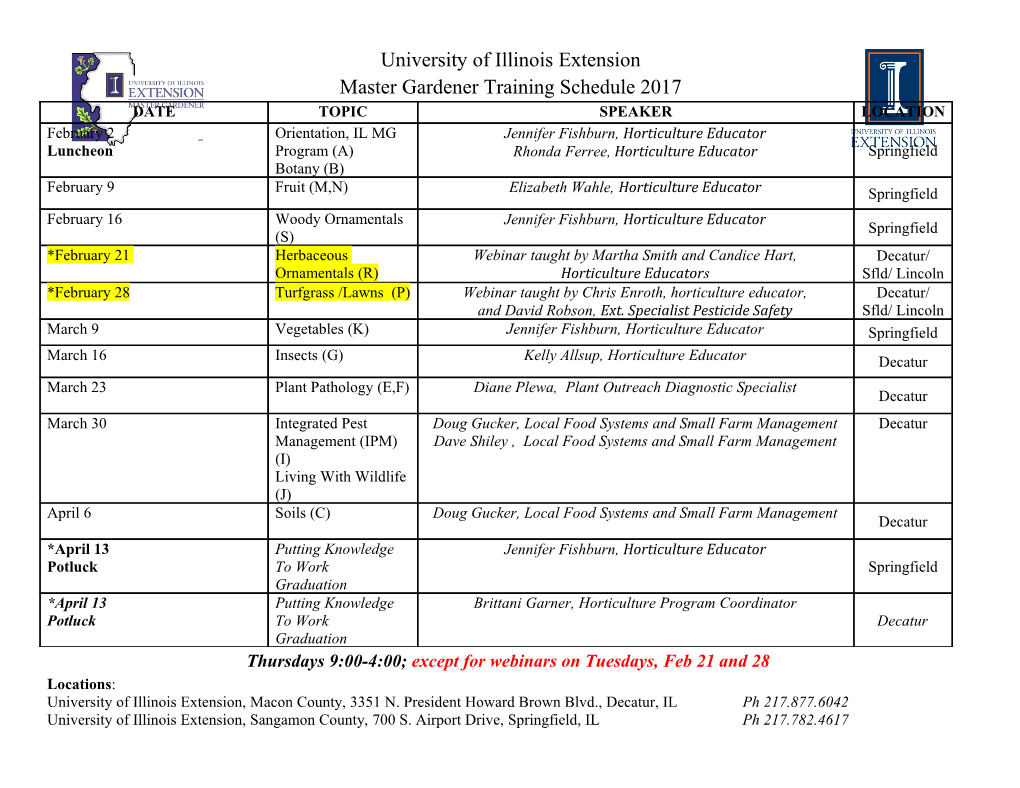
ANALYSIS OF LIGHT-INDUCED IMMEDIATE-EARLY GENE EXPRESSION IN THE SUPRACHIASMATIC NUCLEUS A thesis submitted to Kent State University in partial fulfillment of the requirements for the degree of Master of Science By, Amanda S. Ohnmeiss August 2009 Thesis written by Amanda S. Ohnmeiss B.S. Allegheny College, 2007 M.S., Kent State University, 2009 Approved by _____ Dr. E. Mintz _______________, Advisor ____ Dr. R. Dorman ______________, Director, School of Biomedical Sciences ___Dr. T. Moerland______________, Dean, College of Arts and Sciences ii TABLE OF CONTENTS PAGE LIST OF FIGURES ………………………………………………………………………v LIST OF TABLES………………………………………………………….………….…vi ACKNOWLEDGEMENTS………………………………………………………..…….vii Introduction……………………………………………………….........................1 Suprachiasmatic Nucleus Background…………………........................................1 Entrainment………………………………………………………………………..2 Common Light-Induced Genes of the SCN……………………………………….3 Glutamate………………………………………...………………………..8 Goals………………………………………………………………………9 Methods…………………………………………………………………….…….13 Animals.....................................................................................................13 Experiment 1……………………………………………………………..13 Experiment 2……………………………………………………………..14 Experiment 3………………………………………………………….….14 Experiment 4………………………………………………………….….15 Immunohistochemistry……………………………………………..……15 Data Analysis…………………………………………………………….16 Results…………………………………………………………………………....18 iii Discussion………………………………………………………………………..38 References……………………………………………………………………….47 iv LIST OF FIGURES PAGE Introduction Fig 1: MAPK sequence of events…………………………………………………6 Fig 2: Summary of light induced effects in the SCN………………………….....12 Methods Fig 3: Representative diagrams of SCN division for cell counting……………...17 Results Fig 4: Experiment 1 c-fos expression……………………………………………19 Fig 5: Experiment 2 c-fos expression……………………………………………21 Fig 6: Experment 3 actograms of vehicle/MK-801 injected animals……………23 Fig 7: Experiment 4 c-fos expression……………………………………………26 Fig 8: Experiment 4 egr1 expression………………………………………….…29 Fig 9: Effect of MK-801 on c-fos and egr1 expression………………………….31 Fig 10: Grid view of MK-801 early night c-fos expression……………………...32 Fig 11: Grid view of MK-801 late night c-fos expression……………………….33 Fig 12: Grid view of MK-801 late night egr1 expression………………………..34 Fig 13: Experiment 4 p-ERK expression………………………………………...36 v LIST OF TABLES PAGE Table 1: Experiment 4 c-fos expression data……………………………………………27 Table 2: Experiment 4 egr1 expression data…………………………………………….30 Table 3: Experiment 4 p-ERK data……………………………………………………...37 vi ACKNOWLEDGEMENTS I would like to start by thanking my advisor, Eric Mintz, for his guidance. Where my determination may have been lacking at times, his determination to see his students excel pushed me to complete my research. I have a profound respect for his advising style, as it has prepared me to enter the workforce with knowledge of the importance of self-motivation and holding myself accountable for my work and work ethic. I appreciate his patience, along with the past and present members of the lab (Erin Gilbert, Veronica Porterfield, George Kallingal, and Linley Moreland), in teaching me the techniques I needed to succeed and never finding any question too ridiculous to answer. I would also like to thank the members of my committee for believing that I could finish my research in a year