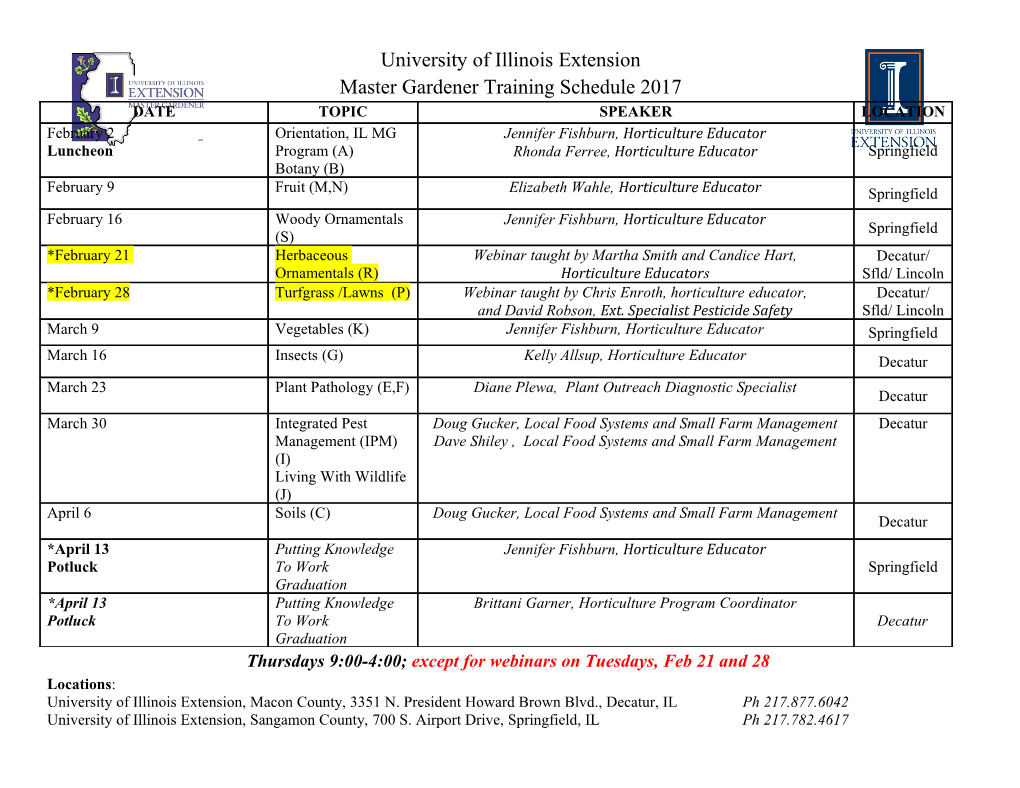
Manin matrices, Casimir elements and Sugawara operators Alexander Molev University of Sydney 1 I Origins and motivations. I Basic properties of Manin matrices. I Applications: I Casimir elements for gln. I Segal–Sugawara vectors for gln. Plan 2 I Basic properties of Manin matrices. I Applications: I Casimir elements for gln. I Segal–Sugawara vectors for gln. Plan I Origins and motivations. 2 I Applications: I Casimir elements for gln. I Segal–Sugawara vectors for gln. Plan I Origins and motivations. I Basic properties of Manin matrices. 2 I Segal–Sugawara vectors for gln. Plan I Origins and motivations. I Basic properties of Manin matrices. I Applications: I Casimir elements for gln. 2 Plan I Origins and motivations. I Basic properties of Manin matrices. I Applications: I Casimir elements for gln. I Segal–Sugawara vectors for gln. 2 By the seminal works of V. Drinfeld (1985) and M. Jimbo (1985), the universal enveloping algebra U(g) of a simple Lie algebra g admits a deformation Uq(g) in the class of Hopf algebras. The dual Hopf algebras are quantized algebras of functions Funq(G) on the associated Lie group G [N. Reshetikhin, L. Takhtajan and L. Faddeev 1990]. A detailed review of the theory and applications: V. Chari and A. Pressley, A guide to quantum groups, 1994. Quantum groups 3 The dual Hopf algebras are quantized algebras of functions Funq(G) on the associated Lie group G [N. Reshetikhin, L. Takhtajan and L. Faddeev 1990]. A detailed review of the theory and applications: V. Chari and A. Pressley, A guide to quantum groups, 1994. Quantum groups By the seminal works of V. Drinfeld (1985) and M. Jimbo (1985), the universal enveloping algebra U(g) of a simple Lie algebra g admits a deformation Uq(g) in the class of Hopf algebras. 3 A detailed review of the theory and applications: V. Chari and A. Pressley, A guide to quantum groups, 1994. Quantum groups By the seminal works of V. Drinfeld (1985) and M. Jimbo (1985), the universal enveloping algebra U(g) of a simple Lie algebra g admits a deformation Uq(g) in the class of Hopf algebras. The dual Hopf algebras are quantized algebras of functions Funq(G) on the associated Lie group G [N. Reshetikhin, L. Takhtajan and L. Faddeev 1990]. 3 Quantum groups By the seminal works of V. Drinfeld (1985) and M. Jimbo (1985), the universal enveloping algebra U(g) of a simple Lie algebra g admits a deformation Uq(g) in the class of Hopf algebras. The dual Hopf algebras are quantized algebras of functions Funq(G) on the associated Lie group G [N. Reshetikhin, L. Takhtajan and L. Faddeev 1990]. A detailed review of the theory and applications: V. Chari and A. Pressley, A guide to quantum groups, 1994. 