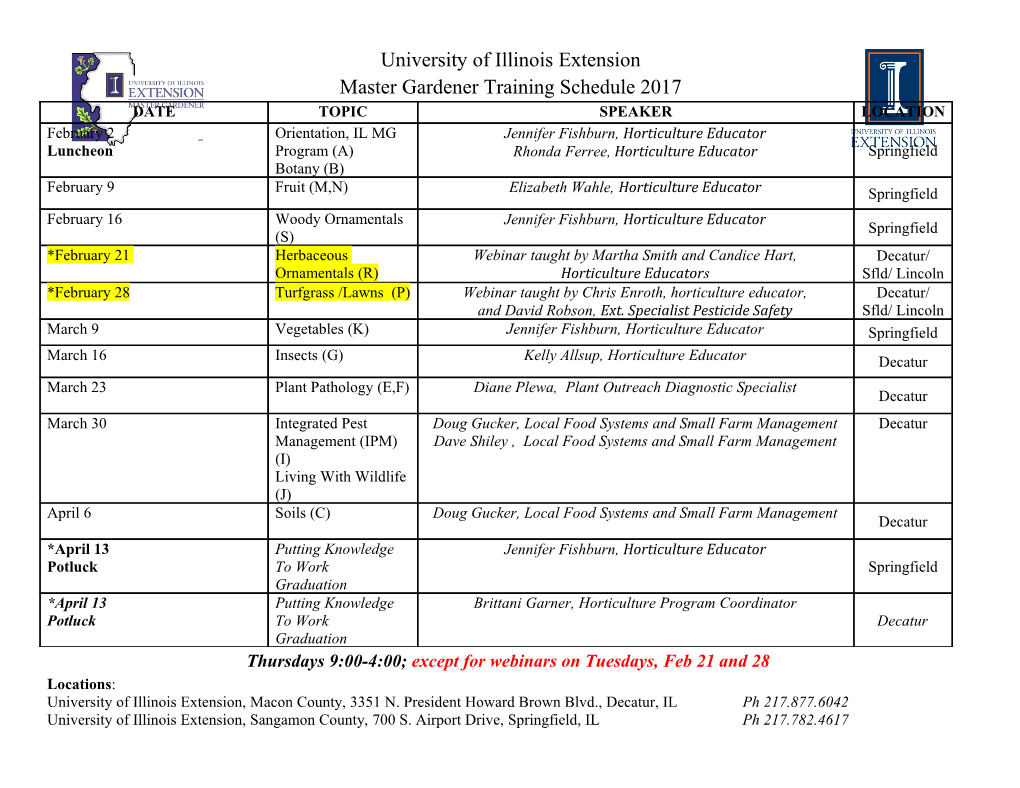
Microbial Fuel Cells for Improved Bioremediation Emi Lemberg1, Joe Vallino2 1University of Chicago 5801 S Ellis Ave, Chicago IL 60637 USA 2The Ecosystems Center: Semester in Environmental Science Marine Biological Laboratory Woods Hole, Massachusetts 02543 USA 2017 Fall Semester 1 Abstract Microbial fuel cells (MFCs) can be used as a power source and as a tool for bioremediation. By creating an electrical connection between the anaerobic sediments and aerobic water column, a MFC can increase the metabolism rates of bacteria in the sediment, allowing the bacteria to break down complex molecules they would not be able to consume otherwise. This experiment focuses on the breakdown of organic matter in sediments from Little Pond in Falmouth, Massachusetts. While the sediments already contain high concentrations of organic matter, caffeine was amended to the sediments to as a proxy for difficult to degrade pollutants. This experiment examines the effect of an added 2 V potential has on of organic matter decomposition in a sediment MFC (Driven MFC). During the incubation, a pH gradient developed in the Driven MFC, with a change in pH of 1.12 on the final day of incubation. The pH gradient caused the current of the MFCs to drop from approximately 2.5 mA to approximately 0.05 mA halfway through the experiment. Additionally, the high pH at the surface of the MFC resulted in the uptake of carbon dioxide for the first 17 days of the incubation. Organic carbon extracted from the sediments suggests that a decrease in the carbon content of sediments occurred; however, the short-term nature of the incubations prevented a statistically significant conclusion when compared to the controls. Introduction Bioremediation is a process in which microorganisms are used to break down pollutants in contaminated sites. Currently, the common method used to remove polluted sediments is to excavate the sediments and transport them in a landfill (Chun et. al, 2012). However, this is an extremely expensive, invasive and destructive method to remove pollutants. Bioremediation offers a solution that is less destructive to the ecosystem and less expensive (Chun et. al, 2012). Ongoing research investigates ways to improve the efficiency of bioremediation tools. One potential tool that can be used for bioremediation is a microbial fuel cell (MFC). When bacteria break down organic matter, it produces carbon dioxide, protons, and electrons. The bacteria gain more energy in breaking down organic matter by using electron acceptors with high electric potentials, such as oxygen. Ideally, the bacteria donate electrons to oxygen molecules, which can combine with hydrogen to produce water. However, when bacteria live in sediments, which are typically anaerobic environments, they are not able to easily access oxygen. Some bacteria are able to access electrons from the oxygen in the water column by using natural shuttles such as iron oxide materials (Li et al., 2015). However, these electron transfers are weak due to low concentrations of electron mediating