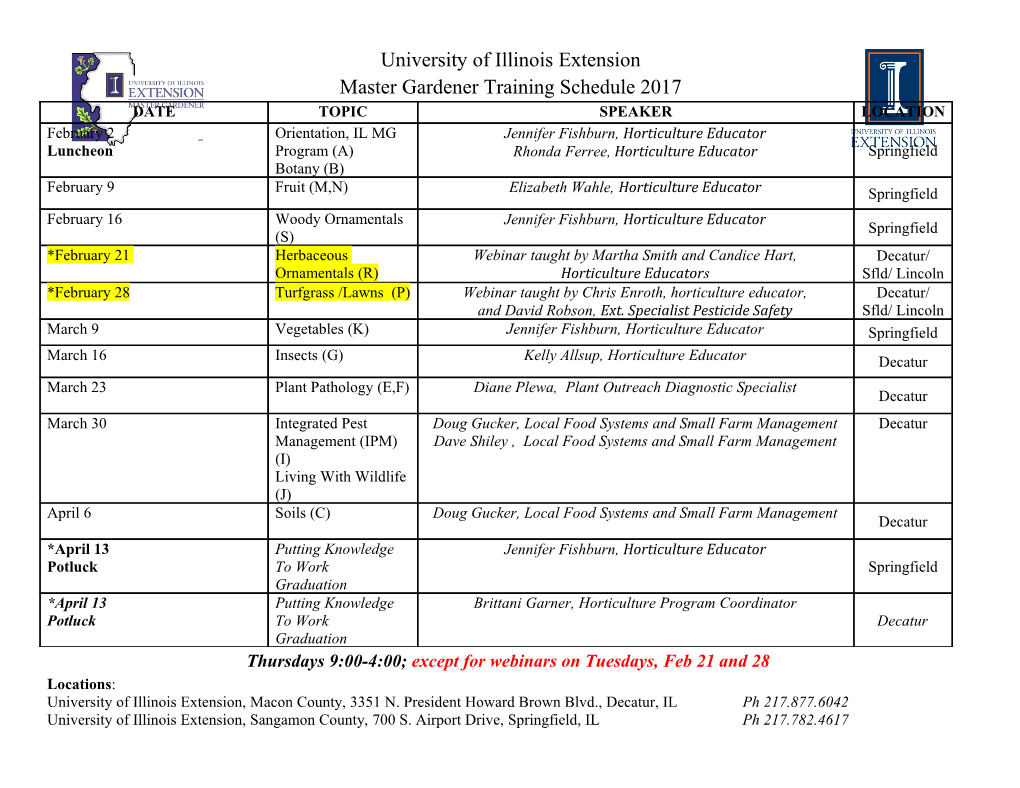
https://ntrs.nasa.gov/search.jsp?R=19700015462 2020-03-23T19:09:28+00:00Z X-327-69-524 PREPRI NT INTERNAL PRESSURES OF A SPACECRAFT OR OTHER SYSTEM OF COMPARTMENTS, CONNECTED IN VARIOUS WAYS AND INCLUDING OUTGASSING MATERIALS, IN A TIME-VARYING 1 PRESSURE ENVIRONMENT J. I. SCIALDONE / INTERNAL PRESSURES OF A SPACECRAFT OR OTHER SYSTEM OF COMPARmMENTS, CONNECTED IN VARIOUS WAYS &W INCLUDING OUTGASSING MATERIALS, IN A TLME-VARYING PRESSURE ENVIRONMENT John 3. Scialdone Test and Evaluation Division Systems Reliability Directorate August 1969 Goddard Space Flight t errter Greenbelt, Mary1ar.d. INTERNAL PRESSURES OF A SPACECMFT OR QTHER SYSTEM OF COMPARTMENTS, CONNECTED IN VARIOUS WAYS AND INCLUDING OUTGASSING MATERIALS, IN A TIME-VARYING PRESSURE E1'IRONMENT Prepared by: fohn Vcialdone Research and Technology Office Approved by hn H. Boeckel eputy Chief, Test and Evaluation Division PROJECT STATUS This report describes a method for calculating the pressure versus time inside each of a system of volumes containing outgassing materials. Authorization Test and Evaluation Charge No. 327-124-12-06-01 iii PRECEDlNG PAGE BLANK NOT FILMED. INTERNAL PRESSURES OF A SPACECRAFT OR OTFIER SYSTEM OF CBMPAR?%lEN'I'S, CONNECTED IN VARIOUS WAYS AND INCLUDING OUTGASSING MATERIAIS, IN A TIME-VARYING PRESSURE ENVIRONMENT John J. Scidd~ne Test and Evaluatim Division SUMMARY A method od calculating the pressure profile for eac:h com- partment of a multi-compartment system such as a spacecraft is presented. Thc method is applicable to volumes connected in series or in parallel or bothby passages of various sizes and geometries, where each volume may include several materials outgassing ac- cox~dingto known or assumed characteristics. The enthe system is exposed to a time-varying pressure environment. .An appropriate set of differe~tialequations with variable co- efficients is derived, which may be solved by computer to provide pressure profiles for each compartment. The profiles obtained zre the result of viscous, intermediate, and molecular flow regimes experienced under appropriate conditions in the system of volumes and passages. Severd computations which have been made are presented and co~nparedto experimental results. Pressure pro- files for volumes with various outgassing materials and various orifice sizes have been calculated and are grouped according to the mole~cularflow regime time constants and the outgassing char- acteristics of the material. From these parametric plots, the quasi-steady pressure in a volume containing outgassing materials can be estimated. PAGE BLANK NOT FILMU), -‘-CEDINGi i'\C CONTENTS INTRODUCTION ...................................... 1 ANALYTICAL MODEL.. ................................. 3 Pressure-Time History Equations ....................... 4 CO~~UC~~S~.C~S..................................... 7 Initial Conditions - Boundary Conditions ................... 13 Outgassing Functions ...................... ', ......... 15 External Pressure ................................... 24 Heat Transfer Considerations .......................... 25 METHOD OF SOLUTION - COMPUTER PROGRAM a 31 COMPARISON OF EXPERIMENTAL AND COMPUTED RESULTS. .... 