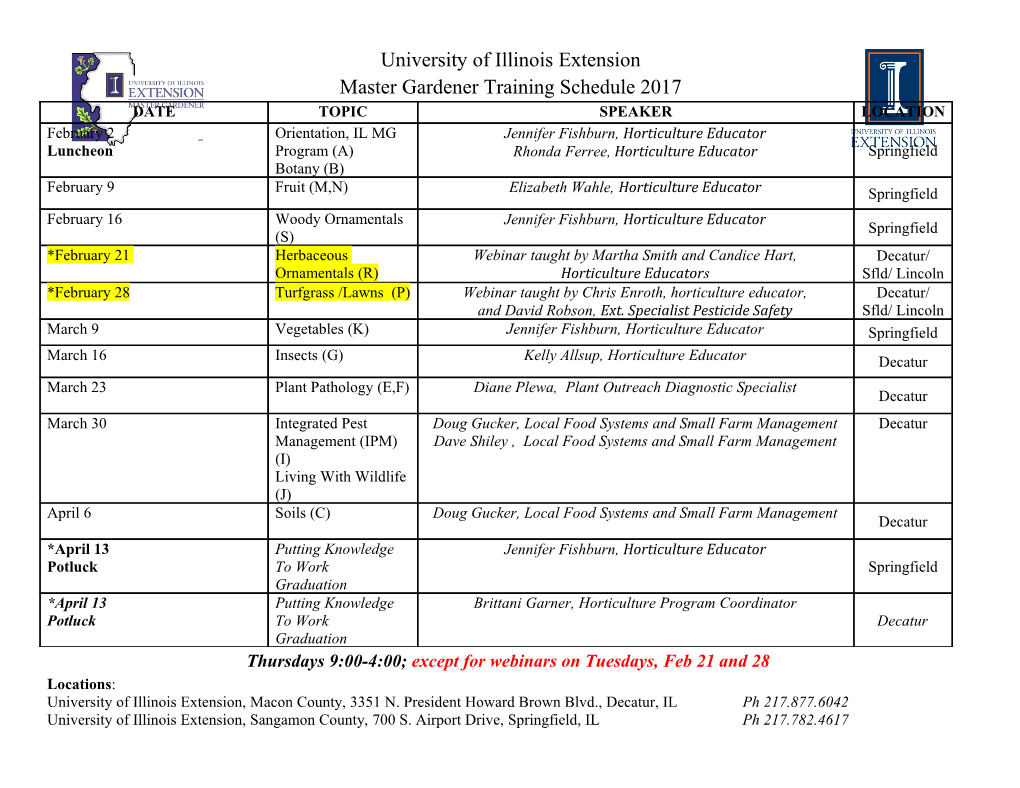
Path Integral in Quantum Field Theory Alexander Belyaev (course based on Lectures by Steven King) Contents 1 Preliminaries 5 1.1 Review of Classical Mechanics of Finite System . 5 1.2 Review of Non-Relativistic Quantum Mechanics . 7 1.3 Relativistic Quantum Mechanics . 14 1.3.1 Relativistic Conventions and Notation . 14 1.3.2 TheKlein-GordonEquation . 15 1.4 ProblemsSet1 ........................... 18 2 The Klein-Gordon Field 19 2.1 Introduction............................. 19 2.2 ClassicalScalarFieldTheory . 20 2.3 QuantumScalarFieldTheory . 28 2.4 ProblemsSet2 ........................... 35 3 Interacting Klein-Gordon Fields 37 3.1 Introduction............................. 37 3.2 PerturbationandScatteringTheory. 37 3.3 TheInteractionHamiltonian. 43 3.4 Example: K π+π− ....................... 45 S → 3.5 Wick’s Theorem, Feynman Propagator, Feynman Diagrams . .. 47 3.6 TheLSZReductionFormula. 52 3.7 ProblemsSet3 ........................... 58 4 Transition Rates and Cross-Sections 61 4.1 TransitionRates .......................... 61 4.2 TheNumberofFinalStates . 63 4.3 Lorentz Invariant Phase Space (LIPS) . 63 4.4 CrossSections............................ 64 4.5 Two-bodyScattering . 65 4.6 DecayRates............................. 66 4.7 OpticalTheorem .......................... 66 4.8 ProblemsSet4 ........................... 68 1 2 CONTENTS 5 Path Integrals in Quantum Mechanics 69 5.1 Introduction............................. 69 5.2 The Point to Point Transition Amplitude . 70 5.3 ImaginaryTime........................... 74 5.4 Transition Amplitudes With an External Driving Force . ... 77 5.5 Expectation Values of Heisenberg Position Operators . .... 81 5.6 Appendix .............................. 83 5.6.1 GaussianIntegration . 83 5.6.2 Functionals ......................... 85 5.7 ProblemsSet5 ........................... 87 6 Path Integral Quantisation of the Klein-Gordon Field 89 6.1 Introduction............................. 89 6.2 TheFeynmanPropagator(again) . 91 6.3 Green’s Functions in Free Field Theory . 94 6.4 Green’s Functions for λφ4 Theory ................. 97 6.5 The 2 2ScatteringAmplitudefromLSZ . 102 → 6.6 Appendix: ProofofEq.6.4.6 . 104 6.7 ProblemsSet6 ........................... 105 7 The Dirac Equation 107 7.1 Relativistic Wave Equations: Reprise . 107 7.2 TheDiracEquation. 108 7.3 Free Particle Solutions I: Interpretation . 109 7.4 FreeParticleSolutionsII:Spin. 112 7.5 Normalisation,GammaMatrices. 114 7.6 LorentzCovariance . 115 7.7 Parity ................................ 119 7.8 BilinearCovariants . 119 7.9 ChargeConjugation. 120 7.10Neutrinos .............................. 121 8 The Free Dirac Field 125 8.1 CanonicalQuantisation. 125 8.2 PathIntegralQuantisation. 127 8.3 TheFeynman PropagatorfortheDiracField. 129 8.4 Appendix: Grassmann Variables . 131 9 The Free Electromagnetic Field 133 9.1 The Classical Electromagnetic Field . 133 9.2 CanonicalQuantisation. 136 9.3 PathIntegralQuantisation. 138 CONTENTS 3 10 Quantum Electrodynamics 141 10.1 FeynmanRulesofQED . 141 10.2 Electron–MuonScattering . 