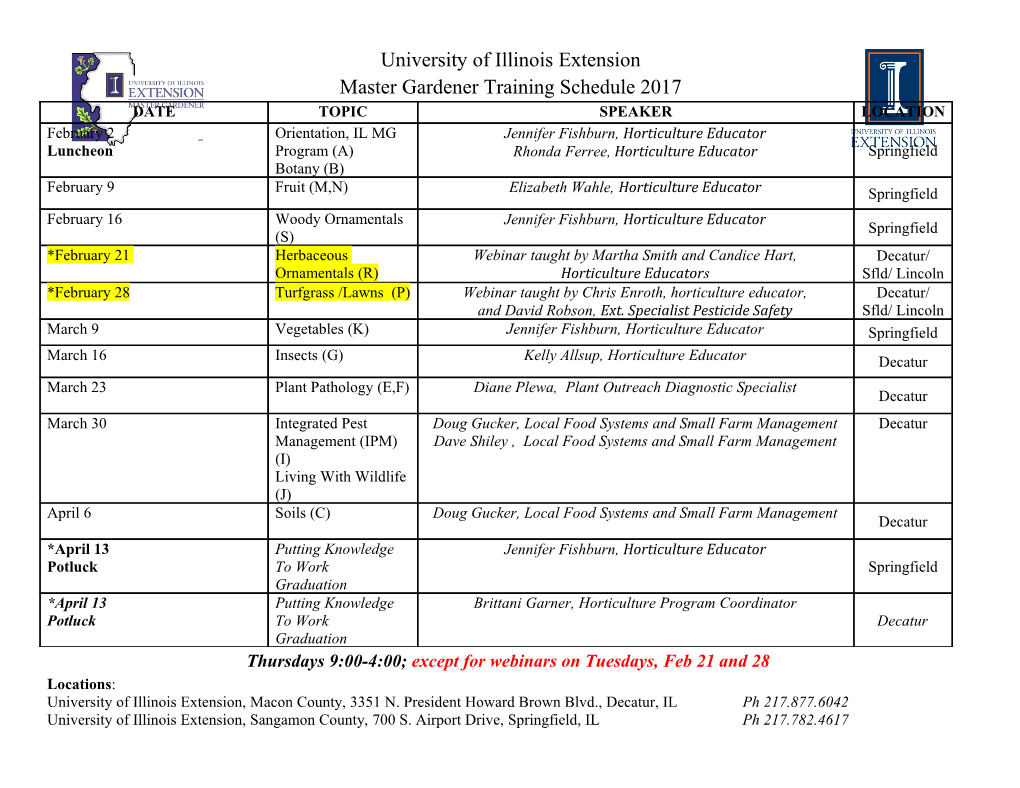
A seven-helix coiled coil Jie Liu*, Qi Zheng*, Yiqun Deng*, Chao-Sheng Cheng*, Neville R. Kallenbach†, and Min Lu*‡ *Department of Biochemistry, Weill Medical College of Cornell University, New York, NY 10021; and †Department of Chemistry, New York University, New York, NY 10003 Edited by Janet M. Thornton, European Bioinformatics Institute, Cambridge, United Kingdom, and approved August 28, 2006 (received for review June 12, 2006) Coiled-coil proteins contain a characteristic seven-residue se- and d residues. Interior packing of the side chains at the a and quence repeat whose positions are designated a to g. The inter- d positions, in fact, has been shown to dominate the global acting surface between ␣-helices in a classical coiled coil is formed architecture of coiled coils (23). Polar side chains at the a and by interspersing nonpolar side chains at the a and d positions with d positions also can destabilize coiled-coil structure yet impose hydrophilic residues at the flanking e and g positions. To explore a high degree of conformational selectivity (4, 24). Moreover, how the chemical nature of these core amino acids dictates the ionic interactions between the e and g residues have been shown overall coiled-coil architecture, we replaced all eight e and g to influence the specificity of coiled-coil assembly (10–18). For residues in the GCN4 leucine zipper with nonpolar alanine side example, repulsive or attractive interactions between the e and chains. Surprisingly, the alanine-containing mutant forms a stable g side chains can control the extent of homo- versus heterodimer- ␣-helical heptamer in aqueous solution. The 1.25-Å resolution ization in model coiled coils (10). This current level of under- crystal structure of the heptamer reveals a parallel seven-stranded standing of the determinants of coiled-coil structure has allowed coiled coil enclosing a large tubular channel with an unusual formulation of robust rules for coiled-coil design, embodied in heptad register shift between adjacent staggered helices. The algorithms that are available for this purpose. Thus, it seems overall geometry comprises two interleaved hydrophobic helical remarkable that fundamental elements of coiled-coil formation screws of interacting cross-sectional a and d layers that have not still remain incompletely understood. Here, we report a striking been seen before. Moreover, asparagines at the a positions play an example resulting from an unanticipated role of nonpolar side essential role in heptamer formation by participating in a set of chains at the normally charged e and g positions. buried interhelix hydrogen bonds. These results demonstrate that Recent experiments show that the presence of apolar amino BIOPHYSICS heptad repeats containing four hydrophobic positions can direct acids at either the e or g position of the heptad repeat can specify assembly of complex, higher-order coiled-coil structures with rich formation of antiparallel four-helix structures (see refs. 17 and diversity for close packing of ␣-helices. 