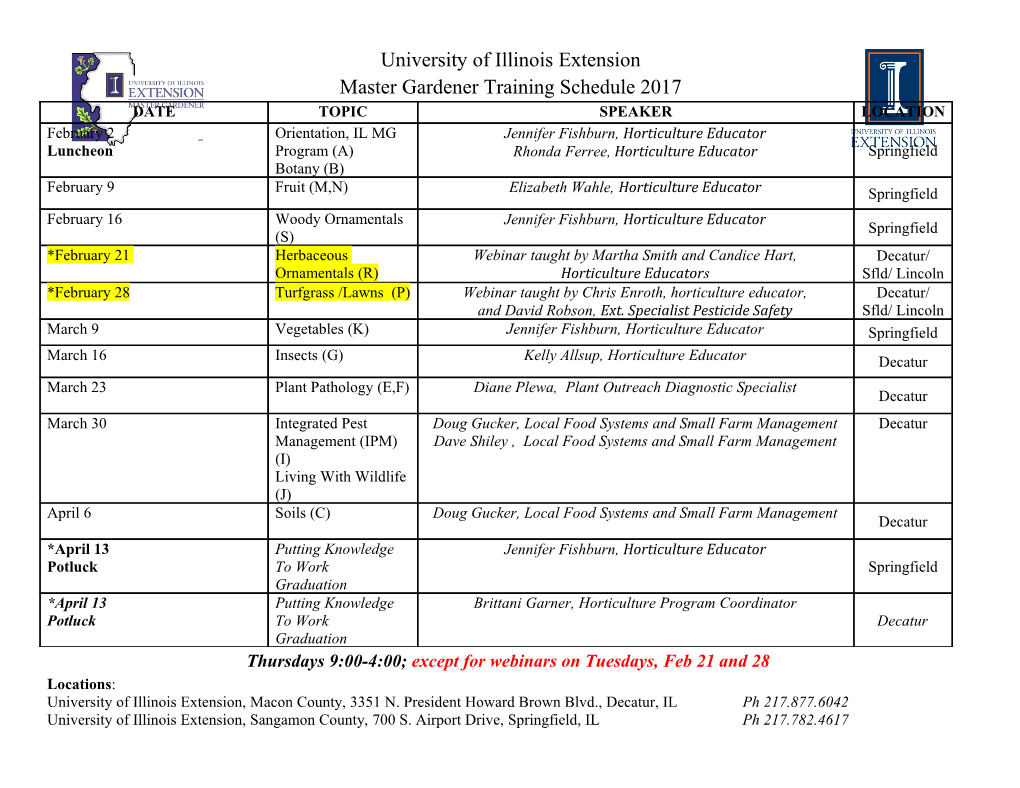
Cells and Materials Volume 5 Number 1 Article 9 1995 Adsorption of Proteins at Solid-Liquid Interfaces Willem Norde Wageningen Agricultural University, The Netherlands Follow this and additional works at: https://digitalcommons.usu.edu/cellsandmaterials Part of the Biomedical Engineering and Bioengineering Commons Recommended Citation Norde, Willem (1995) "Adsorption of Proteins at Solid-Liquid Interfaces," Cells and Materials: Vol. 5 : No. 1 , Article 9. Available at: https://digitalcommons.usu.edu/cellsandmaterials/vol5/iss1/9 This Article is brought to you for free and open access by the Western Dairy Center at DigitalCommons@USU. It has been accepted for inclusion in Cells and Materials by an authorized administrator of DigitalCommons@USU. For more information, please contact [email protected]. Cells and Materials, Vol. 5, No. 1, 1995 (Pages 97-112) 1051-6794/95$5.00+ .25 Scanning Microscopy International , Chicago (AMF O'Hare), IL 60666 USA ADSORPTION OF PROTEINS AT SOLID-LIQillD INTERFACES Willem Norde Department of Physical and Colloid Chemi stry, Wageningen Agri cultural Universi ty, P.O. Box 8038, 6700 EK Wageningen, The Netherlands Telephone number: (31 ) 08370-83540 I FAX number: (31 ) 08370-83777 (Received for publicati on October 4, 1994 and in revised form March 31, 1995) Abstract Introduction This paper concisely reviews the general principles Interacti on between proteins and solid surfaces is underl ying protein adsorption from aqueous solution commonly observed, both in natural and syntheti c sys­ onto a solid surface. The discussion includes the various tems. These interactions are of great relevance in , e.g., stages of the adsorption process, i. e., transport of the medical, bi otechnological and envi ronmental applica­ protein molecules towards the surface, the absorbed tions. In many cases, spontaneous adsorption of pro­ amount under equilibrium conditions, desorption andre­ teins leads to undesired consequences such as th rombus adsorption. Among the interacti ons that determine the formati on on syntheti c cardiovascul ar implants [76, 77 , overall protein adsorption process (1 ) redi stribution of 81, 82], fo uling of hemodi alysis membranes, contact charged groups in the interfacial layer, (2) changes in lenses and bi oprocessing equipment [29] , and plaque the hydrati on of the sorbent and the pro tein surface, and formati on on teeth and dental restorati ves [64, 80] . In (3) structural rearrangements in the protein molec ul e oth er cases, protein adsorpti on is made use of, for in­ play maj or roles. Special att ention is given to the re­ stance in drug deli very and controlled drug release sys­ lati on between the structu ral stabi lity of th e protei n tems [ 4], in di agnosti c tests (immunolatices) [39] , in bio­ molecul e and its adsorption behaviour. sensors [66] and in protein purification tech.niques [1 3]. In all these examples, the influence of the proximity of the sorbent surface on the bi ological fun ctioning and, because of the structure-function relati onship in proteins, on the three-dimensional structure of the pro tein mole­ cul e is of crucial importance. Effecti ve cont rol of the adsorption process requires an understanding of the un­ derl ying