nt the salt on gas hydrate formation and water structure could be r()t = ≈ monitored. We also complemented our kinetic experiments dt Δt (2) using sum frequency generation (SFG) vibrational spectros- where Δt is the time interval (0.5 min). In practice, we calculated the copy to gain molecular insights into the kinetic observations. gas uptake of the hydrate formation based on eq 1. Then, the growth rate of hydrate, r(t), was calculated using the gas uptake versus time (eq 2). We obtained time-dependent growth rate curves against the 2. EXPERIMENTAL SECTION reaction time. From these curves, we determined the maximum values 2.1. Materials. Chemicals used in this study included SDS (99%, of the growth rate and used those values to construct Figure 4. Under Sigma-Aldrich, St. Louis, MO, U.S.A.), NaI (99.5%, Novachem Pty given operating conditions, the experiments were repeated 5 times to Ltd), and methane (99.995%, Coregas, Australia). The water used was acquire averaged values for the induction time and growth rate. purified using an Ultrapure Academic Milli-Q system (Millipore, 2.3. SFG Experiment. SFG vibrational spectroscopy measure- Burlington, MA, U.S.A.). ments were carried out to examine the structural properties of water 2.2. Gas Hydrate Formation Experiment. The kinetics of at the gas−water interface (Figure 2). The details of this method were methane hydrate formation were investigated using
Details
-
File Typepdf
-
Upload Time-
-
Content LanguagesEnglish
-
Upload UserAnonymous/Not logged-in
-
File Pages7 Page
-
File Size-