3 The algebra Funq(Mat2) is generated by four elements a; b; c; d, " ab # understood as the entries of the matrix , such that cd ba = qab; dc = qcd; ca = qac; db = qbd; and bc = cb; ad − da + (q − q−1)bc = 0: [L. Faddeev and L. Takhtajan 1986]. Basic example 4 ba = qab; dc = qcd; ca = qac; db = qbd; and bc = cb; ad − da + (q − q−1)bc = 0: [L. Faddeev and L. Takhtajan 1986]. Basic example The algebra Funq(Mat2) is generated by four elements a; b; c; d, " ab # understood as the entries of the matrix , such that cd 4 and bc = cb; ad − da + (q − q−1)bc = 0: [L. Faddeev and L. Takhtajan 1986]. Basic example The algebra Funq(Mat2) is generated by four elements a; b; c; d, " ab # understood as the entries of the matrix , such that cd ba = qab; dc = qcd; ca = qac; db = qbd; 4 [L. Faddeev and L. Takhtajan 1986]. Basic example The algebra Funq(Mat2) is generated by four elements a; b; c; d, " ab # understood as the entries of the matrix , such that cd ba = qab; dc = qcd; ca = qac; db = qbd; and bc = cb; ad − da + (q − q−1)bc = 0: 4 Basic example The algebra Funq(Mat2) is generated by four elements a; b; c; d, " ab # understood as the entries of the matrix , such that cd ba = qab; dc = qcd; ca = qac; db = qbd; and bc = cb; ad − da + (q − q−1)bc = 0: [L. Faddeev and L. Takhtajan 1986]. 4 A 2 × 2 matrix is q-Manin if the elements x0 and y0 defined by " x0 # " ab #" x # = ; y0 cd y 0 0 0 0 satisfy y x = qx y . The defining relations for Funq(Mat2) " ab # are equivalent to the conditions that the matrix cd and its transpose are q-Manin matrices. As observed by Yu. Manin (1988), the relations are recovered via a “coaction” on the quantum plane: the algebra with generators x; y and the relation yx = qxy. 5 The defining relations for Funq(Mat2) " ab # are equivalent to the conditions that the matrix cd and its transpose are q-Manin matrices. As observed by Yu. Manin (1988), the relations are recovered via a “coaction” on the quantum plane: the algebra with generators x; y and the relation yx = qxy. A 2 × 2 matrix is q-Manin if the elements x0 and y0 defined by " x0 # " ab #" x # = ; y0 cd y satisfy y0x0 = qx0y0. 5 As observed by Yu. Manin (1988), the relations are recovered via a “coaction” on the quantum plane: the algebra with generators x; y and the relation yx = qxy. A 2 × 2 matrix is q-Manin if the elements x0 and y0 defined by " x0 # " ab #" x # = ; y0 cd y 0 0 0 0 satisfy y x = qx y . The defining relations for Funq(Mat2) " ab # are equivalent to the conditions that the matrix cd and its transpose are q-Manin matrices. 5 Consider the characteristic polynomial of a matrix 2 3 M11 ::: M1n 6 7 6 . 7 M = 6 . 7 6 7 4 5 Mn1 ::: Mnn with entries in C: n det(I + tM) = 1 + ∆1 t + ··· + ∆n t : In particular, ∆1 = tr M; ∆n = det M: MacMahon Master Theorem 6 n det(I + tM) = 1 + ∆1 t + ··· + ∆n t : In particular, ∆1 = tr M; ∆n = det M: MacMahon Master Theorem Consider the characteristic polynomial of a matrix 2 3 M11 ::: M1n 6 7 6 . 7 M = 6 . 