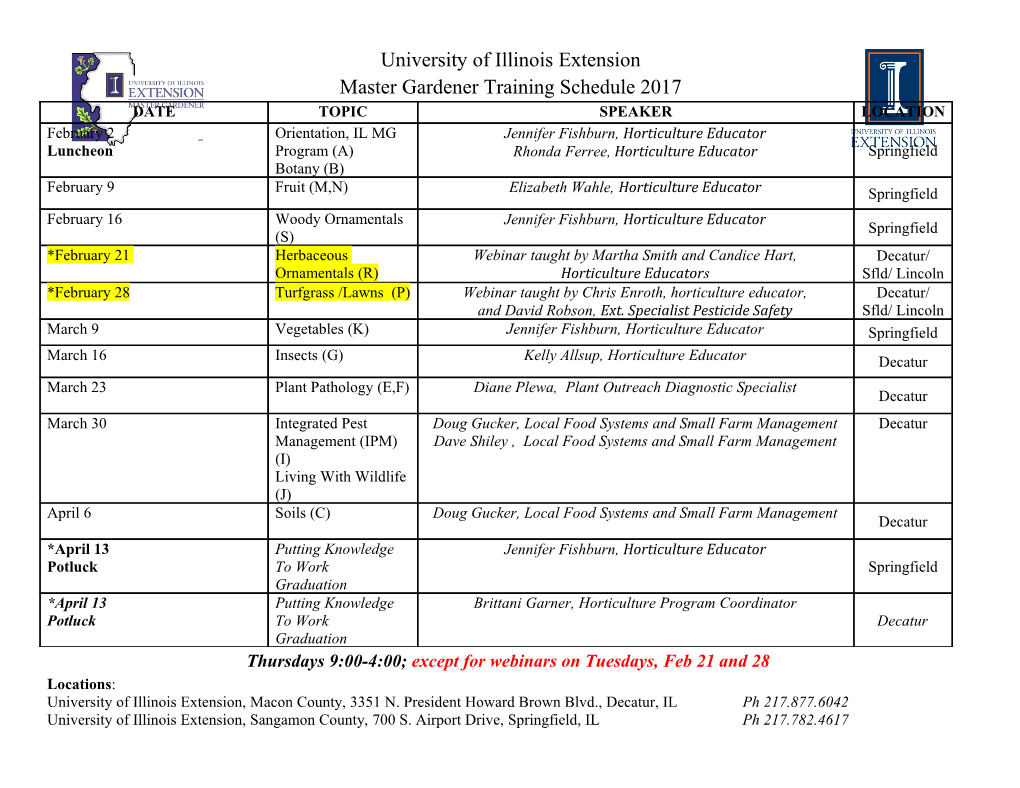
Lecture 3:The Lebesgue Integral 1 of 14 Course: Theory of Probability I Term: Fall 2013 Instructor: Gordan Zitkovic Lecture 3 The Lebesgue Integral The construction of the integral Unless expressly specified otherwise, we pick and fix a measure space (S, S, m) and assume that all functions under consideration are defined there. Definition 3.1 (Simple functions). A function f 2 L0(S, S, m) is said to be simple if it takes only a finite number of values. The collection of all simple functions is denoted by LSimp,0 (more precisely by LSimp,0(S, S, m)) and the family of non-negative simple Simp,0 functions by L+ . Clearly, a simple function f : S ! R admits a (not necessarily unique) representation n f = ∑ ak1Ak , (3.1) k=1 for a1,..., an 2 R and A1,..., An 2 S. Such a representation is called the simple-function representation of f . When the sets Ak, k = 1, . , n are intervals in R, the graph of the simple function f looks like a collection of steps (of heights a1,..., an). For that reason, the simple functions are sometimes referred to as step functions. The Lebesgue integral is very easy to define for non-negative simple functions and this definition allows for further generalizations1: 1 In fact, the progression of events you will see in this section is typical for measure theory: you start with indica- Definition 3.2 (Lebesgue integration for simple functions). For f 2 tor functions, move on to non-negative Simp,0 R simple functions, then to general non- L+ we define the (Lebesgue) integral f dm of f with respect to m negative measurable functions, and fi- by nally to (not-necessarily-non-negative) Z n f dm = a m(A ) 2 [0, ¥], measurable functions. This approach is ∑ k k so common, that it has a name - the = k 1 Standard Machine. = n where f ∑k=1 ak1Ak is a simple-function representation of f , Problem 3.1. Show that the Lebesgue integral is well-defined for sim- n ple functions, i.e., that the value of the expression ∑k=1 akm(Ak) does not depend on the choice of the simple-function representation of f . Last Updated: September 28, 2013 Lecture 3:The Lebesgue Integral 2 of 14 Remark 3.3. 1. It is important to note that R f dm can equal +¥ even if f never takes the value +¥. It is enough to pick f = 1A where m(A) = +¥ - indeed, then R f dm = 1m(A) = ¥, but f only takes values in the set f0, 1g. This is one of the reasons we start with non-negative functions. Otherwise, we would need to deal with the (unsolvable) problem of computing ¥ − ¥. On the other hand, such examples cannot be constructed when m is a finite measure. Indeed, it is easy to show that when m(S) < ¥, we have R f dm < ¥ for all Simp,0 f 2 L+ . 2. One can think of the (simple) Lebesgue integral as a generalization of the notion of (finite) additivity of measures. Indeed, if the simple- = n function representation of f is given by f ∑k=1 1Ak , for pairwise disjoint A1,..., An, then the equality of the values of the integrals n for two representations f = 1[n and f = ∑ 1 is a simple k=1 Ak k=1 Ak restatement of finite additivity. When A1,..., An are not disjoint, then the finite additivity gives way to finite subadditivity n n m([k=1 Ak) ≤ ∑ m(Ak), k=1 but the integral R f dm “takes into account” those x which are cov- ered by more than one Ak, k = 1, . , n. Take, for example, n = 2 and A1 \ A2 = C. Then f = 1 + 1 = 1 + 1 + 1 A1 A2 A1nC 2 C A2nC, and so Z f dm = m(A1 n C) + m(A2 n C) + 2m(C) = m(A1) + m(A2) + m(C). Simp,0 It is easy to see that L+ is a convex cone, i.e., that it is closed under finite linear combinations with non-negative coefficients. The integral map f 7! R f dm preserves this structure: Simp,0 Problem 3.2. For f1, f2 2 L+ and a1, a2 ≥ 0 we have R R 1. if f1(x) ≤ f2(x) for all x 2 S then f1 dm ≤ f2 dm, and R R R 2. (a1 f1 + a2 f2) dm = a1 f1 dm + a2 f2 dm. Simp,0 2 2 Having defined the integral for f 2 L+ , we turn to general Even though there is no obvious advan- non-negative measurable functions. In fact, at no extra cost we can tage