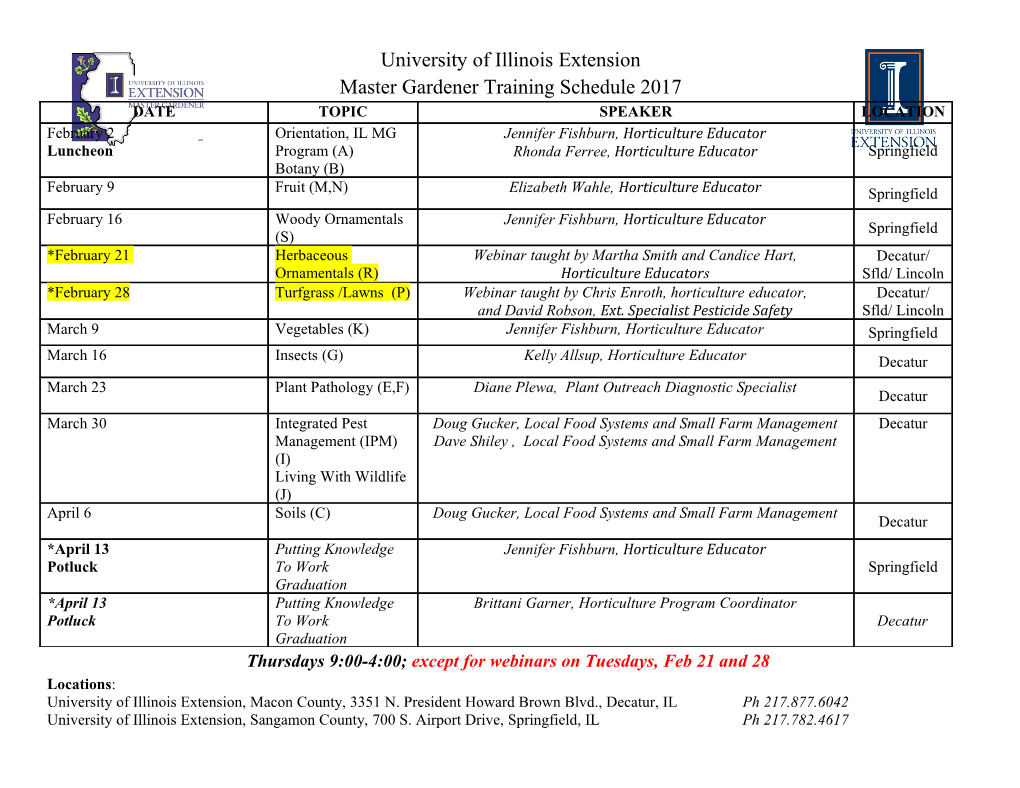
110 Wing and Jørgensen, JAAVSO Volume 31, 2003 Stellar Spectra in the H Band Robert F. Wing Department of Astronomy, Ohio State University, Columbus, OH 43210 Uffe G. Jørgensen Niels Bohr Institute, and Astronomical Observatory, University of Copenhagen, DK-2100 Copenhagen, Denmark Presented at the 91st Annual Meeting of the AAVSO, October 26, 2002 [Ed. note: Since this paper was given, the AAVSO has placed 5 near-IR SSP-4 photometers with observers around the world; J and H observations of program stars are being obtained and added to the AAVSO International Database.] Abstract The H band is a region of the infrared centered at wavelength 1.65 microns in a clear window between atmospheric absorption bands. Cool stars such as Mira variables are brightest in this band, and the amplitudes of the light curves of Miras are typically 5 times smaller in H than in V. Since the AAVSO is currently exploring the possibility of distributing H-band photometers to interested members, it is of interest to examine the stellar spectra that these photometers would measure. In most red giant stars, the strongest spectral features in the H band are a set of absorption bands due to the CO molecule. Theoretical spectra calculated from model atmospheres are used to illustrate the pronounced flux peak in H which persists over a wide range of temperature. The models also show that the light in the H band emerges from deeper layers of the star’s atmosphere than the light in any other band. 1. Introduction When multicolor photometry in the infrared was first standardized in the 1960s, Harold Johnson and his colleagues acquired filters to match the windows in the atmospheric absorption and named them with letters of the alphabet (Johnson 1966). It was only by fortunate coincidence that the widths of the windows between the main bands of atmospheric water vapor are about right for the filters of a wideband multicolor system. The photometric bands (windows) were named alphabetically in order of wavelength following the letter I (which was already understood to mean the nearest infrared, just beyond the red). The infrared filters, in combination with the established optical ones, then formed the UBVRIJKLMN…system (Table 1). In this early work, for several years, there was no H. Historical research (Wing 1994) has shown that it was Eugenio Mendoza of Mexico who introduced the H band, using a filter provided by Johnson in 1966 (Mendoza 1967). This was an important addition for several reasons. Of all the infrared windows, the H band, located halfway between the J and K bands, is the cleanest. It plugs a significant gap in the energy distributions of stars obtained by multicolor photometry, and it is right at the highest point of the energy distributions Wing and Jørgensen, JAAVSO Volume 31, 2003 111 Table 1. Central wavelengths of filters of the standard wideband photometric system. Filter Wavelength (µm) Filter Wavelength (µm) U 0.36 H 1.65 B 0.44 K 2.2 V 0.55 L 3.4 R 0.70 M 5.0 I 0.90 N 10: J 1.25 of many cool stars. According to Dr. Mendoza (1993), it was only by historical accident that this important band was skipped in the early work and later named out of alphabetical order: Johnson had simply been unable to acquire a suitable filter for the H band, and he went ahead without it. As readers may be aware, the Council of the AAVSO have been discussing the possibility of adding some kind of infrared photometry to the organization’s observing repertoire, and a Working Group on Infrared Photometry has been established. Since multicolor infrared work does not seem feasible for private, low- budget observatories, considerable thought has been given to the question of which single infrared filter would provide the most useful supplement to the visual light curves which will undoubtedly remain the major part of the organization’s database. The H band has been selected, and it is the purpose of this paper to discuss the reasons for this choice. In the infrared, the longer the wavelength the brighter the background. Indeed, at the longer infrared wavelengths (beyond about 3 µm), one has to observe through the thermal emission from not only the atmosphere but also the telescope mirror and anything else in the optical path. With increasing wavelength, the technology needed for cooling, chopping, etc. becomes rapidly more elaborate and expensive. The H band, at 1.65 µm, represents the longest wavelength (and hence the most different from V) which we think can be measured in a reasonable number of stars with a small telescope and a simple (thermoelectric) system to cool the detector. In addition, the excellent transparency of the earth’s atmosphere in the H band should allow good photometry to be obtained from sea-level observatories. Most of this paper will deal with spectroscopic information, of two kinds: (a) the position of the H band within the spectral energy distributions of