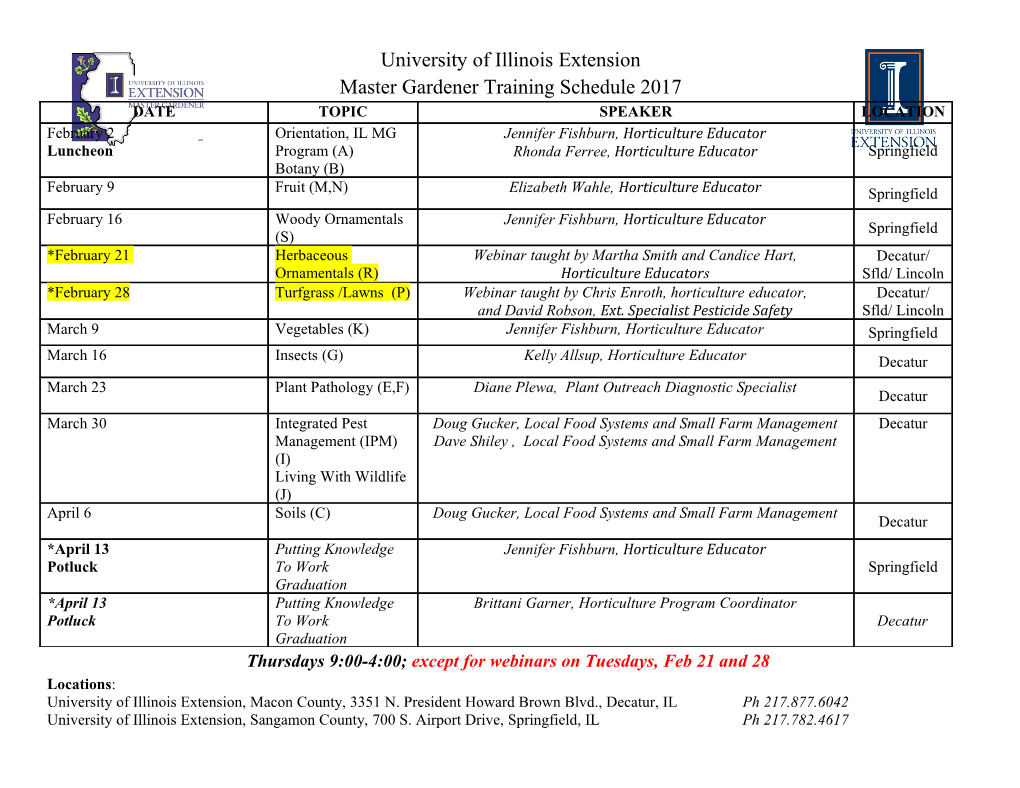
PHYS 4011, 5050: Atomic and Molecular Physics Lecture Notes Tom Kirchner1 Department of Physics and Astronomy York University April 7, 2013 [email protected] Contents 1 Introduction: the field-free Schr¨odinger hydrogen atom 2 1.1 Reductiontoaneffectiveone-bodyproblem . 2 1.2 The central-field problem for the relative motion . 4 1.3 Solution of the Coulomb problem . 5 1.4 Assortedremarks ......................... 13 2 Atoms in electric fields: the Stark effect 16 2.1 Stationary perturbation theory for nondegenerate systems .. 17 2.2 Degenerateperturbationtheory . 22 2.3 Electric field effects on excited states: the linear Stark effect . 24 3 Interaction of atoms with radiation 29 3.1 The semiclassical Hamiltonian . 29 3.2 Time-dependentperturbationtheory . 32 3.3 Photoionization .......................... 41 3.4 Outlook on field quantization . 44 4 Brief introduction to relativistic QM 58 4.1 Klein-Gordonequation . 58 4.2 Diracequation .......................... 60 5 Molecules 70 5.1 The adiabatic (Born-Oppenheimer) approximation . 71 5.2 Nuclear wave equation: rotations and vibrations . 74 + 5.3 The hydrogen molecular ion H2 ................. 77 1 Chapter 1 Introduction: the field-free Schr¨odinger hydrogen atom The starting point of the discussion is the stationary Schr¨odinger equation Hˆ Ψ= EΨ (1.1) for the two-body problem consisting of a nucleus (n) and an electron (e). The Hamiltonian reads pˆ2 pˆ2 Ze2 Hˆ = n + e (1.2) 2m 2m − 4πǫ r r n e 0| e − n| with 31 m = N 1836m ; m 9.1 10− kg n e e ≈ × and N being the number of nucleons (N = 1 for the hydrogen atom itself, where the nucleus is a single proton). The first step is to separate this two-body problem into two effective one-body problems. 1.1 Reduction to an effective one-body prob- lem Consider the (classical) coordinate transformation • (r , p , r , p ) (R, P, r, p) e e n n −→ 2 3 definitions : M = me + mn mn memn ≈ µ = me me + mn ≈ R = mnrn+mere r M ≈ n center of mass − − motion P = p + p = MR˙ p e n ≈ n r = r r e − n relative mnpe mepn motion p = µ˙r = − p M ≈ e QM transformation analogously • (ˆr , pˆ , ˆr , pˆ ) (Rˆ , Pˆ , ˆr, pˆ) (1.3) e e n n −→ insertion into Eq. (1.2) yields Pˆ 2 pˆ2 Ze2 Hˆ = + + V (r) , V (r)= − (1.4) 2M 2µ 4πǫ0r = HˆCM + Hˆrel Eq. (1.4) is the Hamiltonian of a non-interacting two-(quasi-)particle :system ֒ can be separated into two one-particle problems → ansatz: Ψ(r, R) = ΦCM (R)ϕrel(r) (1.5) (Schr¨odinger equations (SEs ֒ → ~2 Hˆ Φ (R) = 2 Φ (R)= E Φ (R) (1.6) CM CM −2M ∇R CM CM CM ~2 Ze2 Hˆ ϕ (r) = 2 φ (r)= E ϕ (r) (1.7) rel rel −2µ∇r − 4πǫ r rel rel rel 0 with E = ECM + Erel (1.8) Equation (1.6) can be solved without difficulty: Φ (R)= AeiKR ֒ → CM 1 free particle K = ~ P − motion ~2K2 ECM = 2M 4 Equation (1.7) can be solved analytically, but before we sketch the solution we consider some general properties/features of the quantum central-field (V (r)= V (r)) problem. 