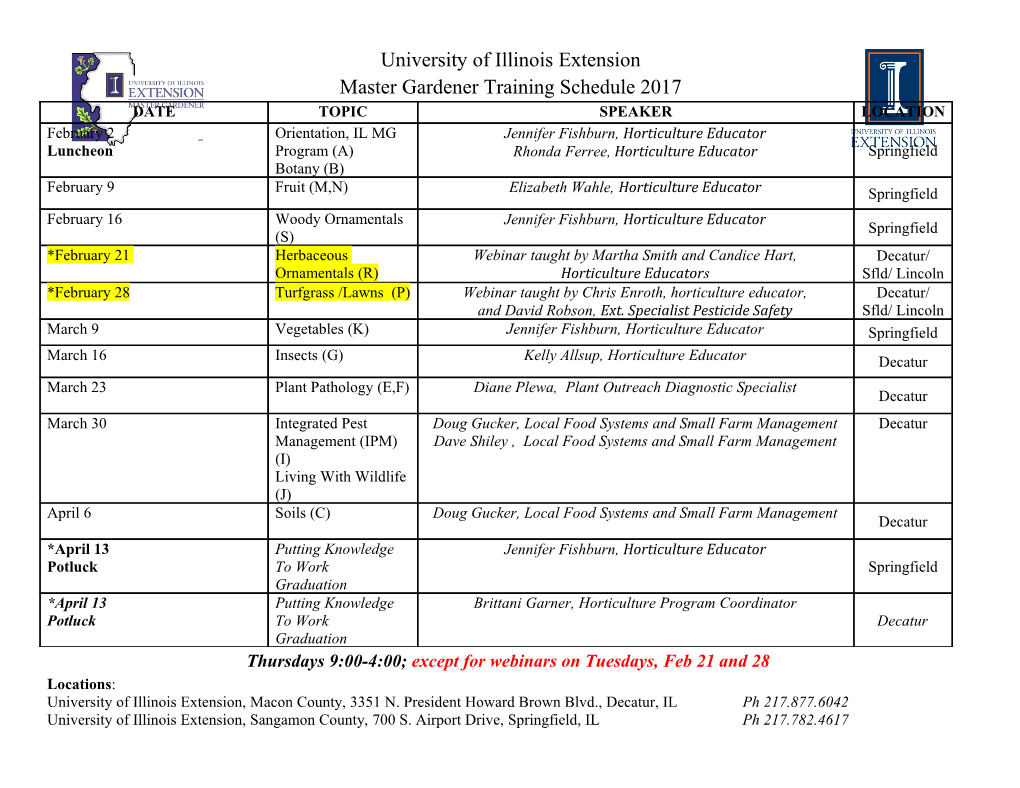
AGRICULTURAL AND FOOD SCIENCE A. Morgenstern et al. (2014) 23: 207–219 Changes in content of major phenolic compounds during leaf development of sea buckthorn (Hippophaë rhamnoides L.) Anne Morgenstern1,2 , Anders Ekholm1, Petra Scheewe2 and Kimmo Rumpunen2,* 1Swedish University of Agricultural Sciences, Department of Plant Breeding, Balsgård, Fjälkestadsvägen 459, 291 94 Kristianstad, Sweden 2 Hochschule für Technik und Wirtschaft Dresden (University of Applied Sciences), Faculty of Agriculture and Landscape Management, Pillnitzer Platz 2, 01326 Dresden, Germany * e-mail: [email protected] Content of total phenolic compounds and antioxidant capacity (FRAP) were investigated in the leaves of three sea buckthorn (Hippophaë rhamnoides L.) cultivars: ‘Otradnaja’, ‘Gibrid Pertjika’ and ‘Ljubitelskaja’, at different dates. In addition, major phenolic compounds (catechin, kaempferol, quercetin, epigallocatechin, kaempferol-3-O-glu- coside, quercetin-3-O-galactoside, isorhamnetin-3-O-glucoside, rutin, gallic acid, procyanidin monomer glycoside, procyanidin dimer aglycone and hydrolyzable tannins I–III) were determined in ‘Ljubitelskaja’. Antioxidant capacity and the content of total phenolic compounds fluctuated during April, May and June, and then increased until the end of July, when the highest values were observed. Total phenolic compounds were strongly correlated with FRAP. Levels were generally higher in ‘Ljubitelskaja’ than in ‘Otradnaja’ and ‘Gibrid Pertjika’. In ‘Ljubitelskaja’, hydrolyzable tannins I–III occurred in higher amounts than did any of the other studied phenolic compounds. The developmen- tal stage of the leaves (harvesting date) had a strong influence on content of phenolic compounds and should be carefully considered when harvesting sea buckthorn leaves for different purposes. Key words: antioxidant capacity, flavonoids, FRAP, polyphenols, tannins Introduction Sea buckthorn (Hippophaë L.) belongs to the family Elaeagnaceae. The most widely distributed species, H. rham- noides, occurs in Central and Northern regions of Europe and Asia, and is divided into eight subspecies (Swenson and Bartish 2003). The plant is a thorny shrub, which can reach approx. six meters in height. About 90% of the genetic resources are located in China, but northern India, Russia and Mongolia are also rich in sea buckthorn (Singh 2003, Erkkola and Yang 2003, Ruan et al. 2013). The sun-loving plant grows in mountainous and coastal ar- eas on well-drained soils, and it is well adapted to dry conditions. Sea buckthorn is often used as a pioneer plant to counteract soil erosion and to improve soil fertility through its N2 fixation capacity (Singh 2003, Qinxiao and Hongyan 2003). These features, together with the valuable fruits, make sea buckthorn an interesting multipur- pose crop for sustainable agriculture. Sea buckthorn fruits are important sources of vitamins, natural antioxidants, oils, triterpenes and other bioac- tive substances (Singh 2006, Geetha et al. 2002, Tsybikova et al. 2006). Both fruits (Rösch et al. 2003) and leaves (Yoshida et al. 1991, Heinäaho et al. 2006, Zu et al. 2006, Moilanen et al. 2013) are rich in polyphenols, and are part of Asian traditional medicine for treatment of, e.g., skin disorders (Upadhyay et al. 2011, Erkkola and Yang, 2003). Beneficial effects of sea buckthorn