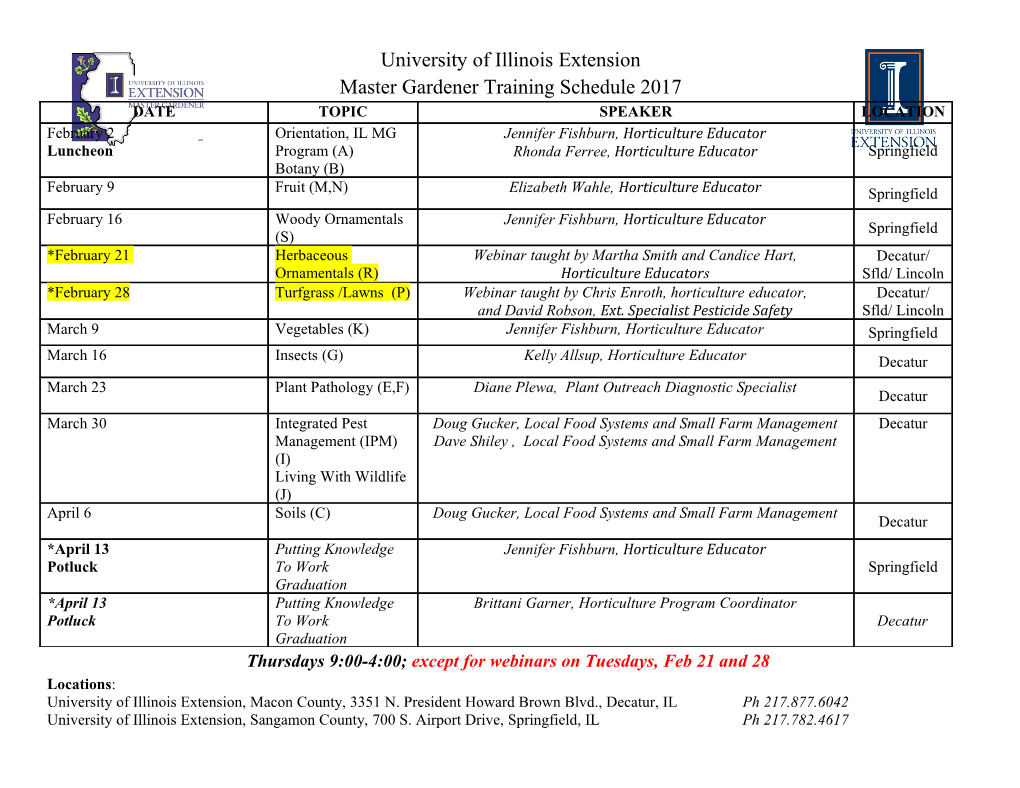
Materials Transactions, Vol. 45, No. 8 (2004) pp. 2489 to 2495 #2004 The Japan Institute of Metals OVERVIEW Metallurgical Chemistry in My Life* Noboru Masuko Metallurgical chemistry’s principle is that ‘‘the chemical process is governed by chemical potential.’’ Successful chemical process technology follows a route that does not go against the governance of chemical potential. In order to realize the technological objective, raw materials and a reactor are necessary, and after the principle is established, the method is supported by the reactor and advances in the materials that comprise such apparatus. Therefore, the technology is a fusion of material, apparatus, experience, and science, all of which are parts of the foundation of a technological method. The author’s involvement is described as an academician from the postwar recovery to the technological rearmament period. In the postwar recovery period, a systematic point-of-view was introduced to a technological principle using phase diagrams that clarified chemical potential, which was a new concept in the field of thermodynamics. In the technological rearmament period, technological evaluation was conducted from a philosophical standpoint. (Received April 26, 2004; Accepted June 2, 2004) Keywords: chemical potential, reactor, Eh-pH diagram, Eh-pH-pL diagram, potential diagram 1. Introduction 2. Chemical Potential Diagrams Ever since graduation from the university in 1957, I have 2.1 Eh-pH-pL diagram resided at the university and worked on joint research with Gibbs defined the intensive quantity of chemical potential metallurgical chemistry industries. Metallurgical chemistry in 1875. As appreciation increased that the concept of phase is a technology, and it has a principle and a method. The rule provided an important foundation of the phase diagram, principle is that ‘‘the chemical process is governed by numerous binary system diagrams for metals were developed chemical potential.’’ That is, successful chemical process from 1900 to 1920. While phase diagrams were measured in technology follows a route that does not go against the many systems and data were accumulated, the measurements governance of chemical potential. In order to realize the of chemical potential were also made, so that actual technological objective, raw materials and a reactor are measurements were available in a database by around necessary, and after the principle is established, the method is 1940. For example, Latimer’s Oxidation Potentials1) was supported by the reactor and advances in the materials that published in 1938, and the second edition in 1952. comprise such apparatus. Therefore, the technology is a With the availability of these actual measurements, fusion of material, apparatus, experience, and science, all of diagrams which presented chemical potential explicitly which are parts of the foundation of a technological method. began to acquire practical meaning. The primer was The process of metallurgical chemistry in Japan has gone Pourbaix’s ‘‘Eh-pH diagram.’’ However, Pourbaix’s 1937 through various developmental stages in its 60-year history thesis was not accepted by corrosion researchers at the time, from recovery after World War II to the present. I call these and, as he himself noted, ‘‘Corrosion is kinetics, so it has stages: the postwar recovery period (19451965), the high nothing to do with thermodynamic equilibrium.’’ With the development period (1975), the technological rearmament support of U.R. Evans, the English translation of the thesis2) period (1985), the exotic materials period (1995), and the was published in 1949. It took time before the clarity of global environment period (1995present). The efforts of ‘‘building a kinetic theory based on the difference of university researchers took several forms in response to the chemical potential as the driving force’’ could be appreciated, needs of each period. however, and it was not until 1960 that this method became This paper describes my involvement as an academician the new stream of thermodynamic application to the hetero- from the postwar recovery to the technological rearmament geneous system. Through my exposure to this ‘‘Eh-pH period. In the postwar recovery period, a systematic point-of- diagram,’’ I was able to work in the vanguard of this stream in view was introduced to a technological principle using phase the field of metallurgical chemistry including the field of diagrams that clarified chemical potential, which was a new hydrometallurgy. I am grateful to have come along at the concept in the field of thermodynamics. In the technological right moment in history. rearmament period, technological evaluation was conducted In 1958, I began research in uranium metallurgy at the from a philosophical standpoint. Institute of Mineral Dressing and Metallurgy of Tohoku University. The carbonate leaching method was applied to uranium ore which was contained in an alkaline based rock. In order to understand the principle of this method, I devised the Eh-pH-pL diagram, where the pL axis, which represents an inverse logarithm of ligand activity that composes the * This Paper was Presented at the Spring Meeting of the Japan Institute of 3) Metals, held in Tokyo, on March 30, 2004, and originally published in complex, is added to Pourbaix’s Eh-pH diagram. Figure 1 Japanese in Materia Japan 43 (2004). shows the conditions of formation of a uranyl carbonate 4À The Forty-Ninth Gold Medalist of the Japan Institute of Metals, 2004 complex [UO2(CO3)3 ] in the stable region of CaCO3 in the 2490 N. Masuko 8 0 7 ++ 6 Fe 2 2- Mn++ 4 5 Zn++ Zn (OH)2 Fe(OH)2 -0.5 2+ ++ FeCO 4 Cu 3 Zn ZnCO3 Zn(CN) Mn(OH)2 Zn(CN) 3 Cu(OH) 2 2- 3 CuCO3 MnCO3 Ca++ 2 pCO Ca(OH)2 ++ 2 Ni Ni(OH)2 2 CaCO3 ZnO 1 -1.0 Zn(OH) Eh / V Eh / 0 NiCO3 Mg++ Zn -1 Mg(OH)2 MgCO3 -2 -1.5 -3 5 6789101112 13 14 15 16 17 pH Fig. 1 Stability domain for uranyl-carbonate complex ion. -2.0 0 5 10 15 pH Fig. 3 Eh-pH diagram of zinc cyanide complex ion. 1 4- [UO2(CO3)3] Eh / V Eh / -0.5 0.5 ++ Cu(NH3) 4 UO3H2O UO3H2O 2+ Zn 2 Zn(OH)2 Eh / V Eh / + Cu(NH3) 2 0 -1.0 Zn(CN) 2 4 1.3 2 468101214 -0.5 8 pH UO2 Zn(CN) 6 -1 4 5 6 7 8 9 10 11 12 13 14 15 CN 4 p pH 2 - + MnO4 + 4H + 3e = MnO2 + 2H2O + 0 O2 + 4H + 4e = 2H2O ++ + Cu(NH3)4 + e = Cu(NH3) 2 + 2NH3 Fig. 2 Eh-pH diagram showing oxidation