143 10.3 Electron–ElectronScattering. 145 10.4 Electron–Positron Annihilation . 146 10.4.1 e+e− e+e− ........................ 146 → 10.4.2 e+e− µ+µ− and e+e− hadrons. 146 10.5 ComptonScattering→ . .→ . 147 4 CONTENTS Chapter 1 Preliminaries 1.1 Review of Classical Mechanics of Finite Sys- tem Consider a finite classical system of particles whose generalised coordinates are qi where i =1,...,N. For example N =3n for n particles in three dimensions. Suppose the Lagrangian given by L = T V , T and V being the kinetic and − potential energy, is: L = L(qi, q˙i) (1.1.1) whereq ˙i denotes the time derivative of qi. For each set of paths qi(t) connecting (qi1, t1) to (qi2, t2) the action is defined by: t2 S[qi(t)] = Ldt (1.1.2) Zt1 Note that S is a functional, i.e. a number whose value depends on a set of functions qi(t). The classical paths are those which minimise (or in general extremise) the numerical value of S, subject to the boundary conditions δqi =0 at the initial and final times t1 and t2. This is the well-known action principle (which can be derived from Feynman’s path integral approach to quantum mechanics – see later) and it leads to the N second order (in time) differential Lagrange equations of motion: d ∂L ∂L =0 (1.1.3) dt ∂q˙i − ∂qi In the Lagrange formalism the independent variables are the coordinates qi and the velocitiesq ˙i. The generalised momenta pi are derived quantities defined by: ∂L pi (1.1.4) ≡ ∂q˙i In the Hamiltonian formalism the variablesq ˙i are traded in for pi, and instead of working with a Lagrangian L = L(qi, q˙i) one works with a Hamiltonian 5 6 CHAPTER 1. PRELIMINARIES H = H(qi,pi) defined by the so-called Legendre transformation: H(q ,p ) p q˙ L(q , q˙ ) (1.1.5) i i ≡ i i − i i Xi where it is understood that theq ˙i are to be written as functions of qi and pi. From Eqs.1.1.3-1.1.5 one readily obtains the Hamilton equations of motion: ∂H ∂H p˙i = , q˙i = (1.1.6) − ∂qi ∂pi which are 2N first order (in time) differential equations. Just as (for a conser- vative force) L = T V , so H = T + V , so the Hamiltonian is just the total − energy in this case. Example 1: The Harmonic Oscillator First consider a single oscillator in oscillating in one dimension (e.g. mass on a spring) The Lagrangian is: 1 1 L = T V = mq˙2 ω2q2 (1.1.7) − 2 − 2 The generalised momentum is: ∂L p = = mq˙ (1.1.8) ∂q˙ The Hamiltonian is: p2 ω2 H = pq˙ L = + q2 (1.1.9) − 2m 2 Example 2: Coupled Harmonic Oscillators Now consider a linear chain of coupled oscillators, say corresponding to a vibrating line of atoms. Let the coordinate qi denote the displacement of the i th atom in the line from its equilibrium position, where we assume that all displacements− correspond to longitudinal vibrations along the length of the line of atoms. To make the system finite, let us form a closed loop of N atoms such that qi+N = qi. The Lagrangian is: N 1 Ω2 Ω2 L = q˙ 2 (q q )2 0 (q )2 (1.1.10) 2 i − 2 i − i+1 − 2 i Xi=1 where we have taken the atoms to have unit mass, and have included a nearest neighbour coupling plus a second independent frequency Ω0. The Lagrange