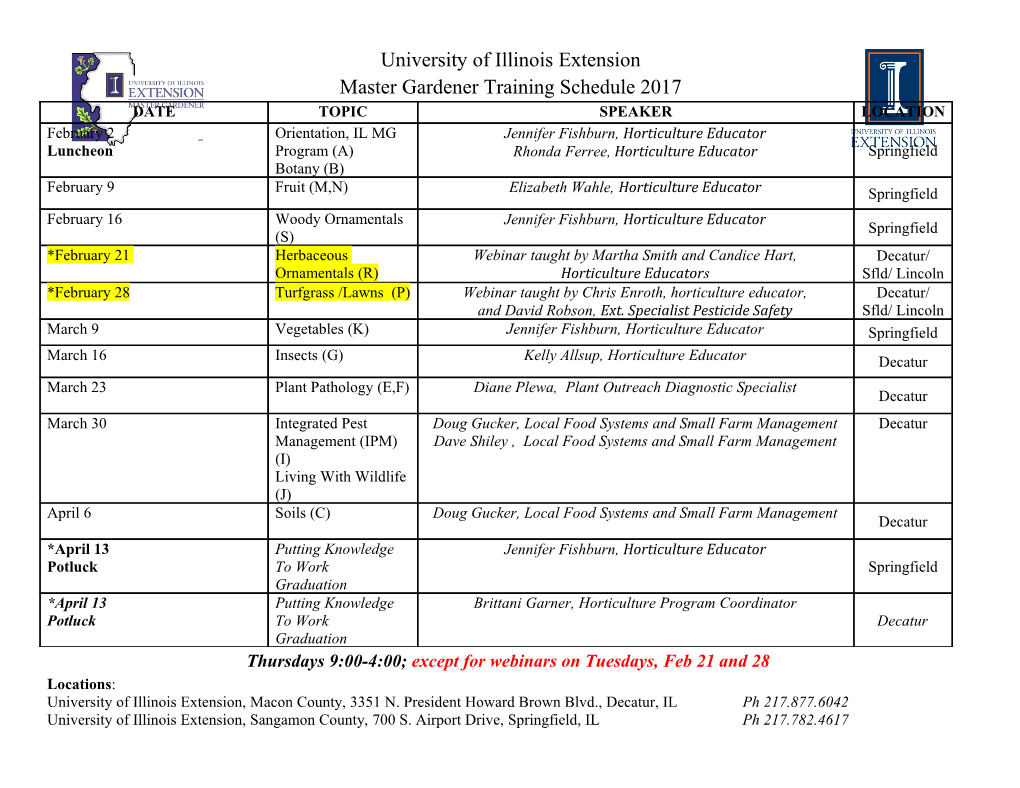
1Catalyst- and silane- controlled Enantioselective Hydrofunctionali- zation of Alkenes by TM-HAT and RPC mechanism Kousuke Ebisawa, Kana Izumi, Yuka Ooka, Hiroaki Kato, Sayori Kanazawa, Sayura Komatsu, Eriko Nishi, Kou Hiroya, and Hiroki Shigehisa* Faculty of Pharmacy, Musashino University, 1-1-20 Shinmachi Nishitokyo-shi, Tokyo 202-8585, Japan Supporting Information Placeholder ABSTRACT: The catalytic enantioselective synthesis of tetrahy- However, there is significant room for improvement in the sub- drofurans, found in the structures of many biologically active strate scope and yield. Lin also recently reported the enantioselec- natural products, via a transition-metal-catalyzed hydrogen atom tive hydrocyanation of conjugated alkenes by dual electrocatalysis transfer (TM-HAT) and radical-polar crossover (RPC) mechanism that include a HAT mechanism.8 is described. Hydroalkoxylation of non-conjugated alkenes pro- ceeded efficiently with excellent enantioselectivity (up to 97:3 er) using a suitable chiral cobalt catalyst, N-fluoro-2,4,6- trimethylpyridinium tetrafluoroborate, and a diethylsilane. Sur- prisingly, absolute configuration of the product was highly de- pendent on the bulkiness of the silane. Mechanistic studies sug- gested a HAT mechanism and multiple enantiodetermining steps via an organocobalt(III) intermediate. DFT calculations suggested the presence of a cationic organocobalt intermediate, and that a critical factor of the enantioselectivity is the thermodynamic sta- bility of the organocobalt(III) intermediate. Transition-metal-catalyzed hydrogen atom transfer (TM-HAT) to alkenes has recently attracted significant attention in synthetic organic chemistry.2 Since Mukaiyama and Isayama reported the Figure 1. Achiral and chiral hydrofunctionalization of alkenes via catalytic hydration of alkenes under aerobic conditions,3 the al- TM-HAT. kene-chemoselective reaction and its modifications have been recently used in the synthesis of complex molecules such as natu- Herein, we disclose the catalytic hydroalkoxylation of non- ral products.4 The hydration has now advanced to use in diverse conjugated alkenes via a TM-HAT and RPC mechanism with hydrofunctionalizations and other useful transformations (Figure excellent enantioselectivity (Figure 1B, right). This catalysis can 1A, left).4a, 5 be carried out without an electrochemical apparatus. This reaction affords chiral tetrahydrofurans, which are found in the structures Our group has also reported diverse hydrofunctionalizations us- of many biologically active natural products.9 Notably, the abso- ing a cobalt Schiff base catalyst, N-fluoropyridinium salt, and a 6 lute configuration of the product was highly dependent on the silane reagent. A remarkable and unique mechanistic feature of bulkiness of the silane reagent, which is considered simply a hy- this reaction is the radical species generated by cobalt hydride- drogen source for the cobalt hydride species. This observation is HAT was transformed by single-electron oxidation into a cationic unique in TM-HAT chemistry. We therefore investigated the species to receive various nucleophiles (Figure 1A, right). The mechanism of this catalysis and the