of UO2 in alkaline solution. Fig. 4 Eh-pH-pCN three-dimensional diagram. rock. Figure 2 shows the Eh-pH diagram representing the categorized into three basic reactions according to the form principle of the use of the cupric amine complex to uranyl of sulfur. Taking the example of sphalerite (ZnS), the carbonate for air oxidation of UO2 in ore. following are the categories: The principle of hydrometallurgy using complex produc- ZnS þ 2Hþ ¼ Zn2þ þ H S tion can be explained clearly by expanding the Eh-pH 2 diagram to a system containing this complex. To collect gold ðhydrogen sulfide generating typeÞ and silver by zinc cementation from pregnant cyanide ZnS ¼ Zn2þ þ S þ 2e solution, it is useful to prepare potential-pH diagrams for gold, silver, and zinc in cyanide solution. Figure 3 shows that ðelemental sulfur forming typeÞ 2þ À þ principle, and Fig. 4 shows the ternary phase diagram using ZnS þ 4H2O ¼ Zn þ HSO4 þ 7H þ 8e 4) zinc as an example. ðsulfuric acid forming typeÞ 2.2 Direct acid leach for sphalerite The potential-pH diagram in Fig. 5 clearly shows the In the beginning of the 1960s, those in the field of conditions under which these reactions occur.5) Experimental nonferrous metallurgy sought possible ways to introduce investigations were conducted for each reaction category, hydrometallurgy to the process of Kuroko (a complex sulfide and processing conditions were identified. Also, an experi- mineral), which is a resource unique to Japan. Hydro- ment using platinum electrode potential as a monitor is metallurgical processing of the sulfide mineral can be shown in Fig. 6. This method of process control employing Metallurgical Chemistry in My Life 2491 E / V 0.4 1.0 Zn++ Pu4+ - Zn++ 10 0.3 HSO4 A PO2 = 1 ++ 2- 0.5 Zn SO4 S PuO2 11 12 = ϕ 0.2 -50 2.5 5 pO - PCl2 = 1 3+ Pu -2 PCl2 = 10 1 Eh / V Eh / 3 -4 0.1 ++ PCl = 10 UO2 2 Zn++ H S U3O3 2 2 U4+ 0 ZnS 5 (ppt) -1.0 4 (Pu) = 10-4 -0.1 UO2 -2 -1 0 123 (U) = 10-2 } -1.5 pH - 2- ++ (HSO4 ) 1, (SO4 ) 1, (Zn ) 1, -1 -1 ++ (H2S) 10 [PH2S 1], (H2S) 10 , (Zn ) 1, -3 -3 ++ ¼ (H2S) 10 , (H2S) 10 , (Zn ) 1, Fig. 7 E-pO diagram of U-Pu separation in NaCl-KCl equimolar solu- -1 ++ ++ -3 (H2S) 10 , (Zn ) 1, (Zn ) 10 tion. Fig. 5 Eh-pH diagram of ZnS. (i) Zone for H2S generation. (ii) Zone for elemental sulfur formation. (iii) Zone for oxidation to sulfate. salt solvent using chlorine as oxidizing agent, Pu dissolves as 3þ 2þ trivalent (Pu ) and U as hexavalent (UO2 ). When UO2 oxide is subject to electrolytic deposition, it can be separated from Pu (Condition IV of the diagram). When oxidation 100 ¼ A occurs with the coexistence of O donor such as SnO2, only U dissolves without dissolution of Pu, and Pu-U separation 80 B also becomes possible (Conditions I and II of the diagram). Several inorganic chemistry experiment results were described In the AEC Report [HW-62431 (1959)] at the 60 300 time, but to understand such multitudinous phenomenolog- S generated (%) S generated 2 ical explanations in a systematic manner, creation of a 40 200 diagram as Figure 7 was useful.
Details
-
File Typepdf
-
Upload Time-
-
Content LanguagesEnglish
-
Upload UserAnonymous/Not logged-in
-
File Pages7 Page
-
File Size-