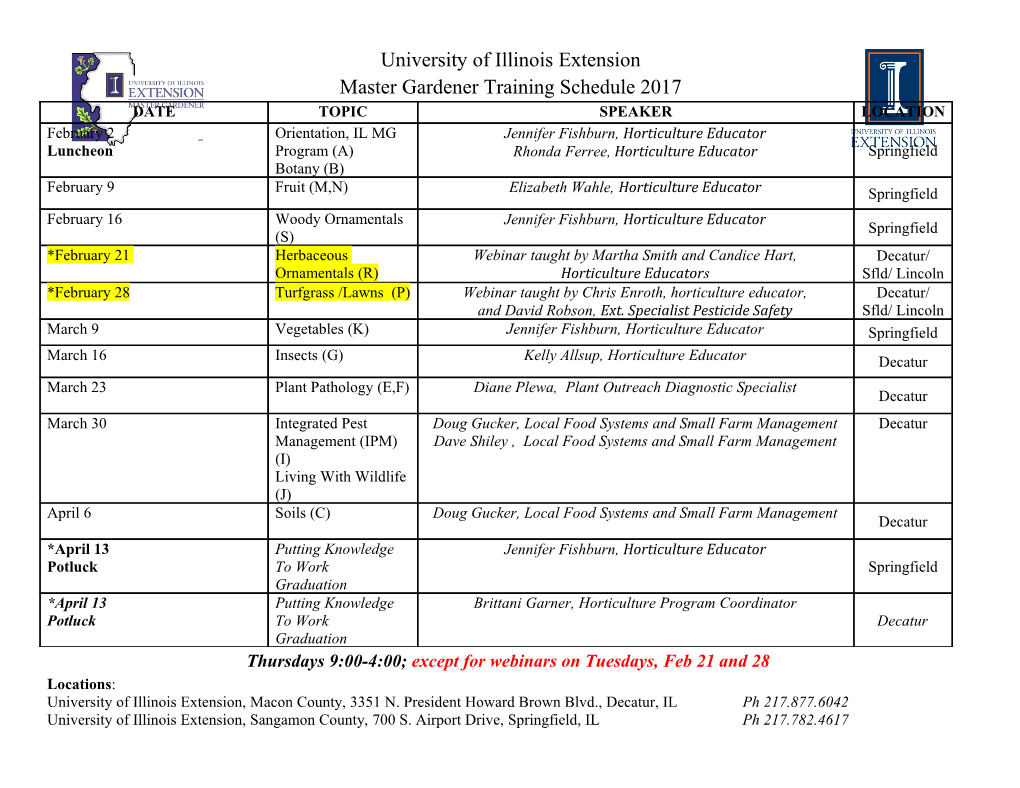
Developmental Neurobiology, edited by Philippe Evrard and Alexandre Minkowski. Nestle Nutrition Workshop Series, Vol. 12. Nestec Ltd., Vevey/Raven Press, Ltd., New York © 1989. Gene Mapping Techniques Jean-Louis Guenet Institut Pasteur, 75724 Paris Cedex 15, France Very accurate gene mapping is essential in both man and laboratory mammals (1- 3). Several techniques have been used over the last 50 years to localize mammalian genes on the chromosomes of a given species. This chapter reviews these tech- niques, with special emphasis on the most recent ones that represent a true break- through in formal genetics. CLASSICAL GENE MAPPING TECHNIQUES AND THEIR LIMITATIONS When two genes are linked they have a tendency to cosegregate during successive generations. The closer the linkage, the more absolute is the cosegregation. This is the fundamental principle of gene mapping, which has been successfully applied to all species, including plants, over many years. In mammals such as humans and mice, the continued discovery of marker genes scattered throughout the genome has facilitated the mapping of new genes so that we now possess for these two species, particularly the mouse, linkage maps that are far more detailed than those existing for other mammals. In the mouse, special matings can be set up, with appropriate stocks, to test for possible autosomal linkage after two successive reproductive rounds: In general, cross-back crosses are used, cross-intercrosses being reserved for studies in which the viability or the fertility of the homozygous mutant under study is impaired. In humans investigations concerning linkage are based on pedigree analysis. In other words, for both species it is essential to define as a starting point a situa- tion where two genes are heterozygous and either in repulsion A + / + B or in cou- pling AB/ + +, then to look for changes in this configuration after a reproductive cycle (forms in coupling giving rise to forms in repulsion and vice versa), and fi- nally to count the percentage or frequency of these recombination events. The smaller the frequency, the tighter is the linkage. At the population level other approaches have been used to test for possible link- age. One such method used in human populations is the lod score, which assesses abnormally high frequencies of association between genes within populations hav- 87 88 GENE MAPPING TECHNIQUES ing common ancestors. In the mouse the use of congenic strains, and especially re- combinant inbred strains (RIS), has proved very useful. The use of RIS for the detection of linkage has been extensively reviewed by Taylor (4), who has consid- ered each strain as the equivalent of an F2 individual with a unique reassociation of parental characters. Here, however, the new genotype is permanent since the strain is inbred. Although such classic techniques have been very useful over the years they share several drawbacks and have in addition two intrinsic major limitations. The drawbacks include their time-consuming nature, particularly in human stud- ies; their expense, since several hundred mice may have to be bred to test for a hy- pothetical linkage or to ensure the necessary precision (since by definition as many recombinants as possible must be scored if tight linkage is to be detected or estab- lished with accuracy); and the fact that many mutant genes cause reductions in via- bility or are not fully expressed in all individuals bearing them. This is particularly likely to happen in the mouse and may prevent the determination of the true segrega- tion ratio, and thus the proportion of recombinants in linkage crosses. The two main limitations arise because: (a) The mapping methodology only al- lows for linkage detection with already known marker genes. By definition, linkage cannot be detected if the gene under investigation is "far away" from all other known genes, (b) The genes coding for invariant products, which by definition have no allelic forms, cannot be mapped using these methods. It is mainly for these reasons that nonsexual techniques have been developed by mammalian geneticists over the last twenty years. These methods use somatic cells of different species grown in vitro and artificially hybridized. Although fundamen- tally different, these new techniques are complementary to the classic ones and should be considered as additions rather than alternatives to the classic mapping technologies. GENE MAPPING WITH SOMATIC CELL HYBRIDS Our knowledge of the mammalian genetic map (and especially the human map) has increased considerably during the past 15 to 20 years as a result of a number of very important advances in cell genetics. Among these were the discovery of human-rodent and rodent-rodent cell hybridization and the study of the segregation of different chromosomes occurring in