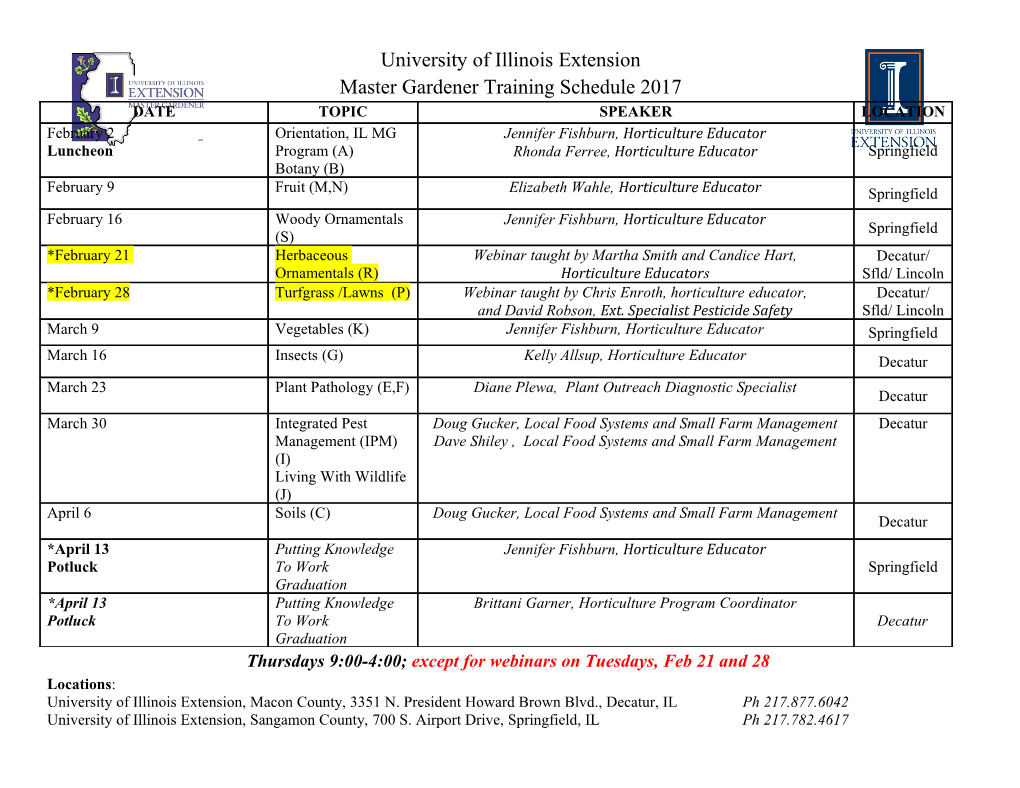
bs_bs_banner Minireview Genetic characterization of caffeine degradation by bacteria and its potential applications Ryan M. Summers,1† Sujit K. Mohanty,2† Sridhar production of chemical and fuels and development of Gopishetty3 and Mani Subramanian2,3* diagnostic tests have also been demonstrated. 1Department of Chemical and Biological Engineering, The University of Alabama, Tuscaloosa, AL 35487, Introduction USA. 2Department of Chemical and Biochemical Engineering Caffeine (1,3,7-trimethylxanthine) and related methylxan- and 3Center for Biocatalysis and Bioprocessing, The thines are natural purine alkaloids found in many plants University of Iowa, Coralville, IA 52241, USA. around the world (Ashihara and Crozier, 1999). These compounds are hypothesized to serve as natural insecti- cides, and have been shown to protect the plants Summary from insects and other predators (Nathanson, 1984; The ability of bacteria to grow on caffeine as sole Hollingsworth et al., 2002). Other possible reasons for carbon and nitrogen source has been known for over biosynthesis of caffeine include inhibition of plant matter 40 years. Extensive research into this subject has (Waller, 1989) and improved pollination (Wright et al., revealed two distinct pathways, N-demethylation and 2013). C-8 oxidation, for bacterial caffeine degradation. Methylxanthines are often consumed by humans in However, the enzymological and genetic basis for foods and beverages, including chocolate, coffee and tea. bacterial caffeine degradation has only recently been Coffee is a major worldwide agricultural commodity, with discovered. This review article discusses the recent millions of metric tons produced and distributed globally discoveries of the genes responsible for both each year (Summers et al., 2014). In addition to the N-demethylation and C-8 oxidation. All of the genes food industry, caffeine and related methylxanthines are for the N-demethylation pathway, encoding enzymes also used in pharmaceuticals as stimulants, diuretics, in the Rieske oxygenase family, reside on 13.2-kb bronchodilators, vasodilators and in the treatment and/or genomic DNA fragment found in Pseudomonas prevention of axial myopia, glaucoma and macular degen- putida CBB5. A nearly identical DNA fragment, with eration (Stavric, 1988a,b,c; Trier et al., 1999; Dash and homologous genes in similar orientation, is found in Gummadi, 2006b; Daly, 2007). Pseudomonas sp. CES. Similarly, genes for C-8 oxi- Although bacterial caffeine degradation has been dation of caffeine have been located on a 25.2-kb studied since the 1970s, very little was known concerning genomic DNA fragment of Pseudomonas sp. CBB1. the enzymes and genes responsible for caffeine degra- The C-8 oxidation genes encode enzymes similar to dation until recently. Several excellent reviews have those found in the uric acid metabolic pathway summarized the bacterial caffeine catabolic pathways of Klebsiella pneumoniae. Various biotechnological (Mazzafera, 2004; Dash and Gummadi, 2006a,b; applications of these genes responsible for bacterial Gummadi et al., 2012), including recent developments caffeine degradation, including bio-decaffeination, (Gopishetty et al., 2012). In