critical factors for its enanti- addition of an N-fluoropyridinium salt to the TM-HAT system oselectivity through various experiments and density functional paved the way for the radical-polar crossover (RPC) mechanism, theory (DFT) calculations. and consequently, enabled the protonation of alkenes without a proton source, thus realizing excellent chemoselectivity and func- Our preliminary experiment using chiral cobalt catalyst C2 (see tional group tolerance. Table 1 for structure) resulted in only slight enantioselectivity (28% ee).6d For this work, we explored the enantioselective hy- Despite numerous reports, enantioselective hydrofunctionaliza- droalkoxylation of alkenyl alcohol 1a as a model compound tion of alkenes based on a TM-HAT reaction mechanism remain (>200 runs, >30 cobalt catalysts, and >20 silanes) and identified rare. Recently, Pronin reported the enantioselective synthesis of the optimal reaction conditions as the use of cobalt catalyst C3 an epoxide from a tertiary allylic alcohol by a TM-HAT and RPC (10 mol %),10 N-fluoro-2,4,6-trimethylpyridinium mechanism using a finely tuned cobalt complex (Figure 1B, left).7 1 Table 1. Screening of cobalt catalyst and silane (summarized)a Best conditions: 1a (0.1 mmol), C3 (10 mol %), 3 (2 equiv.), diethylsilane (2 equiv.), CH2Cl2 (4.0 mL), CF3CH2OH (0.2 mL), −10 °C, 20 min (The same result was obtained for 24 h.) aReaction times for experiments other than the best conditions were 24−96 h. b2 equiv. of silane. c4 equiv. of silane. tetrafluoroborate (3),11 and diethylsilane (S4) in degassed di- We found the sterically least hindered diethylsilane (S4) was op- chloromethane/trifluoroethanol (10:1)12 at -10 °C to afford cyclic timal. To our surprise, the use of the sterically more hindered ether (S)-2a13 in excellent yield (77%) and enantiomeric ratio diisopropylsilane (S5) afforded (R)-2a as the major enantiomer. (97:3) (Table 1). The more hindered di-tert-butylsilane (S6) resulted in no reaction Screening of various chiral cobalt complexes revealed that the of 1a. The stereochemistry of the major enantiomer formed using bulky ligands (C3 – C8) reported by Katsuki are preferable to the a tertiary silane S1 – S3 was also (R)-2a, which is the same as that simpler salen ligand (C1)14 or β-ketoiminate ligand (C2).15 Nota- when using diisopropylsilane (S5). Therefore, steric hindrance of bly, the axial chirality of the binaphthyl unit had a significant the silane determined the absolute configuration of the product. A impact on the enantioselectivity; even C8, which includes an achi- primary silane afforded a near racemate. ral diamine, provided higher enantioselectivity than C1 or C2. After establishing the optimal reaction conditions, we explored Whereas the introduction of two methyl groups on the diamine the scope of the enantioselective hydroalkoxylation with various (C7) did not improve the enantioselectivity, diphenyl (C5) or alkenyl alcohols (Table 2). We commenced examining the steric cyclohexyl (C3) diamine increased the selectivity, probably by effects by introducing a methyl group on the phenyl rings, and locking the conformation of the ligand. The conformation of the found no significant difference in the results among 4-methyl (2b), ligand