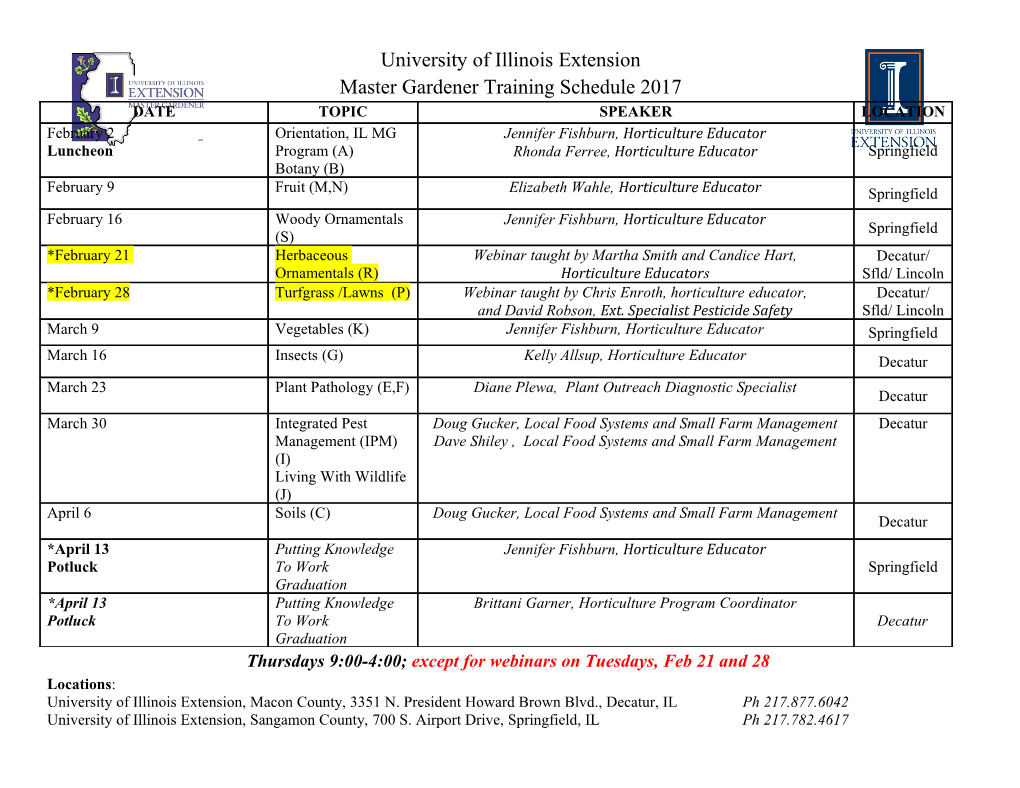
J Phys Fitness Sports Med, 1(4): 631-636 (2012) JPFSM: Review Article Arterial baroreflex regulation of cerebral blood flow in humans Shigehiko Ogoh1*, Ai Hirasawa1 and James P. Fisher2 1 Department of Biomedical Engineering, Toyo University, 2100 Kujirai, Kawagoe-shi, Saitama 350-8585, Japan 2 School of Sport and Exercise Sciences, University of Birmingham, Edgbaston, Birmingham, West Midlands B15 2TT UK Received: October 12, 2012 / Accepted: November 19, 2012 Abstract The arterial baroreflex plays an essential role in the short-term regulation of arterial blood pressure, and thus helps ensure that the vital organs are adequately perfused. For stand- ing humans, appropriate arterial baroreflex control of cardiac output and vasomotor tone are particularly important for cerebral blood flow regulation. However, the numerous mechanisms implicated in the regulation of the cerebral vasculature (e.g. cerebral autoregulation, carbon dioxide reactivity) mean that the precise nature of the direct and indirect effects of the arterial baroreflex on cerebral blood flow regulation are highly complex and remain incompletely un- derstood. This review paper provides an update on recent insights into the influence of the arte- rial baroreflex on cerebral circulation. Keywords : arterial blood pressure, cardiac output, cerebral autoregulation, cerebral CO2 reactiv- ity, autonomic nervous system control cerebral vascular resistance3). Introduction The concept that CA is a powerful mechanism of blood Adequate oxygen delivery is essential for the mainte- flow regulation in the brain has become well established. nance of cerebral function, and a loss of consciousness However, in the early studies of Lassen (1959), the CA rapidly results from inadequate cerebral perfusion and curve relating CBF to MAP was derived from eleven oxygen delivery. The importance of adequate perfusion steady-state data points, obtained under several differ- pressure for the delivery of oxygen to the tissues of the ent conditions and presented in previous publications. body is well established. Thus, the regulation of arterial For example, the lower limit of CA was determined from blood pressure (ABP) by arterial baroreflex modulation MAP and CBF data in young normotensive human sub- of sympathetic outflow to the heart and vasculature, and jects who had a decreased CBF during acute hypotension parasympathetic nerve activity to the heart1), would in- (35 mmHg)5) and in volunteers who had no change in tuitively seem to be an important mechanism for cerebral CBF during pharmacologically induced mild hypotension blood flow (CBF) regulation. However, regulation of CBF (57 mmHg)6). However, this approach is problematic as is highly complex, and neurogenic, hemodynamic, auto- steady-state CBF is regulated by other physiological fac- regulatory and metabolic factors can all contribute. This tors (e.g. cardiac output) as well as CA7-9). Recently, Lu- means that the precise direct and indirect contribution of the arterial baroreflex to the maintenance of cerebral perfusion remains challenging to distinguish. This review paper will provide an update on recent insights into the ow Lucascurve influence of cerebral