this review, we focus on the remediation of caffeine-contaminated environments, discoveries made since the publication of these reviews, with specific emphasis on the genes responsible for caf- feine degradation in bacteria. Received 22 September, 2014; revised 10 December, 2014; accepted 26 December, 2014. *For correspondence. E-mail mani-subramanian Caffeine metabolism @uiowa.edu; Tel. 319 335 4705; Fax 319 335 4901. doi:10.1111/1751-7915.12262 To date, over 35 bacterial strains that are capable of †Authors contributed equally to this review. Funding Information This research was supported by University of degrading caffeine have been isolated and reported Iowa Research Funds. (Table 1). While there is some diversity among the types © 2015 The Author. Microbial Biotechnology published by John Wiley & Sons Ltd and Society for Applied Microbiology. This is an open access article under the terms of the Creative Commons Attribution License, which permits use, distribution and reproduction in any medium, provided the original work is properly cited. 2 R. M. Summers, S. K. Mohanty, S. Gopishetty and M. Subramanian Table 1. Characterized bacterial strains capable of degrading caffeine. Organism Location isolated Catabolic pathway References Pseudomonas putida strain 40 California, USA N-demethylation Woolfolk, 1975 Pseudomonas putida C1 Germany N-demethylation Blecher and Lingens, 1977 Pseudomonas putida C3024 Netherlands N.R. Middelhoven and Bakker, 1982 Pseudomonas putida WS Germany N-demethylation Glück and Lingens, 1987 Pseudomonas sp. No. 6 Japan N-demethylation Asano et al., 1993 Pseudomonas putida No. 352 Japan N-demethylation Asano et al., 1994 Serratia marcescens Brazil N-demethylation Mazzafera et al., 1996 Pseudomonas putida ATCC 700097 California, USA N-demethylation Ogunseitan, 1996 Klebsiella and Rhodococcus India C-8 oxidation Madyastha and Sridhar, 1998 Pseudomonas putida (8 strains) Brazil N.R. Yamaoka-Yano and Mazzafera, 1998 Pseudomonas fluorescens Brazil N.R. Yamaoka-Yano and Mazzafera, 1998 Coryneform (4 strains) Brazil N.R. Yamaoka-Yano and Mazzafera, 1998 Acinetobacter sp. (3 strains) Brazil N.R. Yamaoka-Yano and Mazzafera, 1998 Flavobacterium sp. (2 strains) Brazil N.R. Yamaoka-Yano and Mazzafera, 1998 Moraxella sp. Brazil N.R. Yamaoka-Yano and Mazzafera, 1998 Pseudomonas putida IF-3 Japan N-demethylation Koide et al., 1996 Pseudomonas putida L Brazil N-demethylation Yamaoka-Yano and Mazzafera, 1999 Pseudomonas putida KD6 N.R. N-demethylation Sideso et al., 2001 Alcaligenes sp. Canada C-8 oxidation Mohapatra et al., 2006 Pseudomonas putida NCIM 5235 India N-demethylation Dash and Gummadi, 2006a Pseudomonas sp. CBB1 Iowa, USA C8-oxidation Yu et al., 2008 Pseudomonas alcaligenes CFR 1708 India N.R. Sarath Babu et al., 2005 Alcaligenes fecalis T1 India N.R. Sarath Babu et al., 2005 Acetobacter sp. T3 India N.R. Sarath Babu et al., 2005 Pseudomonas putida CBB5 Iowa, USA N-demethylation Yu et al., 2009 Pseudomonas sp. CES Iowa, USA N-demethylation Yu et al., 2014 N.R., not reported. of bacteria isolated, the majority are Pseudomonas, pri- xanthine. Finally, xanthine is converted to uric acid, marily Pseudomonas putida. Caffeine-degrading bacteria which enters normal purine catabolic pathway. are