fruits have been documented through in vitro studies, animal trials and clinical intervention studies. Thus sea buckthorn pulp oil can inhibit platelet aggregation (Johansson et al. 2000), improve atopic dermatitis (Yang et al. 1999) and alleviate symptoms connected with dry eyes (Larmo et al. 2010) in humans. Sea buckthorn puree can lower the plasma high-sensitivity c-reactive protein level (Larmo et al. 2008), which is a marker for inflammation and a risk factor for cardiovascular disease. Furthermore, ethanol-soluble com- ponents of sea buckthorn fruits have been shown to affect postprandial hyperglycemia and insulin response (Le- htonen et al. 2010a) and the beneficial flavonols of sea buckthorn berries have proven to be highly bioavailable (Larmo et al. 2009, Lehtonen et al. 2010b). Manuscript received January 2014 207 AGRICULTURAL AND FOOD SCIENCE A. Morgenstern et al. (2014) 23: 207–219 In addition to fruits, also sea buckthorn leaves have potential beneficial effects. In animal models an aqueous ex- tract of sea buckthorn leaves has shown adaptogenic properties (Saggu et al. 2007, Saggu and Kumar 2008) and has proven especially efficient for wound healing (Upadhyay et al. 2011), an effect that in part may be attributed to extractable polyphenols (Upadhyay et al. 2010). In vitro studies have, in addition to anti-inflammatory (Jayashan- kar et al. 2012) and free radical scavenging effects (Padwad et al. 2006), revealed strong anti-viral (Tolkachev and Sheichenko 2006, Jain et al. 2008) and α-glucosidase inhibitory effects (Kim et al. 2011) of leaf extracts that also may be attributed to the content of polyphenols. Detailed knowledge about factors influencing the content of sea buckthorn leaf phytochemicals is therefore much needed. Effects of different organic farming methods on the content of phenolic compounds in sea buckthorn leaves have previously been investigated on cultivars of H. rhamnoides subsp. rhamnoides (Heinäaho et al. 2006). Arimboor et al. (2008) studied the content of phenolic acids in leaves of H. rhamnoides subsp. turkestanica and Rongfu et al. (2003) studied the seasonal changes in content of total flavones in leaves of H. rhamnoides subsp. sinensis. In this study we investigate the influence of development stage of sea buckthorn leaves of H. rhamnoides hybrid cultivars on the total antioxidant capacity, total phenolic compounds, and on the content of major single pheno- lic compounds. Material and methods Plant material Leaf samples were collected from three sea buckthorn (H. rhamnoides) cultivars (‘Gibrid Pertjika’, ‘Otradnaja’ and ‘Ljubitelskaja’) of Russian origin grown at Balsgård, Kristianstad, in the south of Sweden. The plants were grown in a randomized field trial in three blocks, surrounded by H. rhamnoides seedlings as border plants. Each block contained nine plants per cultivar, and the trial was planted in 1997. The plants were not pruned, irrigated or fer- tilized during the sampling period. Grass was used as mulch. The leaves were sampled on eight occasions at an interval of two weeks from the end of April to the end of July in 2007: April 23, May 7, May 21, June 4, June 18, July 2, July 16 and July 30. On each sampling date, 12 leaves from each of two plants per cultivar and block were sampled to obtain a repre- sentative sample. Leaves were picked from the middle part of the