autoregulation, arterial blood gases Fl and the arterial baroreflex, on cerebral circulation. ood Bl Cerebral autoregulation al Lassencurve 2) The classic work of Lassen established the concept that br human CBF is maintained within a narrow range despite re changes in mean arterial pressure (MAP) between 60 to Ce 150 mmHg (Fig. 1). This relationship is termed cerebral autoregulation (CA). CA is a homeostatic mechanism that 60 150 buffers fluctuations in CBF when cerebral perfusion pres- sure changes and acts through vasomotor effectors that Cerebral Perfusion Pressure Fig. 1 Human Cerebral Autoregulation Curve presented by *Correspondence: [email protected] Lassen2) and Lucas et al.4) 632 JPFSM: Ogoh S, et al. 4) + - cas et al. re-evaluated the CA curve in healthy humans, tively impermeable to [H ] and [HCO3 ] ions. Therefore, by monitoring cerebral perfusion while pharmacologically CO2 also acts as a powerful respiratory stimulant at the manipulating ABP over a wide range (Fig. 1). In contrast level of the central chemoreceptors in the brain14). The to Lassen2), it was observed that cerebral perfusion closely periodic nature of inspiration and expiration is finely con- followed the pharmacologically-induced change in blood trolled by changes in PaCO2, via the respiratory chemore- pressure. This indicates that CA is imperfect and that a fi- flex, such that pH remains relatively constant. Therefore, nite slope of the plateau region does not necessarily imply changes in CBF have an important role in stabilizing the a defective CA4). However, it should be acknowledged breathing pattern during fluctuating levels of chemical 4) that the vasoactive substances used by Lucas et al. may stimuli, especially PCO2 at the level of the central chemo- have had a direct effect on the cerebral vasculature10,11). receptors18). Indeed, Peebles et al.17) reported that hyper- capnic cerebral CO2 reactivity was inversely related to the increase in minute ventilation (VE). In goats, severe brain Partial pressure of arterial carbon dioxide (PaCO2) 19) ischemia blunts the ventilatory responses to CO2 . Our PaCO2 is a powerful mediator of CBF (Fig. 2). Hypo- research group also demonstrated that under conditions of capnia causes cerebral vasoconstriction and reduces CBF. hypercapnia and exercise, the total respiratory loop gain The resulting reduction in cerebral ‘washout’ attenuates (i.e., the sensitivity of the total respiratory system, includ- the fall of brain tissue PCO2. In contrast, hypercapnia ing the central chemoreflex and lung system, to changes increases CBF by vasodilating the cerebral vasculature, in CO2) was markedly reduced, while cerebrovascular 14) thus limiting elevations in brain tissue PCO2. As sleep de- CO2 reactivity increased . These findings suggest that creases cerebral CO2 reactivity, the level of cerebral neu- cerebrovascular CO2 reactivity is tightly linked to the ral activation may also influence cerebrovascular reactiv- ventilatory response to CO2, meaning that central che- 12) ity to CO2 . Dynamic CA, the rapid change in CBF that moreflex control of minute ventilation (VE) affects CBF buffers a transient change in ABP, is also influenced by regulation. Of note, abnormal chemoreflex control of 13) CO2 