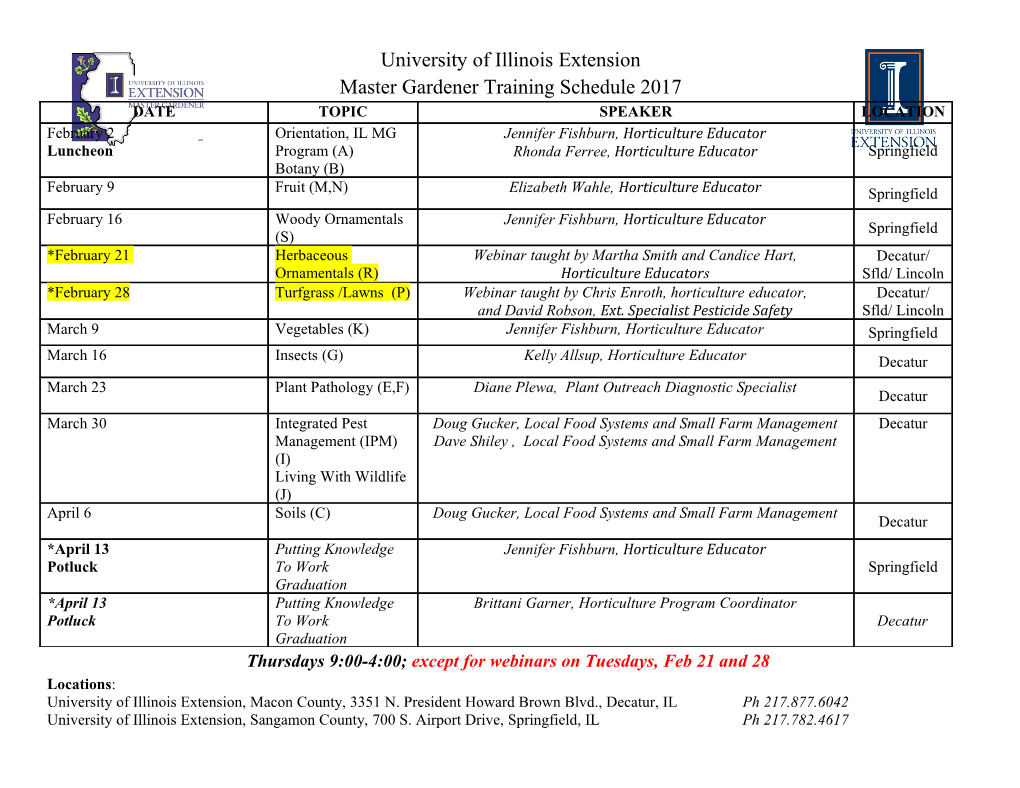
Comparative Tectonics and Geodynamics (2015) 5025.pdf OBSERVATIONAL CONSTRAINTS ON VENUS SURFACE COMPOSITION AND GEOLOGIC HISTORY. M. S. Gilmore, Dept. Earth & Environmental Sciences, Wesleyan University, 265 Church St., Mid- dletown CT 06457 [email protected], www.wesleyan.edu/planetary. Introduction: The Venus atmosphere precludes hematite, the high emissivity anomalies correspond to the visible-near-infrared hyperspectral observations more mafic (less weathered) compositions [10] and the that have revolutionized the study of the surface com- low emissivity anomalies correspond to more felsic position of mars, Mercury and the Moon. Our under- compositions [7, 9, 11]. If the weathering rate of bas- standing of the surface composition the Earth-sized alt to hematite is fast, this suggests the volcanoes are planet Venus is derived wholly from 1) geochemical geologically young [10]. analyses of rocks from 7 landing sites, 2) observations Tesserae – How felsic? The radiance flux corre- of the surface at 1µm through a spectral window in the sponds to low emissivity values for tessera terrain atmosphere. These data are supported by theoretical leading to the following radical conclusions about Ve- and laboratory studies of the weathering, rheology and nus: 1) tesserae have a different (more felsic, less fer- spectra of candidate rocks at Venus temperatures and rous) primary composition. This can include Archean atmospheric composition. – type (TTG) granites formed by dehydration of under- Bulk Composition: The Venera and Vega landers thrust slabs or the lower crust, or true granites which acquired drilled samples (rock + soil) at 7 locations on may require a mature global recycling mechanism in- Venus. Major elements were measured by XRF at 3 troducing water-rich rocks. This may also include an- sites and U/K/Th were measured by γ-ray data at 5 orthosites (or granites) formed via differentiation of sites [1]. All elemental analyses and calculated norma- large mafic intrusions, ala Proterozoic massif anortho- tive mineralogical compositions are consistent with sites. 2) The emissivity values are also consistent with basalt, specifically MORB and high-K alkaline basalts mafic rocks that covered with low emissivity weather- [2]. The landers lie with geologic units mapped as lava ing products formed under a different weathering re- flows that comprise plains that cover the bulk of the gime than the plains. The lack of water today predicts surface of Venus [3]. Thus it is generally accepted that that basalts may weather to hematite and minor anhy- the Venus surface is generally basaltic melts derived drite [e.g., 12]. However, an elevated atmospheric from the mantle. PH2O (perhaps due to enhanced volcanic outgassing) Mineralogy: Radiation observed through 1.0, 1.1, would drive this reaction to completion. Tesserae may and 1.18 µm near-infrared spectral windows in the have formed on an extinct Venus with an active hydro- Venus atmosphere windows contains a large compo- logical cycle producing phyllosilicate minerals whose nent of surface emission [e.g., 4]. In common silicate dehydrated forms may persist today. 