such hybrids; the development of chromo- some staining techniques (the so-called banding techniques) which permit unambig- uous identification of all human, hamster, and mouse chromosomes at the metaphase stage; and the discovery of several hundreds of biochemical markers in the different species which can be identified and characterized as electrophoretic variants (the so-called electromorphs or allozymes, when the particular protein is an enzyme). At present, every human chromosome and every mouse chromosome has at least one biochemical marker and often many more. These technical advances have per- GENE MAPPING TECHNIQUES 89 mitted tremendous progress in mapping of both human (mostly) and mouse genes. For rapid chromosomal assignment of human genes, a panel consisting of a col- lection of cloned human-rodent cell-hybrids containing specific combinations of hu- man chromosomes can be used. The genes coding for proteins that are specific to the human species can be identified by their product, using special biochemical assays. In parallel, it is also possible to identify individual human chromosomes among the chromosomal set of the cell hybrids. Thus genes coding for unique human markers can be mapped to the individual human chromosome that matches its particular pat- tern in the hybrid panel. In some instances only one chromosome of a given species (humans in general) is present in addition to the normal complement of the partner species of the hybrid, so that a nonambiguous localization of the gene can be made almost immediately. In order to increase such gene assignments, the following efforts have been made: (a) To characterize more gene products physically, chemically, and immunologi- cally in order to distinguish clearly human from rodent products. Monoclonal anti- bodies, electrophoresis, and isoelectrofocalization techniques and study of thermal stability of proteins have been of cardinal importance in this field, (b) To devise methods for inducing gene activities which are not normally expressed in cell hy- brids, (c) To construct additional hybrid panels containing new combinations of hu- man, mouse, or Chinese hamster chromosomes, either as intact chromosomes or partially rearranged with each other, (d) To develop sets of deletion hybrids each containing only one chromosome of a given species, with more or less extensive ter- minal deletion. More than 400 genes have been localized using these somatic cell hybrid tech- niques, and about 40 new genes are mapped each year. It is, however, impossible with these techniques to localize genes coding for monomorphic gene products (those which exhibit no variation between the partners of the cell hybrids); they are also characterized by a certain lack of precision in gene localization due to the lim- ited number of chromosomal breakages available. This results in most assignments being less precise than those obtained using the classic techniques. Finally it must be pointed out that genes whose products are not expressed in vitro cannot be mapped using these techniques. THE USE OF RECOMBINANT DNA PROBES IN GENE MAPPING With the recent development of molecular DNA technology, new techniques have become available for mapping genes. Again, these new techniques are comple- mentary to those already discussed. They involve the use of DNA probes. A DNA probe consists of a small DNA segment, containing a specific sequence of the genome, which is used for hybridization at the molecular level with genomic DNA. To be easily kept and prepared in large quantities for hybridization, the DNA sequence of the probe is incorporated within an autoreplicative structure, either a bacteriophage or a plasmid, prior to hybridization. To be easily recognizable (the 90 GENE MAPPING TECHNIQUES primary role of a probe) the DNA sequence is labeled, usually with a radioactive isotope. Probes containing more or less extensive parts of specific genes are able to hy- bridize with the homologous segment of the chromosome carrying the particular gene if used under appropriate technical conditions. Thus if a radioactive probe is hybridized with a sample of DNA prepared from interspecific cell hybrids segregat- ing for a given set of chromosomes it is possible to identify, at least roughly, where the locus coding for this gene is located by matching the pattern of positive hybrid- izations with the pattern of chromosome presence or absence in the panel (Fig. 1). These techniques represent an important advance in formal genetics because they allow gene detection without requiring their expression. Mapping of the sequences of several important structural genes has been performed using heavily labeled cDNA probes and restricted (cut in small segments)
Details
-
File Typepdf
-
Upload Time-
-
Content LanguagesEnglish
-
Upload UserAnonymous/Not logged-in
-
File Pages8 Page
-
File Size-