geographically dispersed, and have been found in Theophylline (1,3-dimethylxanthine) has not been coffee fields (Yamaoka-Yano and Mazzafera, 1998), reported as a metabolite of caffeine in bacteria. However, wastewater streams (Ogunseitan, 1996) and garden it is the first major metabolite of caffeine in fungi (Hakil soil (Blecher and Lingens, 1977; Yu et al., 2008; 2009; et al., 1998), and is further degraded via N-demethylation 2014). to 3-methylxanthine and xanthine. Although the bacterium Metabolic studies with these caffeine-degrading bacte- P. putida CBB5 does not produce theophylline from caf- rial isolates have revealed only two catabolic pathways: feine, it has been reported to degrade theophylline by N-demethylation and C-8 oxidation. The N-demethylation N-demethylation (Yu et al., 2009). Both 3-methylxanthine pathway appears to be the most common, as it has been (major product) and 1-methylxanthine (minor product) are observed in over 80% of reported isolates where metabo- formed from theophylline in CBB5 and are further lism has been characterized. In both pathways, bacteria N-demethylated to form xanthine, as in the caffeine cata- break caffeine down to carbon dioxide and ammonia to bolic pathway. harvest energy and cellular building blocks. Some of the metabolites formed during the bacterial During N-demethylation, the caffeine molecule is N-demethylation of caffeine also undergo C-8 oxidation to sequentially N-demethylated to form xanthine (Fig. 1A). form their corresponding uric acids (Blecher and Lingens, Each of the three methyl groups is removed with incor- 1977; Yamaoka-Yano and Mazzafera, 1999). In most poration of molecular oxygen to produce one formalde- strains, this involves the formation of 3,7-dimethyluric hyde and one water molecule per reaction. Theobromine acid, 1,7-dimethyluric acid and 7-methyluric acid from (3,7-dimethylxanthine) is the major metabolite formed theobromine, paraxanthine and 7-methylxanthine respec- from the first step in the pathway, with small amounts of tively. These methyluric acids are not formed during caf- paraxanthine (1,7-demethylxanthine) also reported in feine N-demethylation in P. putida CBB5. However, CBB5 some strains (Yamaoka-Yano and Mazzafera, 1999; Yu converts approximately 25% of the entire theophylline et al., 2009; 2014). The second step of the pathway metabolite pool to 1,3-dimethyluric acid, 1-methyluric is the N3-demethylation of theobromine or the N1- acid and 3-methyluric acid. There is no evidence that demethylation of paraxanthine to form 7-methylxanthine. these methyluric acids are further metabolized (Yu et al., 7-Methylxathine is further N7-demethylated to form 2009). © 2015 The Author. Microbial Biotechnology published by John Wiley & Sons Ltd Genetics of bacterial caffeine degradation 3 Fig. 1. Proposed caffeine N-demethylation pathway (A) and map of associated genes (B) in Pseudomonas putida CBB5. The dashed arrows in part (A) represent a minor pathway, accounting for 1–2% of metabolized caffeine. NdmA/ndmA = N1-demethylase specific for N1-methyl group of caffeine; NdmD/ndmD = reductase;
Details
-
File Typepdf
-
Upload Time-
-
Content LanguagesEnglish
-
Upload UserAnonymous/Not logged-in
-
File Pages10 Page
-
File Size-