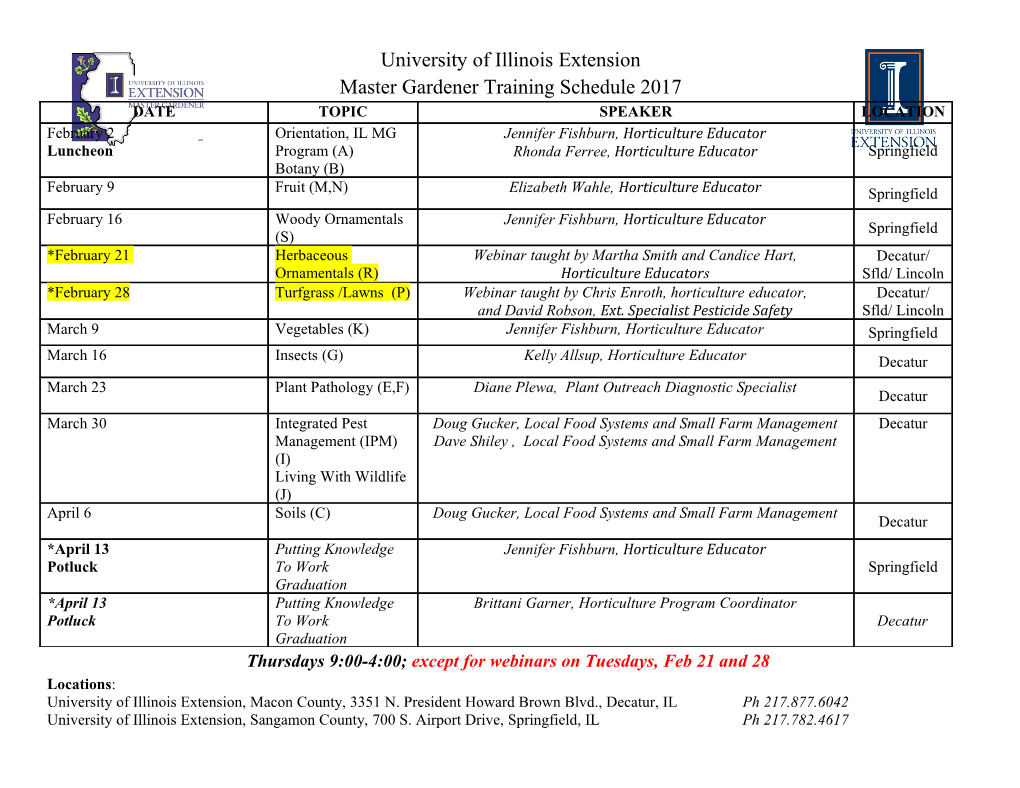
Vesicular Glycolysis Provides On-Board Energy for Fast Axonal Transport Diana Zala,1,2,3 Maria-Victoria Hinckelmann,1,2,3 Hua Yu,1,2,3 Marcel Menezes Lyra da Cunha,1,4 Ge´ raldine Liot,1,2,3,6 Fabrice P. Cordelie` res,1,5 Sergio Marco,1,4 and Fre´ de´ ric Saudou1,2,3,* 1Institut Curie, 91405 Orsay, France 2CNRS UMR3306, 91405 Orsay, France 3INSERM U1005, 91405 Orsay, France 4INSERM U759, 91405 Orsay, France 5IBiSA Cell and Tissue Imaging Facility, Institut Curie, 91405 Orsay, France 6Present address: Institut Curie, CNRS UMR 3347, INSERM U1021, Universite´ Paris-Sud 11, 91405 Orsay, France *Correspondence: [email protected] http://dx.doi.org/10.1016/j.cell.2012.12.029 SUMMARY and Vale, 2009), nerve cells must have energy sources distrib- uted throughout their axons for these molecular motors to Fast axonal transport (FAT) requires consistent function. energy over long distances to fuel the molecular Glucose is the major source of brain energy and is required for motors that transport vesicles. We demonstrate normal brain function. Glucose breakdown occurs in the cytosol that glycolysis provides ATP for the FAT of vesicles. through the glycolytic pathway that converts glucose into pyru- Although inhibiting ATP production from mitochon- vate and generates free energy (Ames, 2000). The net energetic dria did not affect vesicles motility, pharmacological balance of glycolysis is relatively low, with two ATP being produced from one molecule of glucose. In contrast to glycol- or genetic inhibition of the glycolytic enzyme GAPDH ysis, mitochondria produce 34 ATP molecules (Ames, 2000) reduced transport in cultured neurons and in and provide most of the energy used by the brain. However, Drosophila larvae. GAPDH localizes on vesicles via mitochondria are not evenly distributed within neurons, and are a huntingtin-dependent mechanism and is trans- most abundant at sites with a high-energy demand such as ported on fast-moving vesicles within axons. Purified synapses and nodes of Ranvier (MacAskill and Kittler, 2010). motile vesicles showed GAPDH enzymatic activity One fundamental requirement for efficient FAT is a constant and produced ATP. Finally, we show that vesicular supply of ATP. How is ATP distributed within neurons to fuel GAPDH is necessary and sufficient to provide on- the molecular motors? It also remains to be understood how board energy for fast vesicular transport. Although ATP can be continuously provided to fast-moving vesicles in detaching GAPDH from vesicles reduced transport, axonal segments devoid of mitochondria. These questions targeting GAPDH to vesicles was sufficient to pro- are of particular importance in the context of several neurode- generative disorders, such as amyotrophic lateral sclerosis, mote FAT in GAPDH deficient neurons. This specifi- Alzheimer’s, Parkinson’s, and Huntington’s diseases, which cally localized glycolytic machinery may supply are characterized by mitochondrial dysfunction and axonal constant energy, independent of mitochondria, transport defects (Hirokawa et al., 2010; Salinas et al., 2008). for the processive movement of vesicles over long Here we investigated the relative contributions of glycolysis distances in axons. and the mitochondrial respiratory chain in supplying ATP for FAT. INTRODUCTION RESULTS Fast axonal transport (FAT) is mediated by the molecular motors Mitochondria, GAPDH, and Axonal ATP dynein and kinesin that transport cargos