3) The lower minerals, 1 µm reflectance is controlled by crystal field emissivity data are also consistent with tesserae have a spectra of transition metal ions in the crystal lattice. different primary grain size: if igneous, they could be As Fe2+ is the most common transition metal in sili- glassy, or have higher porosity [e.g., 13], or 4) they cates, 1 µm reflectance is dominated by and positively could be non-igneous materials. Note that quartz-rich correlated to ferrous iron content, where olivine and rheologies are prohibited by models of buckle folding pyroxenes have low reflectance, and quartz and feld- for the tesserae [14]. Each of these scenarios requires spars have high reflectance [e.g., 5]; this translates to a that the tesserae have experienced a different and ex- lower emissivity, e, via Kirchoff’s Law: e=1-r, where r tinct geological history than the plains. = reflectivity, typically of room-temperature library References: [1] Surkov et al. (1984) Proc 15th minerals. Measurements of the emissivity of rocks and LPSC, 393 (1986 JGR 91, 215; (1987) JGR 92, 537. minerals at Venus temperatures are underway and are [2] Barsukov et al., (1986) Geochem. Int. 23, 53. [3] necessary to accurately interpret 1 µm radiation [e.g., Weitz & Basilevsky (1993) JGR 98, 17069. [4] Mead- 6]. ows & Crisp (1996) JGR 101, 4595. [5] Hunt & Salis- Observations of Venus made by VEx VIRTIS [7] bury Mod. Geol. 1, 283. [6] Helbert et al. (2013) EPSL (corroborated by VEx VMC [8] and Galileo NIMS [9]) 252, 371. [7] Mueller et al. (2008) JGR 113, E00B17; show surface radiance with a spatial resolution of ≥50 Helbert et al. (2008) GRL 35, L11201. [8] Basilevsky km of the southern hemisphere, which is dominated by et al. (2012) Icarus 217, 434. [9] Hashimoto et al. plains. Regions of higher than average emissivity cor- (2008) JGR 113, E00B24. [10] Smrekar et al. (2010) respond to volcanoes and Lada Terra flows [7] and Science 328, 605. [11] Gilmore et al. Icarus, in revi- areas of lower than average emissivity correspond to sion. [12] Fegley & Treiman (1992) AGU Monograph tesserae [7, 9]. The average emissivity values as cal- 66, 7. [13] Harloff & Arnold (2001) PSS 49, 191. [14] culated via Kirchoff’s Law are consistent with basalt + Brown & Grimm (1997) EPSL 147, 1. .
Details
-
File Typepdf
-
Upload Time-
-
Content LanguagesEnglish
-
Upload UserAnonymous/Not logged-in
-
File Pages1 Page
-
File Size-