at this point of integrating a func- tion which takes the value +¥, it will consider a slightly larger set consisting of all measurable [0, ¥]-valued become clear soon how convenient it re- 0 ally is. functions which we denote by L+ [0, ¥] . Last Updated: September 28, 2013 Lecture 3:The Lebesgue Integral 3 of 14 Definition 3.4 (Lebesgue integral for nonnegative functions). For a Note: While there is no question that function f 2 L0 [0, ¥], we define its Lebesgue integral R f dm by this definition produces a unique num- + ber R f dm, one can wonder if it matches Z Z the previously given definition of the Simp,0 Lebesgue integral for simple functions. f dm = sup g dm : g 2 L+ , g(x) ≤ f (x), 8 x 2 S 2 [0, ¥]. A simple argument based on the mono- tonicity property of part 1. of Problem R = 3.2 can be used to show that this is, in- Problem 3.3. Show that f dm ¥ if there exists a measurable set A deed, the case. with m(A) > 0 such that f (x) = ¥ for x 2 A. On the other hand, show that R f dm = 0 for f of the form 8 <¥, x 2 A, f (x) = ¥1A(x) = :0, x 6= A, whenever m(A) = 0. Note: Relate this to our convention that ¥ × 0 = 0 × ¥ = 0. Finally, we are ready to define the integral for general measurable functions. Each f 2 L0 can be written as a difference of two functions 0 in L+ in many ways. There exists a decomposition which is, in a sense, minimal. We define f + = max( f , 0), f − = max(− f , 0), so that f = f + − f − (and both f + and f − are measurable). The mini- mality we mentioned above is reflected in the fact that for each x 2 S, at most one of f + and f − is non-zero. Definition 3.5 (Integrable functions). A function f 2 L0 is said to be integrable if Z Z f + dm < ¥ and f − dm < ¥. The collection of all integrable functions in L0 is denoted by L1. The family of integrable functions is tailor-made for the following defini- tion: Definition 3.6 (The Lebesgue integral). For f 2 L1, we define the Lebesgue integral R f dm of f by Z Z Z f dm = f + dm − f − dm. Remark 3.7. 1. We have seen so far two cases in which an integral for a function f 2 L0 can be defined: when f ≥ 0 or when f 2 L1. It is possible to combine the two and define the Lebesgue integral for all functions f 2 L0 with f − 2 L1. The set of all such functions is denoted by Last Updated: September 28, 2013 Lecture 3:The Lebesgue Integral 4 of 14 L0−1 and we set Z Z Z f dm = f + dm − f − dm 2 (−¥, ¥], for f 2 L0−1. Note that no problems of the form ¥ − ¥ arise here, and also note 0 0−1 that, like L+, L is only a convex cone, and not a vector space. While the notation L0 and L1 is quite standard, the one we use for L0−1 is not. 0−1 R R 2. For A 2 S and f 2 L we usually write A f dm for f 1A dm. Problem 3.4. Show that the Lebesgue integral remains a monotone operation in L0−1. More precisely, show that if f 2 L0−1 and g 2 L0 are such that g(x) ≥ f (x), for all x 2 S, then g 2 L0−1 and R g dm ≥ R f dm. First properties of the integral The wider the generality to which a definition applies, the harder it is to prove theorems about it. Linearity of the integral is a trivial matter Simp,0 for functions in L+ , but you will see how much we need to work to 0 get it for L+. In fact, it seems that the easiest route towards linearity is through two important results: an approximation theorem and a convergence theorem. Before that, we need to pick some low-hanging fruit: 0 Problem 3.5. Show that for f1, f2 2 L+ [0, ¥] and a 2 [0, ¥] we have R R 1. if f1(x) ≤ f2(x) for all x 2 S then f1 dm ≤ f2 dm. 2. R a f dm = a R f dm. Theorem 3.8 (Monotone convergence theorem). Let f fngn2N be a se- 0 quence in L+ [0, ¥] with the property that f1(x) ≤ f2(x) ≤ ... for all x 2 S. Then Z Z lim fn dm = f dm, n 0 where f (x) = limn fn(x) 2 L+ [0, ¥] , for x 2 S. Proof. The (monotonicity) property (1) of Problem 3.5 above implies R R R that the sequence fn dm is non-decreasing and that fn dm ≤ f dm.
Details
-
File Typepdf
-
Upload Time-
-
Content LanguagesEnglish
-
Upload UserAnonymous/Not logged-in
-
File Pages14 Page
-
File Size-