equations of motion are: q¨ = Ω2(2q q q ) Ω2q (1.1.11) i − i − i−1 − i+1 − 0 i 1.2. REVIEW OF NON-RELATIVISTIC QUANTUM MECHANICS 7 The generalised momenta are: ∂L pi = =q ˙i (1.1.12) ∂q˙i The Hamiltonian is: N 1 Ω2 Ω2 H = p 2 + (q q )2 + 0 (q )2 (1.1.13) 2 i 2 i − i+1 2 i Xi=1 Normal Modes The oscillators above can be uncoupled by taking a discretised Fourier transform of the form N/2 q˜ q = k eij2πk/N (1.1.14) j N 1/2 k=X−N/2 N/2 p˜ p = k e−ij2πk/N (1.1.15) j N 1/2 k=X−N/2 which satisfies the periodic boundary conditions. Note that i2 = 1 and we have labelled the oscillators by j to avoid confusion. − Since the qj are real theq ˜k andp ˜k are complex and satisfy ⋆ ⋆ q˜−k =q ˜k, p˜−k =p ˜k. (1.1.16) Using the result: N ij2π(k−k′)/N e = δkk′ N jX=1 the Hamiltonian becomes, 1 N/2 H = p˜ p˜⋆ + ω2q˜ q˜⋆ (1.1.17) 2 k k k k k k=X−N/2 which corresponds to a sum of uncoupled harmonic oscillators of frequencies given by 2 2 2 2 ωk = Ω 4 sin (πk/N) + Ω0 (1.1.18) 1.2 Review of Non-Relativistic Quantum Me- chanics In quantum mechanics, 1 physical states are represented by vectors ψ > in a vector space (Hilbert space). Physical observables are represented by| linear 1We shall set h/(2π)= c = 1 throughout. 8 CHAPTER 1. PRELIMINARIES Hermitian operatorsω ˆ and the result of a measurement of some observableω ˆ is one of its (real) eigenvalues ω where ωˆ ω>= ω ω> (1.2.1) | | A very useful result is the completeness relation: ∞ I = ω ><ω Z−∞ | | which follows from the fact that the eigenvectors of an Hermitian operator form a complete orthonormal basis, where I is the unit operator. If the system is in some state ψ > and some observableω ˆ is measured then the system will be forced into one| of the eigenstates ψ > ω >. The result of the measurement | →| will be one of the eigenvalues ω which is not classically predictable, but which occurs with a probability P (ω) given by <ω ψ> 2 P (ω)= | | | (1.2.2) < ψ ψ > | The average or expectation value is therefore, < ψ ωˆ ψ > < ωˆ >= | | (1.2.3) < ψ ψ > | Now let us assume we are given a classical Hamiltonian in some particular basis of coordinates H = H(qi,pi). According to quantum mechanics we must replace the number-valued variables qi and pi by Hermitian operators which satisfy the commutation relations: [ˆqi, pˆj]= iIδij, [ˆqi, qˆj]=[ˆpi, pˆj]=0 (1.2.4) where I is the unit operator, 0 is the null operator, [A, B]= AB BA and δij is the Kronecker delta symbol defined in the usual way − δij =1 (i = j) δ =0 (i = j) ij 6 Each coordinate has its own complete set of eigenvectors and real eigenvalues qˆ q >= q q >, pˆ p >= p p > i| i i| i i| i i| i The position and momentum space wavefunctions are thus ψ(q , q ,...)=< q < q . ψ > 1 2 1| 2| | and ψ(p ,p ,...)=<p <p . ψ > 1 2 1| 2| | 1.2. REVIEW OF NON-RELATIVISTIC QUANTUM MECHANICS 9 In one dimension we have simply, ψ(q)=< q ψ >, ψ(p)=<p ψ > | | The way the time dependence of a quantum system is described is a matter of taste and convenience.
Details
-
File Typepdf
-
Upload Time-
-
Content LanguagesEnglish
-
Upload UserAnonymous/Not logged-in
-
File Pages150 Page
-
File Size-