18). In these tetramers, the buried a, d, e and a, d, g side chains interlock in nonclassical combinations of knobs-against-knobs protein design ͉ protein structure ͉ helix–helix interfaces ͉ buried polar and knobs-into-holes packing to form the hydrophobic core. The interactions ͉ cavity lac repressor type of core packing, for example, is of the a, d, e class, with the helices offset by 0.25 heptad (25, 26); on the other elix–helix interactions are ubiquitous in the native structure hand, in the severe acute respiratory syndrome coronavirus S2 Hof proteins and in associations among proteins, including protein, the packing involves the a, d, g side chains with the helix supramolecular assemblies and transmembrane receptors that register shifted by 0.5 heptad (27). Evidently, patterning of mediate cellular signaling and transport. Coiled coils afford a hydrophobic and polar residues beyond the canonical 3–4 coiled- unique model system for elucidating principles of molecular coil sequence can lead to distinctive three-dimensional struc- recognition between helices (1–4). The conformation of coiled tures with characteristic supercoil shapes and exceptional sta- coils is prescribed by a characteristic 7-aa repeat, the 3–4 heptad bility. We have previously investigated the basis of a, d, g core a b c d e f g a d repeat denoted as - - - - - - (5). Positions and are typically packing by characterizing variants of the GCN4 leucine zipper occupied by hydrophobic amino acids such as Leu, Ile, Val, and that adopt antiparallel tetrameric structures in response to Ala, whereas residues at positions e and g are frequently polar hydrophobic substitutions at the g positions (17). Here, we report or charged (5–8). Crick’s (9) classical analysis proposed that the the engineering of a GCN4 leucine-zipper variant containing nonpolar a and d side chains associate by means of complemen- exclusively alanine residues at the e and g positions (Fig. 1). tary ‘‘knobs-into-holes’’ packing to form supercoiled ␣-helical Surprisingly this mutant peptide folds into a hitherto unreported ribbons. Beyond the role of hydrophobic side chains at the core a and d positions, coiled coils can use intra- and interhelical seven-stranded coiled coil consisting of two interleaved parallel electrostatic interactions to tune their stability, especially those helical screws in which the helices are staggered in phase with the between the flanking e and g positions of neighboring chains heptad repeat. This heptamer highlights the need to extend (10–18). Despite their seeming regularity in sequence and current rules for coiled-coil folding and assembly to include packing patterns, coiled coils exhibit remarkable diversity in the nonpolar side chains at the e and g positions. In addition, it number and arrangement of the associating helices: two- to provides a soluble model for analyzing intrahelical interactions five-stranded structures have been identified with parallel or antiparallel helix orientation (2). The structural parameters of Author contributions: J.L. and M.L. designed research; J.L., Q.Z., Y.D., and C.-S.C. performed dimers have been described by Crick (9), and general rules research; J.L., Q.Z., Y.D., C.