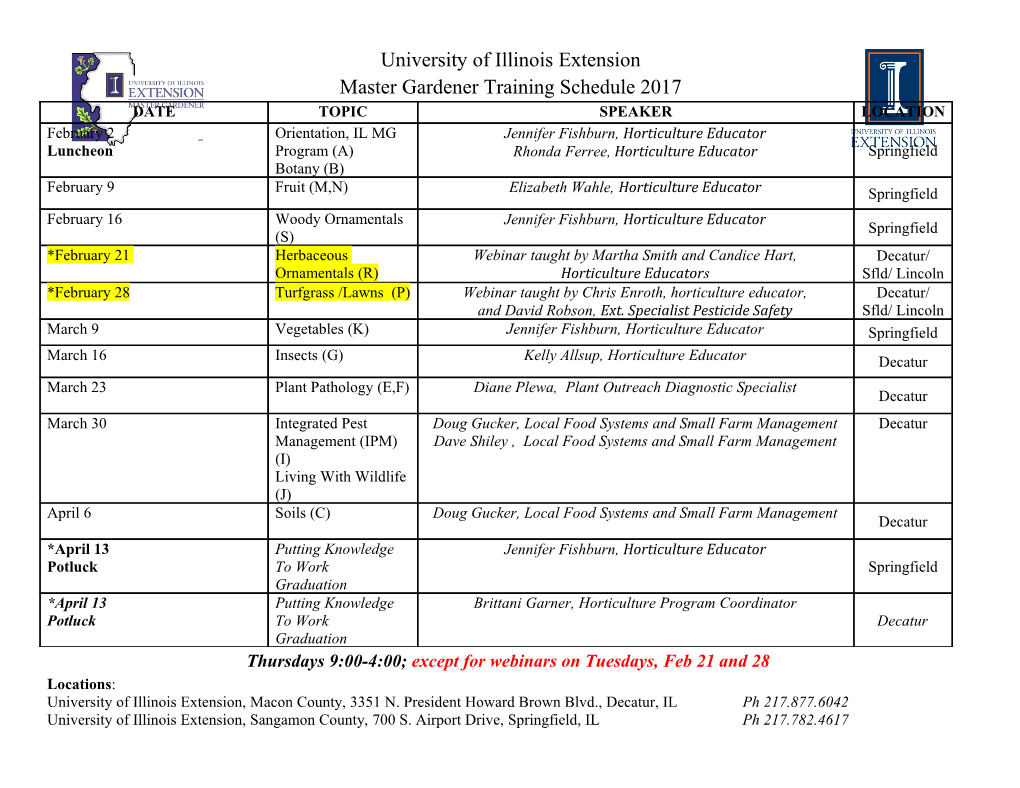
www.nature.com/scientificreports OPEN An autoimmune disease risk SNP, rs2281808, in SIRPG is associated with reduced expression of SIRPγ Received: 29 June 2018 Accepted: 8 October 2018 and heightened efector state in Published: xx xx xxxx human CD8 T-cells Sushmita Sinha1, Nicholas Borcherding1, Pranav S. Renavikar1, Michael P. Crawford1, Eva Tsalikian2, Michael Tansey2, Ezzatollah T. Shivapour3, Frank Bittner3, John Kamholz3, Heena Olalde3, Emilee Gibson3 & Nitin J. Karandikar1 Multiple GWAS studies have shown that the SNP rs2281808 TT variant, present within the SIRPG gene, is associated with autoimmune diseases, such as type 1 diabetes. However, the role of SIRPγ in human T-cells is not known, neither is the functional signifcance of TT variant. Here we investigated SIRPG genotypes and their efects on the fate and function of human T-cells. We found that the presence of T variant resulted in reduction of SIRPγ expression on T-cells. Functionally, SIRPγlow CD8 T-cells in CT and TT individuals existed in a heightened efector state with lower activation threshold and had greater expression of genes and molecules associated with migratory and cytotoxic potential. Further, SIRPγlow CD8 T-cells were defcient in transcription factors associated with long-term functional memory formation. Our study reveals biological consequences of the SNP rs2281808 and provides novel insights into the potential mechanisms by which SIRPγ might regulate human immune responses. Genome-wide association studies have been instrumental in identifying genetic risk variants in autoimmune diseases. However, in most cases, the biological interpretation of how the reported risk variants potentiate auto- immunity remains unknown. Multiple GWAS studies have shown that the SNP rs2281808 TT variant is associ- ated with type 1 diabetes (T1D)1–3. Rs2281808 TT is an intronic SNP present between exons 5 and 6 of the Signal Regulatory Protein (SIRPG) gene and causes a C/T variant. Te rs2281808 T risk allele confers a 1.1 (1.01–1.19) increased risk of developing T1D (per dbSNP; https://www.ncbi.nlm.nih.gov/projects/SNP/) and might be asso- ciated with early onset diabetes patients3. Te biological consequence of rs2281808 T variant and the mechanisms by which it potentiates autoimmunity remains unknown. SIRPγ is absent in the rodents. In humans, SIRPγ is the only SIRP expressed by T-cells4 and its function is poorly understood. Engagement of SIRPγ on T cells by CD47 on APCs has been shown to enhance antigen- specifc T-cell proliferation5. SIRPγ may also be required for trans-endothelial migration of human T-cells in vitro6. Te most substantial hint for the mechanistic role of SIRPγ in autoimmunity comes from a recent study predicting that polymorphisms in SIRPG gene can interfere with transcription factors important in T-cell devel- opment7. Further, Diferential expression of SIRPG has also been reported in Systemic Lupus Erythematosus (SLE) patients, suggesting that SIRPγ might be pathologically relevant in multiple autoimmune diseases. Since polymorphism in SIRPG gene is associated with the development of T1D, we hypothesized that the rs2281808 genotype might modulate SIRPγ-mediated regulation of T-cell efector responses. We provide the frst evidence that rs2281808 T variant is associated with a reduction in SIRPγ expression on human T-cells and that this can have potentially pathogenic consequences since SIRPγlow CD8 T-cells were char- acterized by exaggerated efector responses. 