33 APPLICATIONS AND PAHAMETFUC PRESENTATIONS.. .......... 35 CONCLUSIONS ....................................... 45 RECOMMENDATIONS ................................... 47 REFERENCES ..eee..ae.eaeeemee.e.aeeeeoeeee.eeemmaee 47 APPENDICES A. Typical Computer Input Data and Corresponding Solution (E Chamber) A-1 B. Typical Computer Input Data and Corresponding Solution (3 Chambers) B-1 C. Computer Documentation .............................. C -4. vii ILLUSTRATIONS --Figure 1 Arrangement of Volumes and Passages [Jsed as Model for the Derivation of the Flow Equations . , . .. 2 Degassing Rates Per Unit Surface Area Versus Time for Some Materials . 3 Evacuation of Clean, Dry, Empty 8' x 8' Vacuum Chambe?: for Vacuum Testing of Small Spacecraft. 4 Pressure-Time Test Setup . .. 5 Comparison of Experimental & Computed Recu1ts for a 1-Liter Compartment with Orifice Diameter -437 in., 7 =0.1 sec., Am- bient Temperature . * . = 6 Comparison of Experimental & Computed Results for a 1-Liter Compartment with Orifice Diameter -137 in., 7 =1.0 sec., Ambient Temperature . 7 Comparison of Experimental & Computed Results for a 1-Liter Compartment with Orifice Diameter -040 iu., r =10 sec., Ambient Temperature . .. .. 8 Parametric Curves for a Gne-Compartment Thin Orifice Pres- sure Simulation (7= 0-1 see) at Ambient Temperature. 9 Parametric Curves for a One-Compartment Thin Orifice Pres- sure Simulation (r = 1.0 sec) at Ambient Temperature . 10 Parametric Curves for a One-Compartment Thin Orifice Pres- sure Simulation (7 = 10 sec) at Ambient Temperature . 11 Parametric Curves for a Three-Compartment System Having Thin Orifices, 80 cm2 l1PTFEMOutgassing Material in each Compartment, T = 270°K . .. TABLES Table 1 Coefficients for Conductances . 2 Outgassing Hates Constants for Metallic Materials . 3 Outgassing Rates Constants for Non-Metallic Materials . 4 Coefficients for the Outgassing Equations . 5 Pressure Profile Equations for an 8' x 8' Chamber . 6 ~olurne/Orifice ate rial Combin:dions and Calculated viii SYMBOLS Ai - Surface area in volume i or passage (cm2) area A, - Surface ares of outgassing material (cm2) Conductance through passage irom (1 C i j - sec-') chamber i to chamber j D - Diameter (cm) E - Total internal energy (call Outgassing flow rate in volume i (torr 1 sec-l) Gi = - zQoni (From all materials n contained in Volume i) H - Total enthalpy (cal) J - Tabular value for iwrmediate flow K - Tabular value of moiecular flow in annular section Knudsen's number (dimensionless) Kn - L - Length (cm) Leakage conductance between (1 sec'l) Li j - chambers i and j M - hlolecular weight of gas Po - External pressure P - Absolute pressure in volume i i=l,2,3, 0.0 n PI. - Quasi-static pressure - P - Average pressure Flow rate through passage from (torr 1 sec-' ) Qi j - chamber i to chamber j Leakage flow rate into chamber i (torr 1 sec-') QLi j - from chamber j - 1 Q, - Outgassing rate of surface material (torr 1 oec crn -") after 1 hour vacuum exposure R - Gas const,ant (62.36 torr - 1 gram-' mole OK-') D R - Reynold's nu .hbe r (_UF ) S - Pumping Speed T - Temperature U - Velocity of gas (cm sec-I ) Vi - Volume of chamber i Y - Tabular value for viscous flow in rectangular ducts a - Passage dimension (cm) b - Passage dimension (cm) h - Heat tsmlsport coefficient (cal sec-I cm-2 "K'l) k - Thermal conductivity (cal cm sec-' ~rn-~ OK-~) m. Mass flow rate through passage (grm sec -I) 11 - from chamber i to chamber j Initial outgassing rate per unit area -I) %io - (torr - 1 ~rn-~sec from surface material n in chamber i Final outgassing rate per unit area -2* %i f - (torr 1 cm sec-' ) from surface material n in volume i pi u = ,s 1 - Pressure ratio across an aperture Pi a - Tabular value for nlolecular flow in rectangular ducts C YZF - Specific heat ratio (dimensionless) v 77 - Ratio of viscosity of air @ 15°C to that of a given gas at the same temperature A - Mean free path - Viscosity of gas p - Density of gas 7 - Time constant - Time constant for surface material n in volume i INTERNAL PRESSURES OF A SPACECRAFT OR OTHER SYSTEM OF COMPARTMENTS, CONNECTED IN VARJOUS WAYS AND INCLUDING OUTGASSING MATERIALS, IN A TIME-VARYING PRESSURE ENVIRONMENT INTRODUCTION Many spacecraft failures have been attributed by inference, to improper pressure conditions (Refs. 1 and 2). For example, electrical voltage breakdown has occurred many times during spacecraft testjig or during flight. In some cases it has occurred only during flight with systems which under testing ap- peared to be frc: of this type of problem (Refs. 2 and 3). This breakdown occurs when the ambient gas attains its critical pressure, which is a function of the nature of the gas species, the type of electrodes used and the distance between them, number of charged particles present, and the magnitude of the electric fields. In addition to this difficult problem, some of the other malfunctions and problems dependent on pressure conditions are: * Loss of pressure in sealed components. 0 Loss of lubrication due to evaporation or sublimation. ~eteriorationof adhesives such as loss of plasticizer due to evaporation or sublimation. a Loss of convective heat transfer with resultant development of hot spots or cold spots. Loss of dielectric resistance followed by corona discharge or arcing. Fouling of terminals fc-llowing condensation of outgassed material. ~ossof system calibrat: ;n resulting from unavailability of convective heat transfer at the existin; pressure. a Humidity, aerodynamic performance, radiation and ionization effects, electronic bombardment, etc. a Pressure difference across surfaces sufficient tc cause mechanical failure. Many of the problems listed above can be eliminated or mi.nimized by the use of pressurized containers, coatings, selective insulation, encapsulation, shielding, etc, (Refs. 3 and 4). Also, in many cases possible difficulties can be avoided expediently by employing selective commands for the experiment; e.g., operation of the system at a limited voltage can be commanded for unfavorable pressure conditions, with full voltage operation at favorable pressure. Of course, it is hoped that those problems related to pressure conditions, as well as any other malfunction, will be discovered and eliminated during the thermal-vacuum testing of the spacecraft. These tests attempt to simulate the temperature and pressure encountered during
Details
-
File Typepdf
-
Upload Time-
-
Content LanguagesEnglish
-
Upload UserAnonymous/Not logged-in
-
File Pages89 Page
-
File Size-