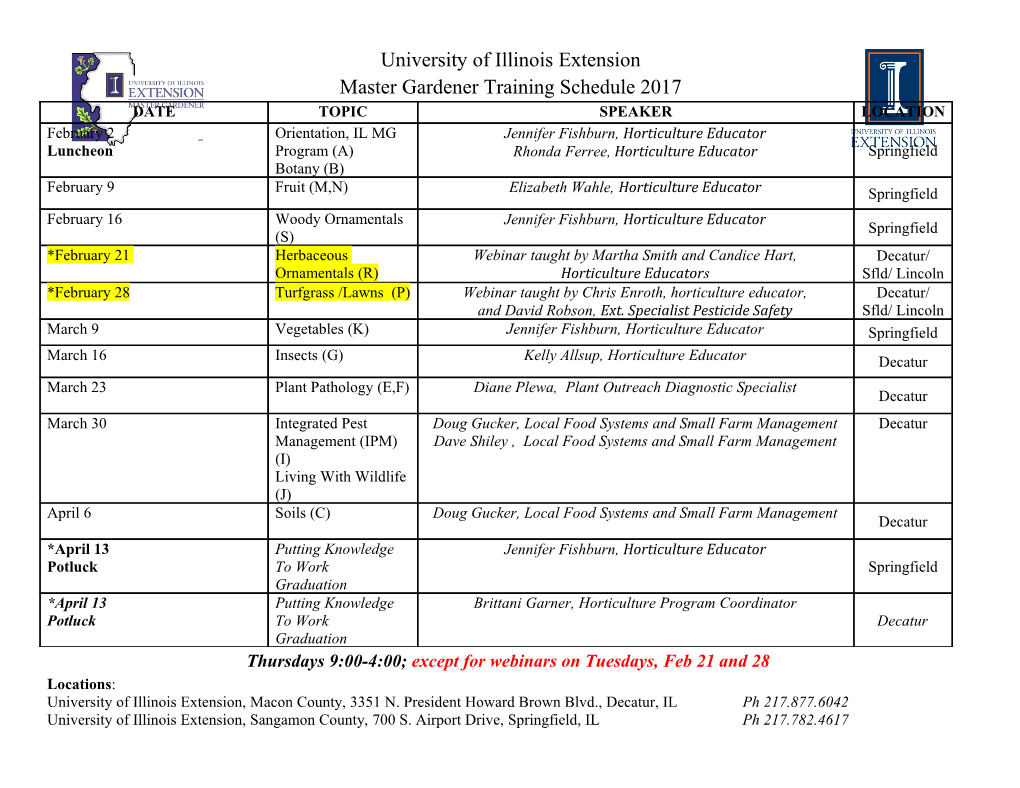
F E RIDE IA R M IC E O D A A C L A E X A M N V D L R L I I G I A S E N 3–21 Stellar Evolution: Massive Stars Structure on the Main Sequence: Simulations show existence of two regimes: lower main sequence : stars have structure similar to Sun: − energy generation: pp-chain (ǫ ∝ T 5) − inner radiative core − convective hull 6 upper main sequence : for central temperatures of 18 × 10 K (1.5 M⊙ stars): pp-chain and CNO-cycle produce equal amounts of energy. Above that: CNO dominates. − energy generation: CNO-cycle (ǫ ∝ T 17) − inner convective core since energy generation from CNO cycle strongly peaked towards center. − outer radiative hull Evolution of the structure of a 3 M⊙ star to the early Asymptotic Giant Branch (Maeder & Meynet, 1989). Evolution of the Sun 26 F E RIDE IA R M IC E O D A A C L A E X A M N V D L R L I I G I A S E N 3–22 Stellar Evolution: Massive Stars Evolution on MS similar, however, faster than for low mass stars. More massive stars (& 1.5 M⊙) reach threshold temperature for 3α before reaching degeneracy =⇒ He just starts to burn. In these objects, higher order fusion processes can kick in (but are energetically unimportant): alpha reactions 12C + 4He → 16O + γ 16O + 4He → 20Ne + γ Internal structure of a 3 M⊙ star 20Ne + 4He → 24Mg + γ which has reached the early Asymptotic Giant Branch. Outer layers continue H shell burning. During evolution of star on red giant branch: convective hull moves deeper into core, can mix fusion products into outer layers. Maeder & Meynet, 1989 Evolution of the Sun 27 Kippenhahn et al. (1965): Evolution of the internal structure of a 5 M⊙ star. Evolution of the structure of a 7 M⊙ star to the carbon burning phase (Maeder & Meynet, 1989). Internal structure of a 7 M⊙ star Kippenhahn et al. (1965) which just starts its carbon burning Evolution of a 5 M⊙ star in the HRD. phase. Maeder & Meynet, 1989 F F E RIDE E RIDE IA R IA R M IC M IC E O E O D D A A A A C C L L A A E E X X A A M M N N V V D D L L R R L L I I I I G G I I A A S S E E N 3–29 N 3–30 Stellar Evolution: Massive Stars Stellar Evolution: Massive Stars −10.0 He C+O Supernova II 5 C+C 10 H He AGB −7.5 Evolution of stars in the HRD 25 Msun To White Dwarf (0.85 Msun) from main sequence to death 4 PN absolute Magnitude 10 To White Dwarf (0.6 Msun) Ejection −5.0 Typical timescales (units of 106 yr; He C+O Predicted evolution of HRD from Schaller et al. 1992): 3 PN lights up 10 −2.5 globular clusters can reproduce 5 MsunH He 1 M⊙ 5 M⊙ 25 M⊙ Core AGB their HRDs, allows age 2 Helium H→He 10000 94 6.4 10 Flash Horizontal Branch 0.0 He→C 12 0.6 determination Luminosity (Lsun) C+C 0.01 1 Low metallicity He C+O RGB 10 stars only +2.5 PN . 0.01 . 0.01 N/A Result: ∼12...13 billion years Cooling C−O WD ∞ ∞ N/A 0 White Dwarf (0.6 Msun) H He =⇒ GCs are oldest objects in 10 +5.0 1 Msun the universe! −1 10 +7.5 Post-H-burning burning: need higher 4.6 4.4 4.2 4.0 3.8 3.6 3.4 core temperatures (Coulomb barrier!), log T less energy release =⇒ last much shorter than hydrogen burning. (M68; Straniero et al., 1997; Fig. 11) after Iben, 1991 Evolution of the Sun 34 Evolution of the Sun 36 F E RIDE IA R M IC E O D A A C L A E X A M N V D L R L I I G I A S E N 3–30 Stellar Evolution: Massive Stars −5 Predicted evolution of HRD from globular clusters can reproduce bol 0 their HRDs, allows age M determination Age = 0.00 Gyr 0.02 Gyr 0.10 Gyr 0.32 Gyr 5 1.00 Gyr 10.00 Gyr 19.95 Gyr 30000 20000 10000 5000 Temperature [K] after Bertelli et al. (1994) Evolution of the Sun 35.
Details
-
File Typepdf
-
Upload Time-
-
Content LanguagesEnglish
-
Upload UserAnonymous/Not logged-in
-
File Pages3 Page
-
File Size-