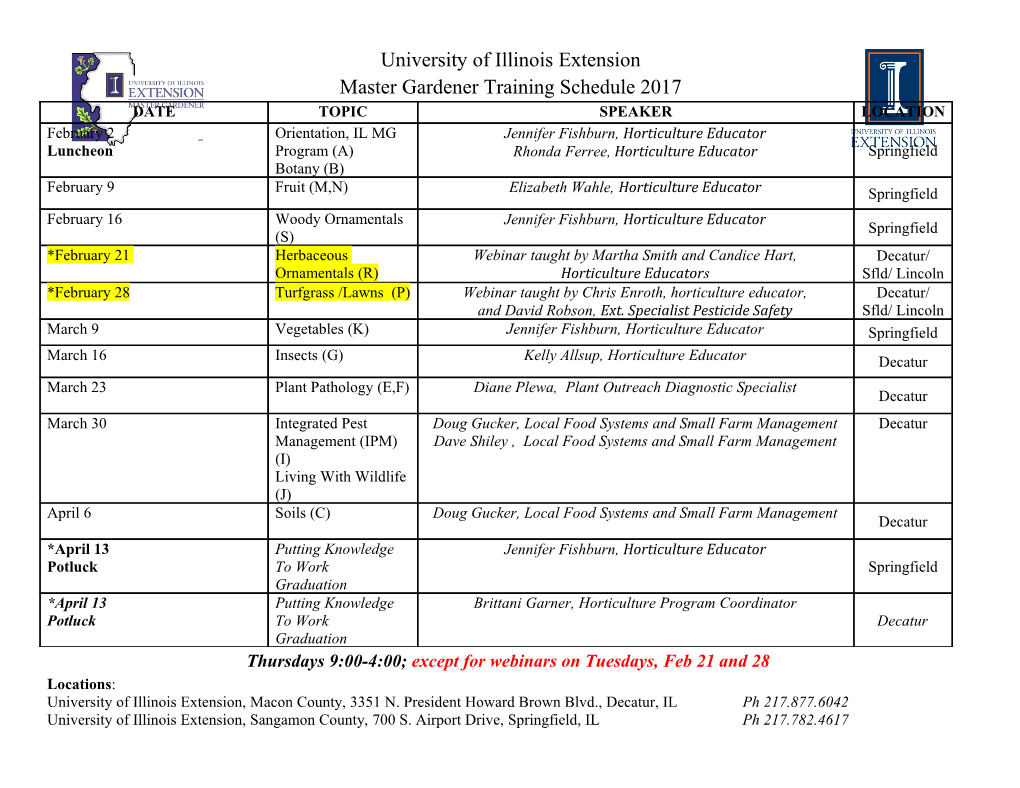
S S symmetry Article Symmetries of Magnetic Fields Driven by Spherical Dynamos of Exoplanets and Their Host Stars Dmitry Sokoloff 1,2,3 , Helmi Malova 4,5 and Egor Yushkov 1,3,5,* 1 Department of Physics, Lomonosov Moscow State University, 119991 Moscow, Russia; [email protected] 2 Institute of Terrestrial Magnetism, Ionosphere and Radio Wave Propagation RAS, 142191 Troitsk, Russia 3 Moscow Center of Fundamental and Applied Mathematics, 119991 Moscow, Russia 4 Skobeltsyn Institute of Nuclear Physics of MSU, 119991 Moscow, Russia; [email protected] 5 Space Research Institute RAS (IKI), 117997 Moscow, Russia * Correspondence: [email protected]; Tel.: +7-916-774-52-72 Received:25 October 2020; Accepted:13 December 2020 ; Published: 15 December 2020 Abstract: Observations of exoplanets open a new area of scientific activity and the structure of exoplanet magnetospheres is an important part of this area. Here we use symmetry arguments and experiences in spherical dynamo modeling to obtain the set of possible magnetic configurations for exoplanets and their corresponding host stars. The main part of our results is that the possible choice is much richer than the basic dipole magnetic field of both exoplanets and stars. Other options, for example, are quadrupole configurations or mixed parity solutions. Expected configurations of current sheets for the above mentioned exoplanet host star systems are presented as well. Keywords: exoplanets and host stars; spherical magnetic dynamo; magnetic field configurations; dipole and quadrupole symmetries; current sheets. 1. Introduction Exoplanets is a new area for scientific investigations that accumulates ideas from various more traditional scientific branches [1–4]. The process of accumulation is far from being straightforward because of the novelty of the topic. In particular, it seems very attractive to suppose that the exoplanets have magnetic fields that are to some extent similar to the geomagnetic field as well as the stars in the planetary system with particular exoplanets having a magnetic field that is to some extent similar to the solar magnetic field [5,6]. In regards to first attempts to observe the magnetic fields of exoplanets see, e.g., [7]. It becomes possible to use for the exoplanet studies, the available bulk knowledge concerning solar magnetosphere, solar wind, and related topics of space physics. The point however is that we know quite a lot about the solar and Earth’s magnetic field from long term studies using various tracers. Of course, we extrapolate the ideas together with available knowledge concerning planetary magnetism in the solar system to the much wider sample of exoplanets. It is far from clear to see in advance how wide a variety of options lost in straightforward extrapolation could be. The available understanding of the magnetic field origin in celestial bodies in the framework of dynamo theory helps in this extrapolation (basic ideas of dynamo theory for astrophysical applications can be found in various books, e.g., [8]). Theoretical abilities, here, are however limited because our knowledge concerning stellar and planetary hydrodynamics required from theoretical prediction is much more limited than one for the Sun and Earth. The point is that the intensive motions of electroconducting media in the form of convection or turbulence and differential rotation seems to be required for dynamo action in exoplanets and their host stars however one needs to know various delicate properties of convection to predict the shape of dynamo-driven configuration. One such Symmetry 2020, 12, 2085; doi:10.3390/sym12122085 www.mdpi.com/journal/symmetry Symmetry 2020, 12, 2085 2 of 13 fine property is the degree of mirror asymmetry of convective flows. Quantification of such values is far from an easy undertaking even for laboratory experiments with liquid metals. The available experiences in laboratory dynamo experiments confirm the point that moderate changes in delicate properties of the flow can result in a substantial modification in dynamo-driven magnetic configuration, see, e.g., [9]. Of course, extensive numerical simulations remain a useful tool for the understanding of exoplanetary dynamos however it looks unrealistic to predict the magnetic configuration based on several integral parameters of an exoplanet. Of course, the magnetic field of an exoplanet similar to Jupiter is expected to be similar to the magnetic field of Jupiter. A non-rotating exoplanet or an exoplanet without any liquid envelope is expected to have no pronounced internal magnetic field. It is natural to expect that a larger rotational moment of a celestial body results in its higher magnetic moments. Stellar activity observations support this expectation (e.g., [10]; remarkably, this early finding in the topic is simultaneously an early paper in exoplanetary studies). However deeper thoughts are required on an approach directed on exoplanet studies specifically. Looking for a way to resolve to some extent the problem, we suggest to base a list of possible magnetic configurations relevant to the problem on the symmetry properties of spherical