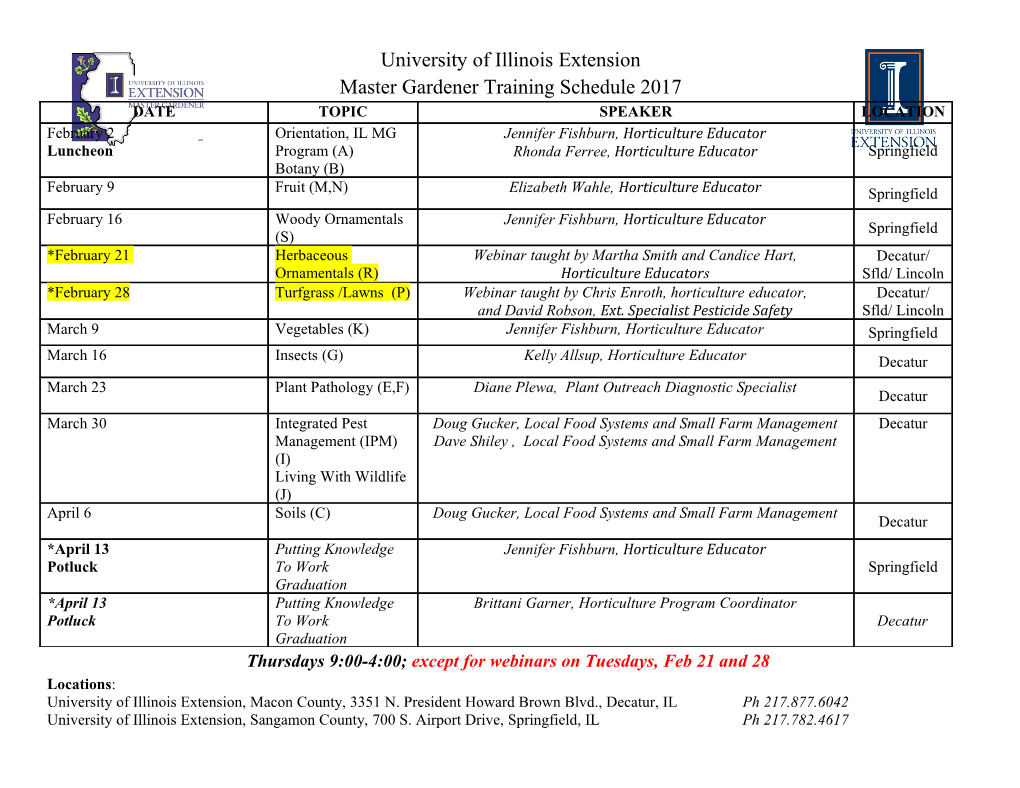
would be released as the martian atmo- struments flown on sounding rockets and sphere struck the surface, a phenomenon terrestrial satellites, by about a factor of seen earlier during perigee passes of the x 0.01 103. The low-mass spectra are currently Earth orbital satellites Explorer C, Ex- being analyzed: preliminary results sug- plorer D, and Explorer E. The mass peak 15N 14N 15N 14N 14N 14N gest upper limits for the mixing ratios of at 16 includes contributions from CO2, TERRESTRIAL H2 and He relative to CO2 of about 10-4. CO, 02, and H2O, in addition to a residu- A. 0. NIER al most probably due to 0. A quan- School of Physics and Astronomy, titative analysis is difficult, however, 12 170 University ofMinnesota, Minneapolis since 0 may be expected to react rapidly M. B. McELROY with surfaces in the instrument. It is Center for Earth and Planetary hoped that further laboratory studies will 13c 1O J2c 160, Physics, Harvard University, clarify these matters, and that they might Cambridge, Massachusetts 02138 eventually permit a more quantitative es- timate for the concentration of 0. 14N 160 References and Notes 1. A. 0. Nier, W. B. Hanson, A. Seiff, M. B. The densities of NO, as shown in Fig. NOISE 12 _8 McElroy, N. W. Spencer, R. J. Duckett, T. C. 2, were obtained from a detailed analysis D. Knight, W. S. Cook, Science 193, 786 (1976). 30 29 28 A typographical error appears in line 11, column of the peak at mass number 30. Figure 3 MASS (AMU) 1, p. 787. The sentence should read "Peaks at gives a scale representation of a typical masses 18 and 17 are...." Fig. 3. Block diagram representing to scale 2. A. 0. Nier, M. B. McElroy, Y. L. Yung, ibid. spectrum obtained by VL1 at an altitude the relative heights for the mass peaks at 30, 194, 68 (1976). near 130 km. Slightly more than half of 29, and 28 amu in a spectral scan obtained 3. K. Biemann, T. Owen, D. R. Rushneck, A. L. near an altitude of 130 km during the descent LaFleur, D. W. Howarth, ibid., p. 76. the signal at mass 30 may be attributed to 4. M. B. McElroy, Y. L. Yung, A. 0. Nier, ibid., of VL1. The diagram shows the contribution p. 70. 12C18O+ formed by the dissociative ioni- of CO+ (from the fragment due to the dis- 5. M. B. McElroy, ibid. 175, 443 (1972). zation of 12C'80160. After correction for sociation of CO2 in the ion source and from 6. F. D. Colegrove, W. B. Hanson, F. S. Johnson, J. Geophys. Res. 70, 4931 (1965); R. S. Lindzen, 12C18O+ from both CO2 and CO, there re- ambient CO) to the several peaks. The remain- Mesospheric Models and Related Experiments, mains a component whose magnitude sig- der, in the case of the peaks at mass 29 and 28, G. Giocco, Ed. (Reidel, Dordrecht, Netherlands, is attributed to ambient N2. The excess peak 1971), p. 120. nificantly exceeds the noise level of the at mass 30 is attributed to ambient NO. The 7. A. 0. Nier and J. L. Hayden, Int. J. Mass is most rea- Spectrom. Ion Phys. 6, 339 (1971). amplifier. This component diagram also illustrates that both the excess 8. A. 0. Nier, J. H. Hoffman, C. Y. Johnson, J. C. sonably