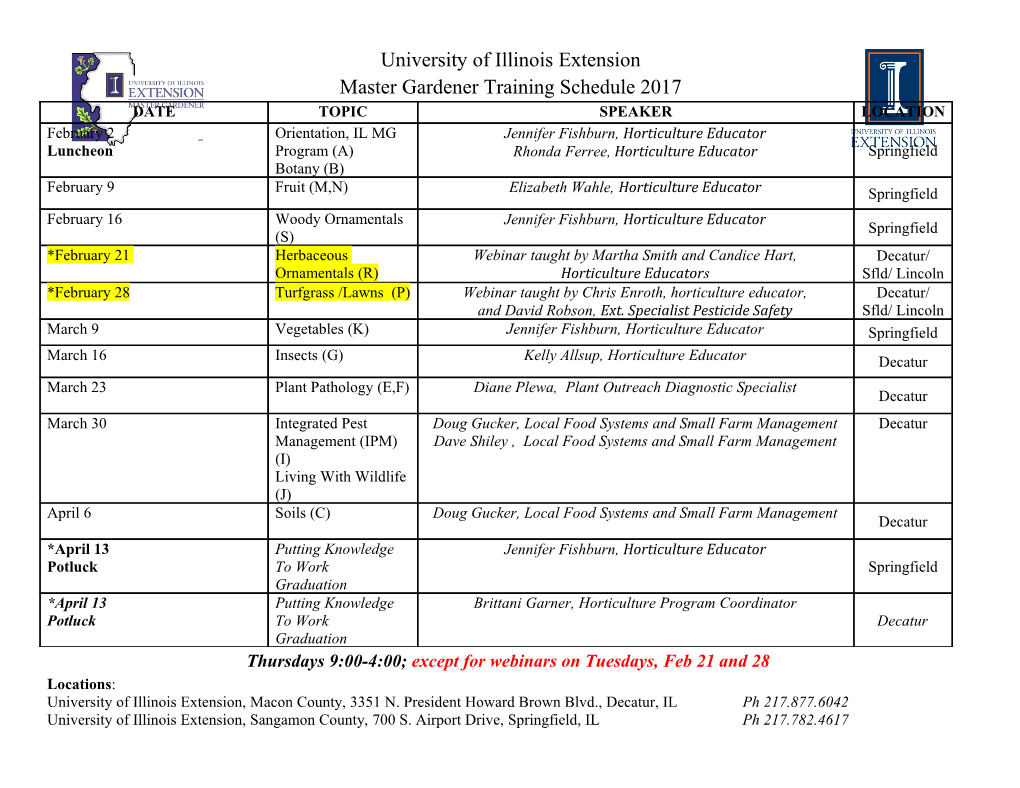
University of Groningen Bachelor Thesis in Physics Research performed at SRON Design and characterization of a LTS flux pump system for possible satellite application Supervisor: Author: dr. ir. G. de Lange (SRON) Laurens Even prof. dr. ir. R.A. Hoekstra (RUG) prof. dr. T. Banerjee (RUG) July 8, 2016 [email protected] student number 2315963 Abstract In this bachelor project research is performed on the design of a prototype low temperature superconducting (LTS) flux pump system. With such a system a superconducting electromagnet can be charged to high currents, while only a low current power supply and cryogenic wiring is necessary. The flux pump could find potential as an application in the SAFARI instrument for the SPICA satellite, but it can also be used for cryogenic energy storage or for offsetting a magnetic field. As a satellite application the flux pump can reduce parasitic heat load on the cold stages of the satellite. Within this thesis we continued the work on an existing flux pump design from a previous bachelor project. This design had previously shown some essential performance characteristics of a flux pump system, but the actual flux pumping was not observed. In this thesis work we investigated the possible causes of this and implemented improvements. These improvements have resulted in a working flux pump system. The main improvement (which was found in a late stage of the project) was the correction of the winding orientation of a secondary coil in the transformer. Other improvements are: (1) the optimization of the transformer cooling, such that higher critical currents in the primary transformer superconducting wire could be achieved, and (2) the increase of the self-inductance of the primary coil of the transformer by replacing the original aluminum transformer core (that will induce eddy currents) by a Vespel polyamide core. For the characterization of the transformer performance we have used a two-phase lock-in measurement technique to determine the self-inductance and mutual inductance of the primary and secondary coils as a function of frequency. The final flux pumping system (V3.0) operates at 4 K, and consists of a transformer with a 4950 turn primary coil (Lp = 4;4 mH self-inductance), a 2 x 10 turn secondary coil (Ls = 0;5 µH, and a 900 turn load coil with an estimated self-inductance around 13;5 mH), all made with supercon- ducting wire. The current in the load coil is monitored by measuring the magnetic field that is generated by the coil with a fluxgate meter. The primary coil has been operated with a maximum current (ramp) of 0;5 A (500 mA/s). The measured and calculated current gain of the flux pump are both around 0;16 mA per cycle for a 50 mA primary current. With this system a current of at least several amperes should be achievable in the load coil (resulting in a magnetic field in the order of at least dozens of milliteslas), but we have limited ourselves to a maximum of 60 mA, because of limitations in the range of the flux gate sensor (not higher than 590 µT). The coupling factor (0 ≤ k ≤ 1) describes the coupling between the primary and secondary side in a transformer, where a value close to 1 would be ideal. For the final flux pumping system k is estimated to be around 0,45. Acknowledgments I would like to thank the following people who helped and supported me during my Bachelor Project that I worked on at SRON: 3 SRON supervisor Gert de Lange for his help on understanding the basics of flux pump systems, its components and the measuring equipment involved in the measurements. Also for his advice on relevant research items and important items to look at. 3 Willem-Jan Vreeling for his help with the laboratory instruments, cryostat operations and helping me understand the LabVIEW programming language to control the flux pump. 3 Axel Detrain for helping me to understand the basics of measuring and determining the characteristics of electromagnetic coils and also for his help in analyzing some of the data. 3 Duc van Nguyen, Rob van der Schuur and Jarno Panman for their help with fabricating parts needed for the flux pump project. Extra thanks to Duc for his comprehensive work related to the soldering of wires and attaching parts together. 3 RUG supervisors Prof. Dr. Ir. R.A. Hoekstra and prof. dr. T. Banerjee for supporting me during my project. 