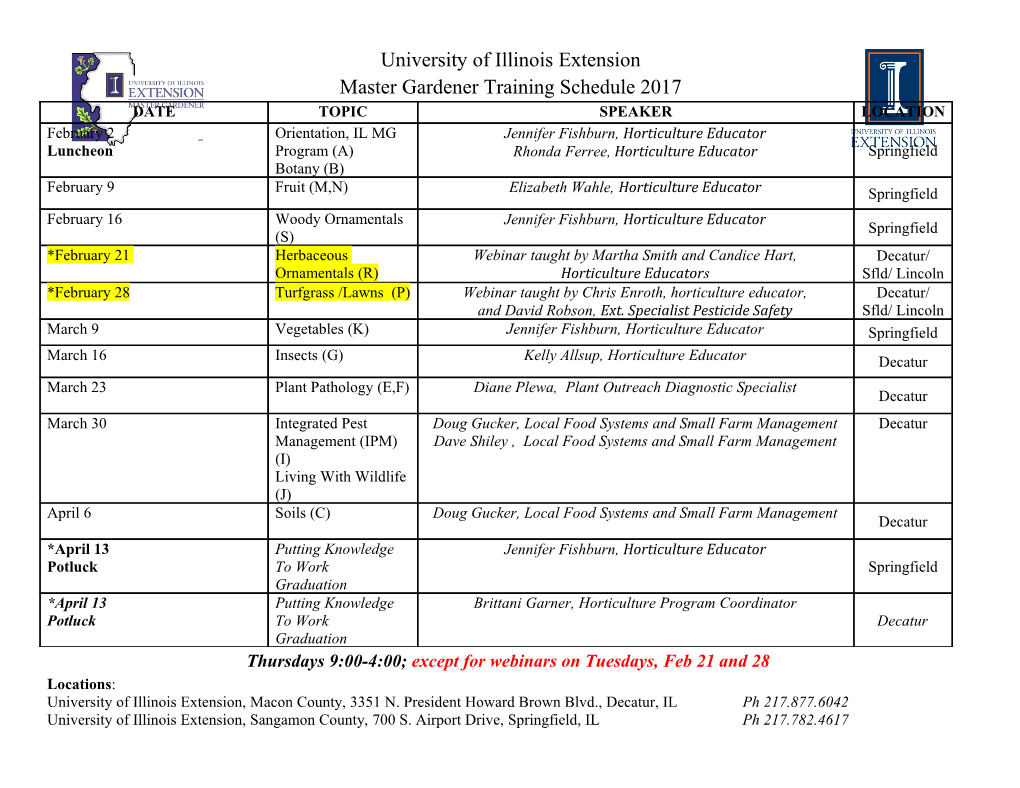
EPSC Abstracts Vol. 14, EPSC2020-501, 2020 https://doi.org/10.5194/epsc2020-501 Europlanet Science Congress 2020 © Author(s) 2021. This work is distributed under the Creative Commons Attribution 4.0 License. Tridymite in Gale crater, Mars: Witness of explosive volcanism in Early Mars? Valerie Payre1, Kirsten L. Siebach1, Michael T. Thorpe1,2, Paula Antoshechkina3, and Elizabeth B. Rampe2 1Rice University, Department of Earth, Environmental and Planetary Sciences, EEPS, United States of America ([email protected]) 2NASA Johnson Space Center, Houston, TX, United States of America 3California Institute of Technology, Pasadena, CA, United States of America Introduction: Tridymite, cristobalite, and quartz are silica polymorphs, i.e., they share the same chemical formula SiO2 but their crystalline structures differ [1]. On Earth, tridymite is stable as a high-temperature hexagonal phase (870<T<1470 °C) and is mainly found in impact and volcanic complexes, crystallizing in hydrothermal or fumarolic environments, within ash plume, or from a melt [1]. Metastable forms of tridymite can exist at lower temperatures (< 450 °C) due to crystalline rearrangement. Metastable monoclinic tridymite is extremely rare on Earth and has only been detected in 5 volcanic and impact locations [2]. On Mars, the Curiosity rover that landed in the 3.8 Ga impact crater Gale surprisingly discovered significant amounts of monoclinic tridymite along with feldspar, cristobalite, magnetite, anhydrite, and Si-rich amorphous material in a lacustrine mudstone target called Buckskin [3]. Initially attributed to silicic volcanism [3], the scarcity of monoclinic tridymite on Earth now raises questions about its formation process on Mars: did hexagonal tridymite first crystallize from a high- temperature environment and then transform to a monoclinic tridymite? Did monoclinic tridymite form directly at low-temperature? A whole set of scenarios can be envisioned to explain the crystallization pathways of monoclinic tridymite, and this study aims to decipher which pathway(s) would be the most plausible. Geological Context:The Buckskin target is a thinly laminated mudstone that is part of a unique >1-m thick SiO2-rich outcrop within the observed 350+ m thick Murray formation [4], which is interpreted to represent a distal lacustrine environment [5]. Highly silicic in composition (SiO2~ 74 wt. %) and strongly depleted in Al2O3 (Al2O3 ~ 5.5 wt. %) [3], Buckskin dramatically differs from more mafic-like compositions measured in most sedimentary rocks along the Curiosity traverse. Methods:Buckskin has been analyzed by X-ray diffraction (XRD) with the CheMin instrument onboard the rover, and XRD patterns revealed the presence of monoclinic tridymite (15%), plagioclase (19%), and minor sanidine (4%), magnetite (3%), cristobalite (3%), and anhydrite (1%), along with a significant amount of a Si-rich amorphous material (44%) that contains opal-A, opal-CT and potential Si-rich glass [3,6]. Note that the XRD pattern of monoclinic tridymite indicates the fully ordered nature of tridymite and significantly differs from any forms of opal (e.g., Fig. 1). Fig. 1. Diffraction pattern of Buckskin mudstone (black line) as observed by CheMin, and opal-CT (yellow line). XRD peaks of monoclinic tridymite (red lines) are shown for comparison. Formation Pathways:On Earth, the rare terrestrial monoclinic tridymite results from the transformation of hexagonal tridymite that crystallized from a silicic magmatic or impact melt [2]. Interestingly, monoclinic tridymite, along with cristobalite, is often observed in meteorites, including martian meteorites, as a product of hexagonal tridymite which formed by magmatic and impact crystallization [7]. Hexagonal tridymite can also be encountered in various settings including pyroclastic flows, ash tuffs, fumarolic and hydrothermal environments. Figure 2 presents the pros and cons of each of the possible formation pathways for the Buckskin sample. Fig. 2. Natural tridymite formation pathways as observed in terrestrial settings and meteorites. One main issue with most of the scenarios is that quartz typically crystallizes due to temperature gradient or slow cooling since quartz is stable at T < 870 °C; however, no quartz has been confidently detected in the lower Murray formation. The second issue is the strikingly low Al2O3 in Buckskin mudstone is hard to reconcile with a silicic origin. The Al-depletion can potentially be explained by a detrital origin: Al could have been depleted during mineral transport by sorting. Fractional crystallization is a process that has been suggested to explain detrital igneous minerals and felsic rock formations from the Bradbury formation, stratigraphically below the Murray formation from which Buckskin was sampled [8-9]. We used the same thermodynamical alphaMELTS models [10-11] that have been used to explain Bradbury igneous minerals [9] to observe whether tridymite was formed from the same melts. Fractional crystallization at pressures of 200 and 500 bars at FMQ +1 can produce tridymite from rhyolitic anhydrous melt, i.e., late stage magmas that previously formed plagioclase and pyroxene analyzed in Bradbury sedimentary rocks. However, no cristobalite is produced. Discussion: According to (extra)-terrestrial observations, monoclinic tridymite is likely a product of hexagonal tridymite. The direct formation of monoclinic tridymite in low-temperature environments cannot be ruled out, although this is not observed in any terrestrial systems. Comparing with well- known settings, the absence of quartz in the Buckskin layer, and the low Al contents suggest that hexagonal tridymite and cristobalite could have been both formed from magmatic crystallization followed by rapid cooling to avoid any quartz crystallization. Although alphaMELTS simulations [10-11] do not predict any cristobalite, this latter could have crystallized at a temperature outside of its stability field, as a metastable form (< 1470 °C; e.g., [1]). A reasonable alternative is that hexagonal tridymite and cristobalite formed from an explosive silicic volcanic eruption, depositing ashes in a watershed that allowed mineral transportation into the ancient lake and the potential tridymite transformation from an hexagonal to a monoclinic form. Rhyolitic glass ashes, potentially from the same magmatic suite as observed for Bradbury igneous materials, may have been altered to opal-A. If true, this would suggest that explosive volcanism on Mars may be not restrained to basaltic systems only. References:[1] Howie, R.A., et al. (1992). (p. 696). Longman; [2] Jackson, J. C., et al. Am. Min. 96.1 (2011): 81-88; [3] Morris, R.V., et al. Proceedings of the National Academy of Sciences 113.26 (2016): 7071-7076; [4] Czarnecki, S., et al. JGR: Planets 125.3 (2020); [5] Hurowitz, J.A., et al. Science 356.6341 (2017); [6] Morrison, S.M., et al. American Mineralogist 103.6 (2018): 857-871; [7] Bridges, J.C., et al. Meteoritics 30.6 (1995): 715-727; [8] Udry, A., et al. JGR: Planets 123.6 (2018): 1525-1540; [9] Payre, V. et al. JGR: Planets, In Review; [10] Ghiorso, M. S., & Sack, R. O. (1995). Contributions to Mineralogy and Petrology, 119(2-3), 197-212; [11] Gualda, G. A., Ghiorso, M. S., Lemons, R. V., & Carley, T. L. (2012). Journal of Petrology, 53(5), 875-890. Powered by TCPDF (www.tcpdf.org).
Details
-
File Typepdf
-
Upload Time-
-
Content LanguagesEnglish
-
Upload UserAnonymous/Not logged-in
-
File Pages3 Page
-
File Size-