dynamos as well as experiences in the field of playing parameters in quite a rich history of spherical dynamo modeling. An immediate message from this business (say, [11]) is that the variety of options here is much wider rather than one suggested by a straightforward extrapolation from solar system experiences. The aim of our paper is to present a variety of options known from the experiences in dynamo modeling and briefly explain how dramatic the corresponding changes required could be in the basic concept of magnetospheric studies for exoplanet-star systems. We note that the results from dynamo theory collected below are already discussed in the scientific literature and (we give their corresponding references) however the attention of the authors was concentrated mainly on magnetic configurations typical for the Sun or Earth so it is from easy for a reader to extract the variety of dynamo-driven magnetic configuration from the original papers. As a result researchers interested in current sheets systematically ignore many options. Our paper aims to fill this gap. We appreciate various specific mechanisms like compression of magnetic field, frozen in stellar wind, relic magnetic field, or battery effects which may be responsible for magnetic field formation in some celestial bodies. We concentrate however on the message from dynamo theory as a main stream of explanation in stellar and planetary magnetic studies. It would be risky to insist that dynamo successfully explains any particular observation in the field however the general progress here looks remarkable. 2. Spherical Dynamos As we know the choice of possible mechanisms for magnetic field self-excitation in stars and planets is very limited. If we are not ready to admit new physics to explain magnetic field generation in an isolated celestial body, we have to consider its self-excitation (and then it being self-sustained) by electromagnetic induction. In other words, we have to consider magnetic field self-excitation by a flow of electrically-conducting media. This idea was suggested by Larmor as early as 1919 and the corresponding physical mechanism is known now as a dynamo [12]. Numerous attempts to somehow modify this starting point in dynamo studies remain unsuccessful and we accept that dynamo is responsible for magnetic fields in celestial bodies, ignoring some rare exceptions (say, relic magnetism in some stars [13]) irrelevant for our problem. The point is that according to the so-called Lenz rule, electromagnetic induction acting in an electrical circuit suppresses a seed magnetic field. In order to obtain the magnetic field self-excitation, we have to consider two systems of magnetic lines and a flow which produces an induction effect for one system, which enhances magnetic field in the second one and vice versa (e.g., [14]). Around 2000, the possibility of such self-excitation was demonstrated experimentally in Latvia and later in Germany using a device with moving liquid sodium. From that time, the dynamo process can be considered an Symmetry 2020, 12, 2085 3 of 13 experimental fact rather than theoretical speculation (see, e.g., [9], for review about laboratory dynamo experiments). Several particular ways to obtain a dynamo self-excitation are known, however one that is relevant in stellar and planetary context was suggested by Parker in 1955 [15]. Consider poloidal magnetic field BP which looks more or less similar to the field of one or several magnetic dipoles situated at the rotation axis and toroidal magnetic field BT situated deep under the surface of the body and directed in the azimuthal direction. Then differential rotation produces toroidal magnetic field from the poloidal one. This is one part of dynamo self-excitation. In order to get a dynamo, we have to restore the poloidal magnetic field from the toroidal one. Parker [15] suggested that it occurs due to a mirror asymmetry of the convective or turbulent flows. This mirror asymmetry can be created by various effects however Parker [15] considered Coriolis force acting on convective vortexes in rotating stratified convection. In 1965 Steenbeck, Krause, and Rädler independently developed a consistent theory of this effect known after their work as the a-effect (e.g., [16]). The a-effect was also obtained in laboratory experiments [17] and can be considered now as an experimental fact. Dynamo models based on the joint action of the differential rotation with angular velocity w and the a-effect known as the spherical aw-dynamos are extensively investigated numerically nowadays. The governing equation for magnetic field B averaged over convective pulsations reads: ¶B = curl ([V × B] + aB − b curl(B)) , ¶t where V is large-scale velocity (mainly differential rotation), a is the so-called a-effect which quantifies mirror asymmetry of convective flows, and b is the coefficient of convective magnetic diffusivity. The first term in the r.h.s. of this equation is usually at least 10 times larger than the second one however it is insufficient for dynamo action.
Details
-
File Typepdf
-
Upload Time-
-
Content LanguagesEnglish
-
Upload UserAnonymous/Not logged-in
-
File Pages13 Page
-
File Size-