mechani sm, i.e., of the interacti ons that are in vo lved. Protein adsorption is an intricate process. Figure 1 shows a schemati c outline of the vari ous steps that are involved: transports toward , and binding at the surface, ori entati on and structure of the adsorbed molecules, reversibility of the sorption process. In this paper, each of the aspects depicted in Figure I will be addressed, emphasizing the relati on between protein structure stability and its adsorption behaviour. Adsorption Kinetics Key Words: Protein adsorption, solid-liquid interface, adsorption kineti cs, thermodynami cs of adsorption, elec­ The rate of transport of a protein molecule from trostatic interaction, hydrophobic interac ti on, rearrange­ soluti on towards an interface increases with increasing ment in protein structure, protein desorption, a-lactalbu­ concentrati on cp of the protein in solution. The min, lysozyme. "reacti on" of the protein with the interface, i.e., the actual att achment at the sorbent surface, is independent of cp. It is, therefore, to be expected that at low cp and low degree of coverage of the sorbent by the pro tein , th e 97 W. Norde Psol -- P~~s ~~~~~~~~-/~-. :--~-~-~~. {-/~ Figure 1. Schematic representation of the protein adsorption process. The native protein is denoted by P (the subscript sol refers to solution and ads to adsorbed state) and the structurally perturbed protein by P* and P**. The following steps are depicted: (1). Transport of P from solution towards the surface. (2). Attachment at the surface (re­ orientation?). (3). Structural rearrangement in the adsorbed molecule. (4). Detachment from the surface. (5). Transport away from the surface. transport process controls the rate of adsorption and that only and distinguish between (a) a solution tangentially at high cp and high degree of coverage, surface reactions flowing along a surface and (b) an impinging jet flow are rate-determining. that hits the surface perpendicularly in a so-called The basic transport mechanisms are diffusion and "stagnation point". convective transport by laminar or turbulent flow. For the tangential flow, the protein molecules are Under quiescent conditions, protein molecules reach the transported towards the sorbent surface by simultaneous sorbent surface by stochastic Brownian motion [59]. If convection and diffusion and, under conditions of a they are relatively rapidly attached at the surface, it steady-state concentration boundary layer in the solution leads to depletion of protein in the layer adjacent to the adjacent to the sorbent surface, the flux is given by the surface. The resulting concentration gradient causes Leveque equation [ 43] protein diffusion from the bulk solution towards the sorbent surface. Under such conditions, the rate of J = 0.54 (y/yD) 113 D cp (2) arrival of the protein at the sorbent surface is given by the Ward and Tordai equation [79] where y is the shear rate at the sorbent surface and y is the distance between the point of observation and the J = c (Dhr)l /2 rl/2 p (I) point where the protein solution has reached the surface at t = 0. where J is the flux of the protein per unit area of sorbent For a stagnation point flow, the flux of protein is surface; t, the time; D, the diffusion coefficient of