attributed to NO, and it is seen peak at mass 30 and the excess peak at mass Holmes, J. Geophys. Res. 69, 979 (1964); A. 0. in all spectra for which the peak at mass 29 (beyond that expected if the nitrogen has a Nier, W. E. Potter, D. R. Hickman, K. Mauers- terrestrial isotopic composition) are well berger, Radio Sci. 8, 271 (1973); J. B. French, 30 exceeds the amplifier noise back- above the noise level of the amplifier which N. M. Reid, A. 0. Nier, J. L. Hayden, CASI Trans. 5, 77 (1972); J. L. Hayden, A. 0. Nier, J. on June 10, 2014 ground. The data indicate a mixing ratio measured the ion currents. B. French, N. M. Reid, R. J. Duckett, Int. J. for NO relative to CO2 of approximately Mass Spectrom. Ion Phys. 15, 37 (1974). 9. M. B. McElroy, T. Y. Kong, Y. L. Yung, A. 0. 10-4. The density of NO in the upper at- Nier, Science 194, 1295 (1976). Mars is thus use electrometer amplifiers rather than 10. J. Mattauch and R. Herzog, Z. Phys. 89, 786 mosphere of significantly (1934). higher than the density of NO at com- electron multipliers. The background 11. Work at the University of Minnesota and at were in Harvard University was supported under NASA parable levels of Earth's atmosphere. fluctuations of these amplifiers contracts NAS- 1-9697 and NAS-1-10492, respec- It is clear from even a casual in- the range (1 to 5) x 10-'4 amp. The in- tively. A.O.N. is indebted to M. Wade and W. spection ofthe data in Fig. 2 that the mar- strumental sensitivity on Viking was Johnson for help in the computations. tian atmosphere must be mixed to therefore less than that for similar in- 12 November 1976 heights greater than 130 km. This obser- www.sciencemag.org vation implies an eddy diffusion coefficient of at least 5 x 107 cm2 sec-1. One would expect a density for N2 of about 5 x 107 cm-3 at 190 km in VLl, or Structure of Mars' Atmosphere up to 100 Kilometers about 108 cm-3 at 160 km in VL2, if diffu- from the Entry Measurements of Viking 2 sive separation should occur above 130 km. The measured densities at these alti- Abstract. The Viking 2 entry science data on the structure ofMars' atmosphere up Downloaded from tudes are only 2 x 107 cm-3 and 4 x 107 to 100 kilometers define a morning atmosphere with an isothermal region near the cm-3, respectively. The densities as surface; a surface pressure 10 percent greater than that recorded simultaneously at measured for CO are also consistent with the Viking I site, which implies a landing site elevation lower by 2.7 kilometers than the assumption of rapid vertical mixing, the reference ellipsoid; and a thermal structure to 100 kilometers at least qualita- as discussed elsewhere (9). tively consistent with pre-Viking modeling of thermal tides. The temperature profile The upper atmospheric mass spec- exhibits waves whose amplitude grows with altitude, to 25°K at 90 kilometers. trometers on both VL1 and VL2 used These waves are believed to be a consequence of layered vertical oscillations and Mattauch-Herzog geometry (10), which associated heating and cooling by compression and expansion, excited by the daily allowed for