reactivity ; because when perfusion pressure is low, breathing is evident in a range of pathological conditions CO2 accumulates (hypercapinia), and at a high perfusion (e.g. chronic lung disease, heart failure, and sleep apnea) 20-22) pressure, CO2 washout (hypocapnia) occurs. The early and may alter dynamic CBF regulation . work of Aaslid et al.3) provided experimental evidence that hypocapnia improves dynamic CA while hypercapnia Partial pressure of arterial oxygen (PaO2) impairs it. An increase in PaCO2 exponentially elevates CBF, but PaO2 is another important regulator of CBF under spe- this hypercapnic cerebral CO2 reactivity is greater than cific conditions, such as high altitude, hypoxic exercise, hypocapnic reactivity14,15). Animal studies indicate that chronic lung disease, and sleep apnea. However, while this may be related to a greater influence of vasodila- hypoxia per se is a cerebral vasodilator and CBF rises tor mediators on intracranial vascular tone compared to in proportion to the severity of isocapnic hypoxia, un- vasoconstrictive mediators16). In humans, Peebles et al.17) der normal conditions the hypoxia-induced activation reported that during hypercapnia there is a large release of peripheral respiratory chemoreceptor activity leads of nitric oxide from the brain, whereas this response is to a hyperventilation-induced lowering of PaCO2 and absent during hypocapnia. subsequent cerebral vasoconstriction23,24). Therefore, the The blood-brain barrier is permeable to CO2, and rela- cerebrovascular bed receives conflicting signals during exposure to acute hypoxia at rest, with the net result often being a transient decrease in CBF coinciding with the peak hypoxic ventilatory response and the greatest degree of hypocapnia23). Conversely, CBF is well maintained with acute hypoxia during sub-maximal exercise, despite Normoxia a greater degree of hypocapnia23). This indicates that exer- cise modifies the interaction between PaO2 and PaCO2 in CBF the regulation of CBF. This is potentially due to hypoxia- induced changes in CA, sympathetic nerve activity and/ Hypoxia or changes in the sensitivity of the cerebrovascular bed to hypoxia and hypocapnia23). Recently, we examined the in- teraction between PO2 and PCO2 in CBF regulation (Ogoh et al. unpublished) and observed that during hypoxia (12%), CO2 reactivity was attenuated, indicating that hy- CO2 poxia may affect the CBF response to CO2 (Fig. 2). 14) Fig. 2 CBF responses to CO2 during normoxia and hypoxia condition (Ogoh unpublished data). JPFSM: ABR and CBF regulation 633 tion in humans did not elicit significant changes in SV, Sympathetic nerve activity: interactions with cerebral in either the seated or supine positions32,33). These results CO2 reactivity and autoregulation confirm the data of Geerdes et al.34) in animals. This sug- Traditional thought dictates that increases in sympa- gests that at rest, SV changes have minimal influence in thetic activity appear to have a limited effect on the cere- regulating ABP via the carotid baroreflex. Therefore, ca- bral vasculature of humans, particularly at rest.
Details
-
File Typepdf
-
Upload Time-
-
Content LanguagesEnglish
-
Upload UserAnonymous/Not logged-in
-
File Pages6 Page
-
File Size-