when I came to them after joining Dr. Mintz’s lab in January of 2008. A year and a half later they were ready to advise me as I prepared to defend my thesis. They have educated me in other areas of the field of Neuroscience with passion for their concentrations and, for that, I greatly admire them. I will forever be grateful to my parents for forgiving me for deciding not to go to medical school and supporting my decision to join the Kent State University Biomedical Sciences program. They have always had the utmost faith in my abilities (perhaps more so than I) and continue to be 100% supportive of my decisions in all aspects of my life, whether they agree with them or not. Without them, I would be nothing and I hope that they know how much I truly appreciate their opinions and advice. vii Lastly, I’d like to thank my fiancée, Brian, for encouraging me whenever I became overwhelmed or cranky. He refused to let me give up and pushed me to work hard and prove to myself that I could do it. viii Introduction: Rhythms are present in almost every aspect of the environment; be it seasonal weather changes or fluctuations in day length. In order to survive it is necessary for mammals to be able to anticipate these rhythms and the patterns by which they fluctuate. These mammals rely on environmental cues in order to synchronize their own circadian (daily) rhythms and data collected to date implicate the suprachiasmatic nucleus (SCN) as the primary mammalian circadian clock. The SCN is a group of approximately 20,000 neurons (Riley & Moore, 1977; van den Pol, 1980; Güldner, 1983), which resides in the hypothalamus adjacent to the third ventricle and just dorsal to the optic chiasm (Cassone et al, 1988). Destruction of the SCN results in a loss of circadian control over endocrine, metabolic, and behavioral rhythms (Abe et al, 1979; Stephan & Zucker, 1972). Rhythms in a previously arrhythmic animal (due to lesioning of the SCN) are restored by transplanting small neural grafts from the suprachiasmatic region of a donor animal (Ralph et al, 1990) and, according to lesion studies done by Harrington et al (1993), it appears that rhythms are exhibited as long as 15-25% of the SCN remains intact. There are also clocks located peripherally in the body, such as those found in the liver and pancreas, that are partially dependent on the SCN for the generation of rhythms (Damiola et al, 2000). Destruction of the SCN disrupts the expression of circadian genes in these peripheral clocks (Reddy et al, 2007). Therefore the SCN uses environmental cues such as light and dark to synchronize 1 2 rhythms that control a variety of physiological and behavioral processes both centrally and peripherally. Entrainment The SCN relies on photic cues to synchronize (entrain) rhythms to the environment and photoreceptors located in the retina are responsible for mediating the entraining effect that light has on the SCN/entire mammalian circadian system. Retinal ganglion cells carry information to the SCN via two pathways. The first is through direct connection via the retinohypothalamic tract (RHT) and the second is indirectly via the intergeniculate leaflet (IGL) (Rusak & Boulos, 1981). Destruction of the IGL results in changes in amplitude of phase shifts resulting from photic stimulation but does not abolish them (Pickard et al, 1987). Destruction of the RHT results in an inability of light to entrain circadian rhythms (Johnson et al, 1988a) which