1Department of Pathology, University of Iowa, 200 Hawkins Dr., Iowa City, IA, 52242, USA. 2Department of Pediatrics, University of Iowa, 200 Hawkins Dr., Iowa City, IA, 52242, USA. 3Department of Neurology, University of Iowa, 200 Hawkins Dr., Iowa City, IA, 52242, USA. Correspondence and requests for materials should be addressed to S.S. (email: [email protected]) SCIENTIFIC REPORTS | (2018) 8:15440 | DOI:10.1038/s41598-018-33901-1 1 www.nature.com/scientificreports/ Figure 1. Autoimmune disease risk SNP rs2281808 causes low of SIRPγ expression on human T-cells. All the 79 PBMC samples from HD were subjected to fow cytometry staining and genotyping for rs2281808 using TaqMan chemistry. SIRPγ expression relative to rs2281808 genotyping status was analyzed on gated CD3 CD4 and CD3 CD8 T-cells (A–E). Representative histograms (A,C) and cumulative MFI data (B,D) are shown. CD8 T-cells showed a bimodal expression of SIRPγ, which was used to determine the frequency of SIRPγhigh and SIRPγlow cells. Te frequency of SIRPγlow CD8 T-cells is shown in (E). Isotype staining is shown in grey. Gates are shown for SIRPγlow cells. One-way ANOVA with Tukey’s posthoc test was performed and p < 0.05 was considered signifcant. Results SNP rs2281808 TT is associated with the reduction of SIRPγ expression on T cells. To determine whether the rs2281808 TT variant regulates SIRPγ expression on T-cells, 79 healthy donors (HD) were geno- typed for SNP rs2281808 and assessed for SIRPγ expression. We found that 45 and 31 HD showed the CC and CT genotypes, respectively, whereas the TT variant was present in 3 HD. Flow cytometry revealed that the CC genotype was associated with robust SIRPγ expression on the majority of CD4 and CD8 T-cells. In contrast, CD4 (Fig. 1A,B) and CD8 (Fig. 1C,D) T-cells from rs2281808 TT carriers had signifcantly reduced surface expression of SIRPγ, whereas the CT genotype was associated with an intermediate SIRPγ expression that was signifcantly lower than CC cells (SIRPγ-MFI on CD4 T-cells in TT vs. CT vs CC: 203 ± 10.8 vs. 350 ± 123 vs 526 ± 244, CC vs. CT & CT vs. TT, p < 0.05; CC vs. TT, p < 0.01, p < 0.05 and SIRPγ-MFI on CD8 T-cells in TT vs. CT vs. CC: 160 ± 7.9 vs. 275 ± 93 vs. 439 ± 170; CC vs. CT & CT vs. TT, p < 0.05; CC vs. TT, p < 0.01). We also noted that, in contrast to the unimodal distribution of SIRPγ on CD4 T-cells, it showed a bimodal dis- tribution on CD8 T-cells, which was particularly pronounced in CT carriers (Fig. 1C), who showed signifcantly greater frequencies of SIRPγlow CD8 T-cells as compared to CC carriers (21.8% ± 12 vs. 37.4% ± 11, p < 0.05; Fig. 1E). In keeping with the MFI, the majority of CD8 T-cells (≥80%) in TT carriers were SIRPγlow (Fig. 1C,E). SCIENTIFIC REPORTS | (2018) 8:15440 | DOI:10.1038/s41598-018-33901-1 2 www.nature.com/scientificreports/ Figure 2. CT and TT carriers, but not CC individuals, have a signifcantly greater proportion of naïve CD8 T-cells with a SIRPγlow phenotype. Flow cytometry staining for CD27 and CD45RO was used