1.2 The central-field problem for the relative motion pˆ2 Consider Hˆ = + V (r) . rel 2µ (1.9) One can show that Hˆrel is invariant with respect to rotations, and therefore commutes with the angular momentum operator ˆl = ˆr pˆ. (1.10) × This is a manifestation of angular momentum conservation. In particular, 2 the operators Hˆrel,ˆl , ˆlz form a complete set of compatible operators, i.e., ˆ2 ˆ ˆ2 ˆ [Hˆrel, l ] = [Hˆrel, lz] = [l , lz] = 0 (1.11) they have a common set of eigenstates. The eigenstates of ˆl2, ˆl are the ֒ → z spherical harmonics Ylm. (ansatz ϕ (r)= R (r)Y (θ,ϕ) . (1.12 ֒ → rel l lm insertion into Eq. (1.7) for Hamiltonian (1.9) yields the radial SE 2 ~2 pˆr l(l + 1) + 2 + V (r) Erel Rl(r) = 0 (1.13) (2µ 2µr − ) ~2 withp ˆ2 = ∂ (r2∂ ) r − r2 r r ˆl2 and operator identity pˆ2 =p ˆ2 + r r2 (can be proven in spherical coordinates in coordinate space). Useful definition : yl(r)= rRl(r) (1.14) 5 (1.13) l(l + 1) (y′′(r)+ ǫ U(r) y (r) = 0 (1.15 ֒ → l − − r2 l h i ~2 ~2 E = ǫ, V (r)= U(r) rel 2µ 2µ 1.3 Solution of the Coulomb problem The radial Eq. (1.15) is very similar to the one-dimensional SE. There are, however, two important differences. First, the total (effective) potential con- sists of two parts eff l(l + 1) r U (r) = U(r)+ →∞ 0 l r2 −→ ′′angular momentum barrier′′ տ (cf. classical central-field problem) Second, the boundary conditions are dif- ferent. a) Boundary conditions r 0 • ϕ−→(r) 2 = R (r) 2 Y (θ,ϕ) 2 < | rel | | l | | lm | ∞ in particular for r =0 ’regularity condition’ ֒ → yl(0)=0 (1.16) r • −→ ∞ 1. Erel < 0 (bound spectrum) ∞ ϕ (r) 2 d3r = r2R2(r) dr Y (θ,ϕ) 2 dΩ | rel | l | lm | Z Z0 Z ∞ = y2(r) dr < l ∞ Z0 (square integrable solutions required) r (y (r) →∞ 0 (′strong′ boundary condition ֒ → l −→ 6 2. Erel > 0 (continuous spectrum) oscillatory solutions y (r) for r ֒ → l −→ ∞ note: for Erel > 0 the solution leads to Rutherford’s scattering formula (which is identical in classical mechanics and QM) b) Bound-state solutions definition : κ2 = ǫ > 0 − ~2 4πǫ0 10 a = 0.53 10− m µe2 ≈ · for µ m , a a is the ′Bohr radius′ ≡ e ≡ 0 :(radial Eq. (1.15 ֒ → 2 d l(l + 1) 2Z 2 2 2 + κ yl(r) = 0 (1.17) dr − r ar − ! transformation: x =2κr d2 1 d2 = ֒ → dx2 4κ2 dr2 d2 l(l + 1) λ 1 (yl(x) = 0 (1.18 + 2 2 ֒ → dx − x x − 4! asymptotic solutions: 1. x −→ ∞ d2 1 yl(x)=0 2 ֒ → dx − 4! x x y (x)= Ae− 2 + Be 2 ֒ → l because of y (x )=0 ֒ B =0 l →∞ → 7 2. x 0 −→ d2 l(l + 1) yl(x)=0 2 2 ֒ → dx − x ! A y (x)= + Bxl+1 ֒ → l xl . because of y (0) = 0 ֒ A = 0 l → This consideration motivates the following ansatz l+1 x yl(x)= x e− 2 vl(x). (1.19) Insertion into Eq. (1.18) yields a new differential equation for vl(x): d2 d (x 2 + (2l +2 x) (l +1 λ) vl(x)=0. (1.20 ֒ → ( dx − dx − − ) The square integrable solution of (1.20) (’Kummer’s’ or ’Laplace’s’ differen- tial eq.) are known; they are the associated Laguerre polynomials: 2 p (p + k)! Lk(x)= ( )j xj. p − (p j)!(k + j)!j! j=0 X − More specifically: 2l+1 nr = n l 1 0 vl(x)= L (x) , − − ≥ n l 1 n 1 l − − ⇐⇒ − ≥ Z with n λn = , n =1, 2, ... (1.21) ≡ κna The detailed treatment shows that the integrability of the solutions requires λ = Z to be positive, integer numbers quantization of κ (i.e. quantiza- κa −→ tion of the energy)1 l+1 κnr 2l+1 (ynl(r)= Anlr e− Ln l 1(2κnr ֒ → − − 1 One finds the square integrable solutions of (1.20) explicitly by using the ansatz vl(x)= l i i bix and by taking the boundary (and regularity) conditions into account. P 8 and properly normalized wave functions take the form (n l 1)! 1 l+ 2 l+2 ϕrel(r) ϕnlm(r) = − − 3 2 κn √a ≡ (n + l)! l κnr 2l+1 r e− Ln l 1(2κnr)Ylm(θ,ϕ) (1.22) × − − n 0 ≥ Rnl(r)Ylm(θ,ϕ) l n 1 ≡ l≤ m− l − ≤ ≤ The quantization condition (1.21) yields ~2 Z2 E E = n =1, 2, ... (1.23) rel ≡ n −2µa2 n2 Z2 13.6 eV . (1.24) ≈ − n2 The lowest-lying hydrogen eigenfunctions (’orbitals’): nl m nr = n l 1 ϕnlm(r) En − − 3 − 2 Zr 1 Z a 2 100 0 1s √π a e− RZ 3 1 Z 2 Zr Zr RZ2 200 1 2s 2 e 2a 4√2π a a − 4 3 − 1 Z 2 Zr Zr RZ2 210 0 2p e 2a cos θ 0 4√2π a a − 4 3 1 Z 2 Zr Zr iϕ RZ2 2 1 1 0 2p 1 e− 2a sin θe± ± ± ∓ 8√π a a 4 with R = 13.6 eV 9 E s p d 0 n = 3 3 s 3 p - 1 3 p 0 3 p 1 3 d - 2 3 d - 1 3 d 0 3 d 1 3 d 2 n = 2 2 s 2 p - 1 2 p 0 2 p 1 n = 1 1 s Figure 1.1: Energy spectrum of the Schr¨odinger-Coulomb problem. Note that n the Coulomb potential supports infinitely many bound states (E →∞ 0). n −→ Degeneracy of energies (which depend only on n) given n l =0, 1, ..., n 1 given l m = l, ..., l − − n 1 − 2l +1)= n2) ֒ → Xl=0 each energy level E is n2-fold degenerate. Note that all central-field −→ n problems share the (2l + 1)-fold degeneracy which originates from rotational invariance. The fact that the energies do not depend on nr,l separately, but only on n = nr + l + 1 is specific to the Coulomb problem (one names n the principal quantum number and nr the radial quantum number, which determines the number of nodes in the radial wave functions).
Details
-
File Typepdf
-
Upload Time-
-
Content LanguagesEnglish
-
Upload UserAnonymous/Not logged-in
-
File Pages84 Page
-
File Size-