supports a role of the RHT as the primary circuit necessary for photic entrainment. Exposure to light can have significant effects on the behavior of an animal. One such effect is a phase shift, where the animal either begins/ends its activity period earlier or later than it did before the light exposure occurred. Whether a phase shift occurs or not depends on whether the light exposure occurs during the subjective night or the subjective day. Exposure to light during the subjective night will result in a phase shift of activity rhythms whereas a light pulse during the subjective day has no effect (Pittendrigh & Daan, 1976). The direction of the phase shift elicited by exposure to light during the subjective night is also subject to variation depending on which part of the night the light 3 pulse occurs. A light pulse given during the early night will result in a phase delay while a light pulse given during the late night will result in a phase advance (Daan & Pittendrigh, 1976). Entrainment to a new light cycle may take several days depending on how much the new light cycle deviates from the cycle the animal was previously entrained to. Common Light-Induced Genes of the SCN In addition to the behavioral phase shifts during the subjective night, light pulses also result in the expression of immediate early genes (IEGs) (Guido et al, 1999). Immediate early genes are rapidly and transiently expressed in response to a variety of stimuli (for our purposes, to photic stimuli). A light pulse at just any time of the day will not necessarily result in the expression of IEGs in the SCN. Most IEGs will only be expressed during a time when light pulses are expected to cause phase shifts, (i.e. subjective night) (Rusak et al, 1990). Examples of IEGs that are expressed in response to photic stimulation include FBJ osteosarcoma oncogene (c-fos), early growth response protein 1 (egr1), and Jun oncogene (Jun). To date, c-fos is the most studied and well characterized of the IEGs. It is induced by photic stimulation of the retinas (Rea, 1989) and its expression occurs mainly in retinorecipient areas of the SCN (Aronin et al, 1990). C-fos also exhibits its own spontaneous rhythms in the dorsomedial portion of the SCN independent of the effects of light (Sumová et al, 1998). It has been proposed that the increase in c-fos immunoreactivity is the result of an increase in Fos mRNA (Kornhauser et al, 1990). 4 Porterfield et al (2007) have shown that the increase in c-fos expression following a light pulse is approximately 30 fold. In hamsters, the degree to which c-fos expression is induced by photic stimulation is correlated with the behavioral phase shifting effects of light such that brighter light results in larger phase shifts and increased c-fos expression (Lin et al, 1997). Also, when antisense oligonucleotides are used in rats to prevent the translation of c-fos protein, photic phase shifts are inhibited (Wollnik et al, 1995). Each of these pieces of evidence suggests a link between c-fos expression and light induced phase shifts. However, c-fos knockout mice are able to entrain to light dark cycles (Honrado et al, 1996) suggesting that c-fos is not necessary for photic entrainment. Egr1, another IEG, is a DNA-binding transcription factor and is also induced by light (Kornhauser et al, 1990).
Details
-
File Typepdf
-
Upload Time-
-
Content LanguagesEnglish
-
Upload UserAnonymous/Not logged-in
-
File Pages60 Page
-
File Size-