-S.C., N.R.K., and M.L. analyzed data; and N.R.K. and M.L. wrote governing the close packing of ␣-helices in hexamers and the paper. dodecamers have been deduced (19, 20). The authors declare no conflict of interest. Analysis of coiled coils provides insight into the general This article is a PNAS direct submission. problem of protein folding by simplifying the geometrical and Abbreviation: ABS, acetate-buffered saline. topological context. Although the hydrophobic cores of globular Data deposition: The atomic coordinates and structure factors have been deposited in the proteins can be repacked quite freely, formation of an apolar Protein Data Bank, www.pdb.org (PDB ID code 2HY6). core in coiled coils is spatially constrained, allowing dissection of ‡To whom correspondence should be addressed at: Department of Biochemistry, Weill their structure and folding in unprecedented detail (21, 22). The Medical College of Cornell University, 1300 York Avenue, New York, NY 10021. E-mail: canonical knobs-into-holes packing of coiled coils confers ex- [email protected]. quisite sensitivity to the stereochemical properties of the core a © 2006 by The National Academy of Sciences of the USA www.pnas.org͞cgi͞doi͞10.1073͞pnas.0604871103 PNAS ͉ October 17, 2006 ͉ vol. 103 ͉ no. 42 ͉ 15457–15462 Downloaded by guest on September 25, 2021 Fig. 1. Helical wheel projection of residues Met-1 to Arg-34 of the GCN4-pAA sequence. The view is from the N terminus. Heptad repeat positions are labeled a through g. GCN4-pAA differs from the recombinant dimeric leucine- zipper peptide GCN4-pR by alanine substitutions at four e and four g positions (bold). The sequences of GCN4-pR and GCN4-pAA (with the eight mutated e and g positions set in italics) are as follows: GCN4-pR, MK VKQLEDK VEELLSK NYHLENE VARLKKL VGER; GCN4-pAA, MK VKQLADA VEELASA NYHLANA VARLAKA VGER. The GCN4-pR sequence contains an additional Met-Lys-Val and no Arg-Met at its N terminus but is otherwise identical to GCN4-p1. in more complex protein interfaces and makes available a unique nanoscale model cavity to explore by using different solutes. Results and Discussion Design of GCN4-pAA. We chose the dimeric GCN4 leucine zipper as our engineering scaffold because it represents the prototypical and best-studied model with which to decipher the structural determinants of coiled-coil assembly and geometry (23). The hydrophobic interface between the two ␣-helical chains of the leucine zipper is formed by interlocking of residues at positions a, d, e, and g (28). Interhelical ionic interactions between charged side chains at the e and g positions also can contribute to dimerization specificity (28). Our previous studies have demonstrated that replacing g residues with nonpolar amino acids in the recombinant leucine-zipper peptide GCN4-pR can switch both stoichiometry and helix orientation to produce a stable antiparallel tetramer structure (17). This finding suggests that the selection of hydrophobic residues at both the e and g Fig. 2. The GCN4-pAA peptide forms a seven-stranded helical bundle. (A)CD positions of the 3–4 heptad repeat might offer another mecha- spectrum at 20°C in ABS (pH 5.2) and 50 M peptide concentration. (B) nism of mediating association of ␣-helices. To investigate this Thermal melt monitored by CD at 222 nm. (C) Representative sedimentation equilibrium data of a 100 M sample at 20°C and 18,000 rpm in ABS (pH 5.2). possibility, we engineered a GCN4-pR variant called GCN4- The data fit closely to a heptameric complex. (Upper) The deviation in the data pAA with alanine substitutions at four e and four g positions from the linear fit for a heptameric model is plotted.
Details
-
File Typepdf
-
Upload Time-
-
Content LanguagesEnglish
-
Upload UserAnonymous/Not logged-in
-
File Pages6 Page
-
File Size-