over long distances in To reliably analyze vesicular FAT, we designed microfluidic- neurons (Hirokawa et al., 2010). This extremely rapid and effi- based devices derived from a previously described microfluidic cient transport is essential to deliver molecules such as trophic culture platform (Taylor et al., 2005). Embryonic rat cortical factors to nerve terminals that can be distant up to 1 m from neurons can extend long processes into the channels, but only cell bodies in the case of human motor neurons (Salinas et al., axons can reach the distal chamber located 450 mm away from 2008). FAT consumes energy because the dynein and kinesin the proximal chamber containing the cell bodies and most motors require ATP to transport cargos along microtubules. of the dendrites, as shown by Tau staining (Figure S1A Because kinesin hydrolyses one ATP per 8 nm step (Gennerich available online). We electroporated cortical neurons with Cell 152, 479–491, January 31, 2013 ª2013 Elsevier Inc. 479 ABFigure 1. Microfluidic Device to Analyze Transport and ATP Content in Axons (A) Transport of BDNF-mCherry in rat cortical axons. The top picture is a time projection of BDNF that reveals the presence of several axons in the microchannel. Top middle corresponds to the generated kymograph. Analyzed trajectories are shown with a color code, green for anterograde, red for retrograde, and blue for static vesicles. Bottom illustrates the distribution of velocity from ten analyzed kymographs (anterograde n = 174, retrograde n = 154). (B) Rat cortical neurons expressing Mito-DR and GAPDH-eGFP. Top is an overlaid picture taken in the cell body chamber compartment and middle shows the distal part of the channel with two axons (differential interference contrast [DIC] in gray, Mito-DR in red, and GAPDH-eGFP in green). Bottom is a two-color kymograph. (C) Rat cortical neurons expressing Perceval. pH C reporter SNARF 5 was added to the medium and chambers were analyzed with confocal micros- copy. Upper (1) DIC, reveals axons in the channel. SNARF5 labeling reveals two axons that were analyzed for their intracellular pH (2) and normal- ized to the isobastic point (iso, 3). The upper axon in the chamber shows high expression of Perceval that was used to analyze ADP (4) and ATP (5). The ADP, ATP overlay is shown in (o, 6). (D) Perceval signal reveals small fluctuations of the ATP:ADP ratio within axons whereas the pH ratio remained constant. D See also Figure S1. and Sheetz, 2004; Tristan et al., 2011), mitochondria were abundant in cell bodies and discontinuously distributed in neurites, whereas GAPDH was diffused throughout the cytoplasm (Figure 1B). The same pattern of distribution was fluorescent-tagged cargos and plated the neurons in micro- observed in axons extending within the microchannels (Fig- chambers. We performed FAT assays 3–5 days after the cells ure 1B). Mitochondria exhibited characteristic dynamic behavior were plated, when axons had reached the distal chamber. We within the microchambers, and were either stationary, very used brain-derived neurotrophic factor tagged with mCherry dynamic, or changing directions (Movie S2) as illustrated by (BDNF-mCherry), which displays the characteristic features of the two-color kymograph (Figure 1B). We also investigated the a FAT-dependent cargo and retains BDNF function (Kwinter trafficking of BDNF-mCherry-containing vesicles and of mito- et al., 2009). To selectively analyze axonal transport, we re- chondria