simultaneous collection of thermal cycling ofthe planet surface. As is necessary for gravity wave propagation, ions with different masses. Two collect- the atmosphere is stable against convection, except possibly in some very local re- ors were used to measure ions differing gions. Temperature is everywhere appreciably above the carbon dioxide con- in mass by approximately a factor of 7 densation boundary at both landing sites, precluding the occurrence ofcarbon diox- (7). The heavy collector was sensitive to ide hazes in northern summer at latitudes to at least 50°N. Thus, ground level mists the mass range 7 to 49 atomic mass units seen in these latitudes would appear to be condensed water vapor. (amu), whereas the light collector record- ed ions in the range 1 to 7 amu. Because The second Viking lander entered the Time (P.D.T.), and landed at 3:58:20 of weight restrictions imposed at an early atmosphere of Mars on 3 September p.m. P.D.T. in Utopia Planitia at 47.66°N stage of the project, we were obliged to 1976 at about 3:49 p.m. Pacific Daylight latitude and 225.78°W longitude at 9:06 1300 SCIENCE, VOL. 194 a.m. Mars local time. During entry and Table 1. Surface elevations at the Viking 2 landing site indicated by measurements of the plan- descent, measurements were made to de- et's radius after landing. fine the structure of the atmosphere be- Radius (km) Landing low about 120 km, as on Viking 1 (1). site Smooth elevation Real-time transmission of the entry data eismooth Measured b - a to the earth, by relay through the orbiter, (a)elsi(b) (km) was prevented by problems with the atti- tude stabilization of the orbiter during Topographic 3385.0 + 0.7(14) 3381.4 + 1.3(15) -3.6 ± 1.5 this period. The data were recorded on Gravitational 3384.3 ± 0.7(14) 3381.5 ± 0.6(16) -2.8 ± 0.9 tape recorders, however, on both the or- biter and the lander, and were trans- to mitted the earth from the orbiter rec- lander radar altimeter below about 130 difference with the measured pressure ord almost exactly 24 hours later. The re- km were also invaluable. difference. sults presented here are based on The first data interpreted after touch- The profile of density for the altitude analysis of the first orbiter replay. down were the temperature and pressure range to 100 km defined by Viking 2 (pri- We present a preliminary analysis, measurements during parachute descent, marily by the accelerometers) is shown which does not include all the correc- shown in Fig. 1. Significant atmospheric in Fig. 2. (The upper altitude limit will ul- tions and refinements that will ultimately temperature data were obtained by the timately extend to about 125 km.) Den- be applied. The primary corrections sensor mounted on footpad 2, from the sities determined independently from omitted here are corrections for effects time ofleg deployment at 3.9 km to retro- stagnation pressure measurements are in- due to atmospheric final winds, correc- rocket firing at 1.5 km.
Details
-
File Typepdf
-
Upload Time-
-
Content LanguagesEnglish
-
Upload UserAnonymous/Not logged-in
-
File Pages4 Page
-
File Size-