the described by an equation derived by Dabros and Van de protein in solution; and 1r is 3.14 . Experimental data Yen [19] confirm diffusion-controlled adsorption from non-flow­ ing solutions of low concentration [ 10, 22, 24, 44]. In practice, however, proteins mostly adsorb from flowing solutions. Here, we will consider laminar fl ow where v is the kinematic viscosity of the solution,¢ the 98 Protein adsorption 1.5 1.5 Si02 I PS N I N I E E 01 0'1 E LSZ E a. LA L L LSZ a. LA 200 100 200 time/s time /s Figure 2. Adsorption of lysozyme and a-lactalbumin fro m an impinging jet fl ow (cp = 1 g dm-3) on hydrophilic silica (left) and on hydrophobic polystyrene-coated silica (rig ht). Adsorbed amount as a function of time, as determined by refl ectometry. 0.01 M phosphate buffer pH 7.0; T = 25°C. Dashed line represents the flu x of the pro tei n molecules arriving at the surface, according to equati on (3). volume flux and R the radius of the nozzle of the device By way of example, the adsorption rates for two that supplies the solution to the sorbent surface. Equa­ well-characteri zed proteins, i.e., hen's egg lysozyme tion (3) is only valid under the conditions th at (a) the (LSZ) and bovine milk a-lactalbumin (aLA) at both a nozzle of the supplier is cylindricall y shaped, (b) the dis­ hydro ph ili c and a hydrophobic stagnati on point , are tance between the nozzle and the surface is much larger shown in Figure 2, together with the theoretical flu xes than R, (c) the particles in the solution are spheri cal and towards th e surface . Some relevant characteri sti cs of (d) interacti ons between the particles in solution are LSZ and a LA, as well as of the sorbent surfaces, are absent. summari zed in Table I . Note that the proteins are si mi­ Initial adsorption rates (i. e., at conditions of low lar as to their molecul ar dimensions and masses, but dif­ surface coverage, so that, in principle, each arriving fer markedly with respect to their isoelectric points and protein molecule can be accommodated at the surface) structural stabilities. Hence, comparative studies with may be compared with the flu xes calculated usin g the these systems may help to understand the role of hydro­ appropriate equation (1 ), (2) or (3). Adsorption rates phobic dehydrati on, electrostatic interacti on and protein have been measured by various authors [3 , 15 , 27, 35, structure stability in the behav iour of proteins at 56, 75] applying a wide spectrum of ex perimental tech­ interfaces. niques. An extensive review has been gtven by With both LSZ and aLA, th e initial adsorption rate Ramsden [65]. is smaller than the flu x. In general , the initial adsorption rates show the On the hydrophilic silica surface, the adsorbing frac­ same dependence on the experimental variables as ex ­ ti on of a LA is much smaller than that of LSZ. The dif­ pressed in the corresponding equati ons for J. However, ference refl ects the diffe rence in electrostati c interacti on in many cases the adsorption rate is considerably smaller between th e proteins and the sorbent , i.e., attracti on of than the flux towards the surface [ 11 , 22, 31 , 58].
Details
-
File Typepdf
-
Upload Time-
-
Content LanguagesEnglish
-
Upload UserAnonymous/Not logged-in
-
File Pages17 Page
-
File Size-