stars of different types, and (b) the spectral content of the H band, i.e., the molecular bands and atomic lines that fall within this window and absorb some of the radiation. Theoretical spectra will be used for this purpose. In Section 5 we will briefly consider the character of the light curves that cool variables are expected to show in H, and what we can learn from them. 112 Wing and Jørgensen, JAAVSO Volume 31, 2003 2. Synthetic spectra A spectrum calculated from a mathematical model of a stellar atmosphere is commonly called a “synthetic spectrum,” and the magnitudes and colors obtained by multiplying a synthetic spectrum by a set of filter response functions are called “synthetic photometry” and “synthetic colors.” The calculations are horrendous, but once the programs and line lists are set up, even a theoretician can enjoy doing photometry! An important application of synthetic photometry is to derive fundamental parameters of stars such as effective temperature and gravity. This is done by comparing synthetic colors to observed colors. When a good match is obtained for a sufficiently extensive set of colors, the effective temperature of the model used to generate the synthetic colors is taken to be the effective temperature of the matching star. This type of comparison, which usually involves a collaboration between (at least) two people, makes both the observations and the calculations much more useful than they would be by themselves. To turn the observed colors and indices into fundamental stellar parameters, the model atmospheres are indispensable. At the same time, the models would be of limited value if they were not tested through comparison of observed and synthetic spectra and colors. The authors of this paper were involved in just such comparisons when we realized that the figures we were generating might be of interest to the AAVSO, especially to members involved in the development and use of an H-band photometer. A model atmosphere specifies the temperature, gas pressure, etc. of each atmospheric layer, and this in turn determines the degree of ionization and excitation of each type of atom, as well as the equilibrium between atoms and molecules, in each layer. The photons making up a star’s spectrum arise from a range of depths in the atmosphere, and their probability of escaping from the star depends upon the absorption characteristics of these atoms and molecules. A tremendous amount of atomic and molecular data must therefore be stored in the computer before a realistic spectrum, with all its absorption lines, can be computed. The difficulties are especially severe for the coolest stars, since the molecules that form at low temperatures have very complex absorption spectra. The development of programs to compute model atmospheres, and the compilation of the necessary line lists for all the relevant atoms and molecules, has been the work of a great many people over several decades (see, for example, Gustafsson and Jørgensen 1985, 1994). Until quite recently, however, applications of synthetic spectra were mostly limited to stars warmer than about 4000 K. For cooler stars, comparisons with observation were discouraging—the models were known to be deficient, the line lists were incomplete, and some important molecules could not yet be included in the calculations at all. In the last few years, however, the situation has changed dramatically, primarily as a result of the inclusion of water vapor, which grossly affects not only the spectra of cool stars but also the structure of their atmospheres (Jørgensen et al. 2001). Current models for cool stars are computed with opacities based on 50 million or more lines from the water molecule alone! Wing and Jørgensen, JAAVSO Volume 31, 2003 113 Once synthetic spectra can be calculated correctly, they constitute an excellent educational tool. One can, like a spectroscopist, explore a limited region of the spectrum in detail, or one can compute the entire spectrum, from zero wavelength to infinity, and examine the overall energy distribution. We will do a little of both. 3. Energy distributions of dwarfs and giants The synthetic spectra of a set of solar-composition dwarfs (i.e., main-sequence stars) of various temperatures are shown in Figure 1. They were computed at the Niels Bohr Institute in Copenhagen, using model atmospheres calculated with the MARCS code, which was originally developed at the Uppsala Observatory in Sweden. The quantity plotted is the flux per unit frequency interval. It may seem odd to plot the flux in frequency units against the wavelength, but this choice gives the best (flattest) presentation of the spectra in the region of interest. To interpret the overall energy distributions, it is helpful to compare them to blackbody curves plotted in the same units (Figure 2).
Details
-
File Typepdf
-
Upload Time-
-
Content LanguagesEnglish
-
Upload UserAnonymous/Not logged-in
-
File Pages11 Page
-
File Size-