is crucial for reactivity and enantioselectivity, as seen by 3-methyl (2c), 2-methyl (2d), 3,5-xylyl (2e), 1-naphthyl (2f), and the significantly worse results from using the diastereomers C4 2-naphthyl (2g). We next investigated the electronic effect of the and C6. aromatic ring. Whereas introduction of a methoxy group did not Extensive silane screening showed that a secondary silane was change the yield or enantioselectivity (2h), introduction of a halo- essential for obtaining (S)-2a with excellent enantioselectivity. gen substituent slightly decreased enantioselectivities (2i-2k). Examination of the functional group tolerance of this reaction 2 Table 2. Substrate scope of enantioselective hydroalkoxylation of alkenyl alcohol. Conditions: 1b - t (0.1 mmol), C3 (10 mol %), 3 (2 equiv.), diethylsilane (2 equiv.), CH2Cl2 (4.0 mL), CF3CH2OH (0.2 mL), −10 °C, 16 h. atemperature = −20 °C. revealed that product could be obtained from alkenyl alcohols 100 bearing an acid-sensitive acetal (2l), fluoro-anion-sensitive silyl ether (2m), or base-sensitive acetate (2n). Replacing the phenyl ring with heterocycles such as thiophene (2o) or N-tosyl indole 50 (%) (2p) resulted in comparable yields and enantioselectivities. Unfor- 2a - tunately, introducing a methylthio group (2q) or other subunits ) 0 S such as indane (2r), N-Ts-piperidine (2s), or bisphenethyl (2t) ( instead of the diaryl groups reduced the enantioselectivity. For- ee of -50 mation of a 6-membered ring (2u, low conversion), use of a di- substituted alkene (2v, low conversion), or a MOM-protected alkenyl alcohol (2b, 16%, er 51:49) were found to be ineffective -100 under our conditions. 0 20 40 60 80 100 ee of C3 (%) Diethylsilane 1,1,3,3-Tetramethyldisiloxane Figure 2. Examination of nonlinear effect (1a to 2a). 3 4.0 ing Information). This result is indicative of the involvement of a reversible TM-HAT process. 3.0 ln(er) 2.0 3.3 3.5 3.7 3.9 4.1 4.3 -3 1/T (10 K) Figure 3. Eyring plot using S4 (1a to 2a). Figure 6. Energetically optimized structure of organocobalt(III) intermediates and calculated energetic difference between dia- stereomers. (R)-5a and (S)-5a are derived from 1a. (R)-5r and (S)-5r are derived from 1r. UBP86-D2/6- 311+G(d,p)/IEFPCM//UBP-D2/6-31G(d)/IEFPCM Figure 4. Proposed mechanism. A chiral product from an achiral radical species is formed dur- ing C–O bond formation. Using diethylsilane (S4), the enantiose- lectivity of product (S)-2a was found to be directly proportional to the enantiomeric excess of C3, and nonlinear effects in the chiral induction were not observed (Figure 2).17 This suggests the in- volvement of a single catalyst in the enantiodetermining step. Using 1,1,3,3-tetramethyldisiloxane (S1) forming (R)-2a gave the same result (see Supporting Information). We also found that for S4, variation of the er was not monoton- ic with the temperature (Figure 3). The enantioselective catalysis showed concave Eyring plots characterized by two lines with an inversion point, following the isoinversion principle (Tinv = −10 °C).18 The origin of such nonlinearity is generally taken as evidence
Details
-
File Typepdf
-
Upload Time-
-
Content LanguagesEnglish
-
Upload UserAnonymous/Not logged-in
-
File Pages7 Page
-
File Size-