7 6 7 4 5 Mn1 ::: Mnn with entries in C: 6 In particular, ∆1 = tr M; ∆n = det M: MacMahon Master Theorem Consider the characteristic polynomial of a matrix 2 3 M11 ::: M1n 6 7 6 . 7 M = 6 . 7 6 7 4 5 Mn1 ::: Mnn with entries in C: n det(I + tM) = 1 + ∆1 t + ··· + ∆n t : 6 MacMahon Master Theorem Consider the characteristic polynomial of a matrix 2 3 M11 ::: M1n 6 7 6 . 7 M = 6 . 7 6 7 4 5 Mn1 ::: Mnn with entries in C: n det(I + tM) = 1 + ∆1 t + ··· + ∆n t : In particular, ∆1 = tr M; ∆n = det M: 6 Consider the algebra n n End C ⊗ ::: ⊗ End C ⊗ C | {z } k and for a = 1;:::; k set n X Ma = I ⊗ ::: ⊗ I ⊗ eij ⊗ I ⊗ ::: ⊗ I ⊗ Mij: | {z } | {z } i;j=1 a−1 k−a Regard the matrix M as the element n X n M = eij ⊗ Mij 2 End C ⊗ C: i;j=1 7 and for a = 1;:::; k set n X Ma = I ⊗ ::: ⊗ I ⊗ eij ⊗ I ⊗ ::: ⊗ I ⊗ Mij: | {z } | {z } i;j=1 a−1 k−a Regard the matrix M as the element n X n M = eij ⊗ Mij 2 End C ⊗ C: i;j=1 Consider the algebra n n End C ⊗ ::: ⊗ End C ⊗ C | {z } k 7 Regard the matrix M as the element n X n M = eij ⊗ Mij 2 End C ⊗ C: i;j=1 Consider the algebra n n End C ⊗ ::: ⊗ End C ⊗ C | {z } k and for a = 1;:::; k set n X Ma = I ⊗ ::: ⊗ I ⊗ eij ⊗ I ⊗ ::: ⊗ I ⊗ Mij: | {z } | {z } i;j=1 a−1 k−a 7 Denote by H(k) and A(k) the respective images of the symmetrizer and anti-symmetrizer 1 X 1 X σ and sgn σ · σ: k! k! σ2Sk σ2Sk We regard H(k) and A(k) as elements of the algebra n n End C ⊗ ::: ⊗ End C : | {z } k The symmetric group Sk acts on the tensor product space n n C ⊗ ::: ⊗ C | {z } k by permutations of tensor factors. 8 We regard H(k) and A(k) as elements of the algebra n n End C ⊗ ::: ⊗ End C : | {z } k The symmetric group Sk acts on the tensor product space n n C ⊗ ::: ⊗ C | {z } k by permutations of tensor factors. Denote by H(k) and A(k) the respective images of the symmetrizer and anti-symmetrizer 1 X 1 X σ and sgn σ · σ: k! k! σ2Sk σ2Sk 8 The symmetric group Sk acts on the tensor product space n n C ⊗ ::: ⊗ C | {z } k by permutations of tensor factors. Denote by H(k) and A(k) the respective images of the symmetrizer and anti-symmetrizer 1 X 1 X σ and sgn σ · σ: k! k! σ2Sk σ2Sk We regard H(k) and A(k) as elements of the algebra n n End C ⊗ ::: ⊗ End C : | {z } k 8 Introduce the sums n X k (k) Ferm = 1 + (−1) tr A M1 ::: Mk = det(I − M); k=1 1 X (k) Bos = 1 + tr H M1 ::: Mk: k=1 Theorem [P. MacMahon 1916]. We have the identity Bos × Ferm = 1: We have (k) ∆k = tr A M1 ::: Mk; k = 1;:::; n: 9 Theorem [P. MacMahon 1916]. We have the identity Bos × Ferm = 1: We have (k) ∆k = tr A M1 ::: Mk; k = 1;:::; n: Introduce the sums n X k (k) Ferm = 1 + (−1) tr A M1 ::: Mk = det(I − M); k=1 1 X (k) Bos = 1 + tr H M1 ::: Mk: k=1 9 We have (k) ∆k = tr A M1 ::: Mk; k = 1;:::; n: Introduce the sums n X k (k) Ferm = 1 + (−1) tr A M1 ::: Mk = det(I − M); k=1 1 X (k) Bos = 1 + tr H M1 ::: Mk: k=1 Theorem [P.
Details
-
File Typepdf
-
Upload Time-
-
Content LanguagesEnglish
-
Upload UserAnonymous/Not logged-in
-
File Pages119 Page
-
File Size-