to analyze SIRPγlow CD8 T-cells in the naïve (CD27+ CD45RO−), central memory (CD27+ CD45RO+), efector memory (CD27−CD45RO+) and terminally diferentiated CD27−CD45RO−) fractions. Detailed gating strategy is shown in Supplementary Fig. 1. In CC individuals, SIRPγlow CD8 T-cells were essentially absent from the naïve pool, whereas in CT and TT carriers, SIRPγlow CD8 T-cells were present in the naïve pool at increasing frequencies (A). Representative dot plots are shown in (B), where the blue population indicates SIRPγlow CD8 T-cells and the red population corresponds to the SIRPγhigh CD8 T-cells. One-way ANOVA with Tukey’s posthoc test was performed and p < 0.05 was considered signifcant. Unlike CT/TT carriers, SIRPγlow CD8 T-cells in CC carriers are absent from the naïve pool. We also noted that 6/42 (14%) of CC individuals in HD showed relatively higher frequencies of SIRPγlow CD8 T-cells compared to the rest of the CC donors. Similarly, there were 7/32 (22%) of CT individuals who showed a relatively low fraction of SIRPγlow CD8 T-cells (Fig. 1E, outliers). In this regard, the CC individuals exhibited a CT pattern of staining and vice versa. We hypothesized that the SIRPγlow cells from CC individuals may represent down- regulation of SIRPγ during efector/memory diferentiation (as opposed to having a SIRPγlow fraction in naïve CD8 T-cells). To test this, we evaluated the distribution of SIRPγ-high and low cells within the naïve vs. efector/ memory fractions. Te gating strategy is shown in Supplementary Fig. 1. Te overall distribution of CD8 T cell subsets based on their rs2281808 genotyping status is shown in Supplementary Fig. 2. Interestingly, in all CC carriers, the vast majority (94.5% ± 3.4) of SIRPγlow CD8 T-cells were present in memory/terminally-diferentiated fraction (Fig. 2A,B). In contrast, SIRPγlow CD8 T-cells from CT carriers were also present at greater proportions in the naïve T-cell fraction (27.5% ± 9.7; Fig. 2A,C). Te absence of SIRPγlow CD8 T-cells from the naïve pool of CC carriers suggests that thymically-derived CD8 T-cells of CC individuals express high levels of SIRPγ, which can then downregulate its expression during diferentiation. Importantly, C/T replacement even in one locus appears to alter this pattern in CT carriers, where higher frequencies of naïve cells are SIRPγlow, signifcantly more than CC subjects (Fig. 2A). As expected from the overall staining pattern, TT individuals carried SIRPγlow CD8 T-cells in all fractions. SIRPγlow CD8 T-cells from CT carriers display an efector cell profle with enhanced cytotoxic potential. We focused on studying CD8 T-cells since the bimodal distribution of SIRPγ on CD8 T-cells gave us the opportunity to study SIRPγhigh and SIRPγlow cells from the same HD in subsequent studies, in addition to comparing CC vs. TT CD8 T-cells. First, we performed RNA sequencing analysis on fow-sorted SIRPγ-high vs. low CD8 T-cells from the same individuals. Te SIRPγlow versus SIRPγhigh cells were equally distributed between naïve, central memory (CM), efector memory (EM) and terminally diferentiated (TD) fractions in the two individuals as shown in Fig.
Details
-
File Typepdf
-
Upload Time-
-
Content LanguagesEnglish
-
Upload UserAnonymous/Not logged-in
-
File Pages9 Page
-
File Size-