branches. All samples of a cultivar were then pooled before subsampling for biochemical analyses. Measurements and preparation of leaves Leaf length was measured, and the fresh and dry weight was determined (in triplicates) before and after freeze-dry- ing. Dry matter of leaves was calculated, and the freeze-dried leaves were then milled to a fine powder with an IKA® type A10 mill, the powder was stored frozen at −20 °C until used for analyses of total antioxidant capacity and total phenolic compounds. For ‘Ljubitelskaja’ major single phenolic compounds were also analyzed. FRAP analysis of antioxidant capacity Analysis of antioxidant capacity was undertaken with the FRAP (Ferric Reducing Ability of Plasma) method accord- ing to Benzie and Strain (1996) with minor modifications. Leaf powder (25 mg) was extracted in duplicate with 1 ml of 90% MeOH. After a 15 min extraction in ultrasonic bath the samples were centrifuged for 10 min. The super- natant was then diluted 50 times by mixing an aliquot of 20 µl with 980 µl of 90% MeOH. A reagent solution was prepared as follows: 100 ml of acetate buffer solution (pH 3.61) was mixed with 10 ml of 2, 4, 6-Tris(2-pyridyl)-s- triazine (TPTZ) solution and 10 ml of iron(III) chloride hexahydrate (Fe3+) solution. The TPTZ solution consisted of 312 mg of TPTZ powder (Fluka) mixed with 100 ml of 40 mM HCl (30%, Suprapur®, Merck) in an ultrasonic bath for 3 min. A 20 mM Fe³+ solution was made from iron(III) chloride hexahydrate (Fluka) and a stock solution of 2 mM Fe2+ was made from iron(II) sulfate heptahydrate (Fluka) using distilled water. Then standards of 800, 400 and 200 µM Fe2+ solutions were prepared. Absorbance was recorded at 593 nm following a 240 s reaction period. The re- agent solution was kept in a water bath at 37 °C during the whole time. For analysis, 1 ml of the reagent solution was put into a 10 × 4 × 45 mm cuvette set in a Shimadzu (UV-2101PC) spectrophotometer, 10 µl of the standard solution or the sample were then added and mixed with the reagent solution in the cuvette. Each diluted sample was analyzed in triplicate against a blank that contained only the reagent. 208 AGRICULTURAL AND FOOD SCIENCE A. Morgenstern et al. (2014) 23: 207–219 Total phenolic compounds analysis Total phenolic compounds analysis (TP) was conducted with Folin–Ciocalteu’s reagent (BDH) according to Single- ton and Rosst (1965) with minor modifications. Leaf powder (25 mg) was extracted in duplicates with 1 ml of 50% EtOH (containing 0.338% of orthophosphoric acid), ultrasonicated for 15 min and centrifuged for 10 min. Then the extracts were diluted four times by mixing an aliquot of 250 µl with 750 µl of 50% EtOH solution. Next, 10 µl of each diluted extract was mixed with 100 µl of 5% aqueous EtOH, 2 ml of 15% sodium carbonate monohy- -1 drate (175.05 g l of Na2CO3•H2O), 200 µl of Folin–Ciocalteu’s phenol reagent and 1 ml of distilled water in a 10 x 10 x 45 mm cuvette. The samples were kept at room temperature for 2 h before spectrophotometric analysis. A standard stock solution of 705 µM gallic acid in 5% EtOH was prepared and diluted to make a 5 point standard curve for quantification. Absorbance was recorded at 765 nm using a Shimadzu (UV-2101PC) spectrophotometer.
Details
-
File Typepdf
-
Upload Time-
-
Content LanguagesEnglish
-
Upload UserAnonymous/Not logged-in
-
File Pages13 Page
-
File Size-