in the same axons and found no overlap between corded vesicular dynamics in the distal part of the microchannels stationary or dynamic populations (Figure S1B). In contrast to and generated kymographs from the video recordings. Analyzes mitochondria, GAPDH was diffuse throughout the cytosol of of BDNF vesicle dynamics revealed fast and highly processive the chambered axons and its distribution did not appear to anterograde and retrograde movements (Figure 1A; Movie S1) change over time (Figure 1B). at velocities similar to those previously reported (Kwinter et al., Although there are many reliable methods to measure ATP 2009). content within cells, the subcellular location of ATP is more diffi- To analyze the distribution of mitochondria and of the key cult to resolve. To selectively determine changes in ATP content glycolytic enzyme glyceraldehyde-3-phosphate dehydrogenase within axons, we took advantage of a genetically encoded fluo- (GAPDH) in the microfluidic devices, we used a mitochondrial rescent sensor that monitors ATP:ADP ratio in living cells (Berg signal peptide fused to dsRed (Mito-DR) and GAPDH tagged et al., 2009). The ATP:ADP ratio was constant over long with GFP (GADPH-GFP) and verified that the respective behav- distances within the axons (Figures 1C and 1D). As the signal iors of these constructs in axons grown in microchannels were emitted by Perceval is pH-dependent, we measured possible similar to that in cultured neurons. As previously reported (Miller variations in intracellular pH using the SNARF 5 probe and 480 Cell 152, 479–491, January 31, 2013 ª2013 Elsevier Inc. observed no major fluctuations throughout the axons. This transport of vesicles may not strictly depend on mitochondria. suggests that there is a constant availability in ATP within axons Previous work showed that milton-C expression in neurons that is provided by both mitochondria and glycolysis. redistributes mitochondria into the cell bodies but does not alter mitochondria function (Glater et al., 2006). We therefore GAPDH but Not Mitochondria Is Critical for FAT expressed milton-C in cortical neurons and observed that mito- We next determined whether mitochondrial H+-ATP-synthase chondria clustered within cell bodies so that axons were inhibition by oligomycin, which blocks ATP production from the depleted of mitochondria (Figure 3A). By transfecting Perceval mitochondria (Chang and Reynolds, 2006), or whether GAPDH into neurons, we observed a progressive but marked reduction inhibition by iodoacetate affected FAT in cultured cortical of ATP:ADP ratio within axons of neurons coexpressing milton- neurons. We first measured the efficacy of oligomycin and C whereas the ratio in the cell body remained high (Figure 3B). iodoacetate in modulating ATP content within neurons. We We next coexpressed milton-C, GFP, and mito-DR in neurons added 1 mM pyruvate to the iodoacetate-treated neurons to and plated them in microchambers. Having verified that mito- bypass glycolysis and to allow mitochondria to continue to chondria were also absent from axons in microchannels as produce ATP. A 5 min treatment with oligomycin (20 mM) induced a result of milton-C expression (Figure 3C), we assessed BDNF a 25% reduction in ATP that reached to 80% after 30 min (Fig- vesicle dynamics in neurons expressing milton-C and BDNF- ure 2A).
Details
-
File Typepdf
-
Upload Time-
-
Content LanguagesEnglish
-
Upload UserAnonymous/Not logged-in
-
File Pages13 Page
-
File Size-