3 Wim van den Berg for his continuous support related to questions I had about his former research on the flux pump system. Special thanks for his one day revisit at SRON. During which he helped me put the improved system (V3.0) together and also at doing the first (and last) measurements on the improvement flux pump system. This was almost at the end of my bachelor project, but luckily it was still in time. ii Contents abstract i Acknowledgments ii 1 Introduction 1 1.1 Origin of this research . .1 1.2 Topic of this research . .1 1.3 Research questions and aim of this research . .2 1.3.1 Research aim 1 . .2 1.3.2 Research aim 2 . .3 1.3.3 Research aim 3 . .3 1.4 Thesis outline . .3 2 Theoretical Background 5 2.1 Superconductivity in short . .5 2.2 Flux pump . .6 2.2.1 Half and full wave rectifier flux pump operation . .8 2.2.2 Description of the flux pump system used in this research project . .9 2.2.3 Flux pumping quantified . 10 2.2.4 Coupling factor, mutual inductance and stray fields . 12 2.2.5 Flux pump operation example . 13 2.3 Two and four terminal resistance measurement methods (sensors) . 13 3 Experimental Methods/setup 15 3.1 Description of flux pump setup V2.0 and V3.0 . 15 3.2 Self-inductance values of the coils present in setup V2.0 and V3.0 . 18 3.3 Expected relation between load coil current and measured magnetic field strength 19 3.4 Description of three self-made coils/transformers . 19 4 Results & Discussion 21 4.1 Measurements on self-made coils/transformers . 21 4.1.1 General remarks about the measurements on coil 1 and 2. 21 4.1.2 Coil 1 (aluminum) and coil 2 (Vespel) with air core . 22 4.1.3 Coil 1 and coil 2 with iron material in core . 22 4.1.4 Coil 1 and 2 put in liquid nitrogen . 22 4.1.5 Coil 3 with and without ferrite (high permeability) core . 22 4.1.6 Summary about test coils . 22 4.2 Expected transformer coupling factor and (current) gain . 23 4.3 Repeated experiments on flux pump setup V2.0 . 24 4.4 Lock-in amplifier frequency sweep measurements analysis and comparison with LTspice IV simulations . 25 iii 4.5 Flux pump cryostat measurements . 25 4.5.1 Persistent current test . 26 4.5.2 Trouble with the fluxgate meter . 26 4.5.3 Calibration fluxgate meter (Bartington) output in µT with current magnitude 27 4.5.4 Heat switches & persistent current test (commutation) . 28 4.5.5 Flux pumping fast to 60 mA (590 µT) ..................... 30 4.5.6 Flux pumping slow to 60 mA (590 µT)..................... 32 4.5.7 Critical temperature determination of the load coil during system warm up 33 5 Conclusions and recommendations for future work 34 5.1 Research aims and questions . 34 5.1.1 Research aims 1 and 2 . 34 5.1.2 Research aim 3 . 34 5.2 Recommendations for future improvements . 35 References 37 Appendices 39 A Measurements of test coil parameters 40 A.1 Test coils 1 and 2 - inner air core left empty . 40 A.2 Test coils 1 and 2 and inner core filled with some magnetic iron material . 41 A.3 Test coils 1 and 2 - in liquid nitrogen . 41 A.4 Test coil 3 - with & without an iron core/casing . 42 B Results lock-in amplifier and simulation with LTspice IV 43 C LabVIEW control and instrument setup of the flux pump system 46 D Possible magnetic bearing setup for the FTS 47 E Primary coils V1.0, V2.0 and V3.0 48 F Pinout flux pump experiment (setup V3.0) 49 iv Chapter 1 Introduction 1.1 Origin of this research SRON, Netherlands Institute for Space Research, focuses on building technology and advanced space instruments for use in astrophysical, Earth science and exoplanetary research. SRON, together with ESA (European Space Agency) and JAXA (Japanese Space Agency), is working on the SAFARI instrument which is an infrared spectrometer. It is to be included in the SPICA satellite (Space Infrared Telescope for Cosmology and Astrophysics) and launched to space in 2028, on one of JAXA's launch vehicles [1][2]. The original plan for the SAFARI instrument on the SPICA satellite was to have the incoming light analyzed by a Fourier Transform Spectrometer (FTS). The FTS includes a movable mirror which is suspended in magnetic bearings with a magnetic linear stage providing the ability for the mirror to move (see appendix D for possible applications). In order to test this system on earth the magnetic bearings need to provide a stronger magnetic field than required in space due to the earth gravitational force. This is called ’1G-off-loading’ and it can be implemented such that the mirror is magnetically levitated and free to be controlled by the linear magnetic stage. The mirror will also be cooled to almost absolute zero (cryogenic) temperature (−273 ◦C) to minimize background noise (heat radiation) and to improve experimental results.
Details
-
File Typepdf
-
Upload Time-
-
Content LanguagesEnglish
-
Upload UserAnonymous/Not logged-in
-
File Pages55 Page
-
File Size-