substances in the sediment (Li et al., 2015). Similarly, some cable bacteria are able to form chains to reach the surface of the sediments (Schauer 2014). However, in most cases bacteria must transfer their electrons to less 2 energetically favorable reactions, such as to sulfate, which lowers the amount of energy they receive and inhibits their ability to break down difficult to degrade organic matter. A MFC functions in a manner similar to common batteries. However, the chemistry in the MFC is catalyzed by the metabolism of bacteria (Figure 1). A MFC is created by placing the anode into the sediment, an anaerobic environment, while the cathode is placed in the water column, an anaerobic environment. The following reaction, catalyzed by the consumption of organic matter (CH2O) by bacteria, occurs at the anode: + - CH2O + H2O -> CO2 + 4H + 4e The anode is the electron acceptor, which transfers the microbes’ electrons from the sediments to the water column through a wire where the electrons are donated to oxygen. The following reaction occurs at the cathode: + - 4H + 4e +2O2 -> 2H2O By living on the MFC anode, microbes are able to utilize the reaction with the greatest electric potential and break down organic matter that cannot be broken down under anaerobic conditions, or is decomposed slowly. Earlier research on MFCs focused on creating batteries that can produce a current to power another instrument necessary to monitor the site while cleaning up biotoxins (Santoro 2017). Additionally, MFCs can be used by waste water treatment plants to break down organic matter, particularly sulfides, and produce electricity (Du et. al., 2007). However, another use for MFCs is to use a similar design to increase rates of bioremediation. One method that is being tested is applying an external voltage to the fuel cell. The external voltage will further increase the electric potential between the anode and cathode, which should increase the rates of bioremediation (Figure 1b). For example, Bellagamba et. al. (2016) tested the effects of applying two different voltages to MFCs. One cell received 2 Volts continuously, while the other received 2 Volts at an intermediate rate (three days of voltage, four days off); however, Bellagamba et. al. (2016) observed very little difference in the rate of biotoxin removal between the two fuel cells. Our experiment examined the rate of pollutant removal in sediments based on three different MFC designs: a control where the anode and cathode were not connected (Control MFC), a single fuel cell without external voltage (Passive MFC), and a fuel cell with a controlled added external voltage (Driven MFC). Additionally, caffeine was added to the sediments as a pollutant surrogate. 3 Methods Sediment Sediment samples for this experiment were collected from Little Pond, a local estuary in Falmouth that experiences pollutant loading from fertilizers, septic system leaching, pavement runoff and other sources. While the sediments are highly nutrient loaded, we could not identify a specific pollutant that could be measured directly. Therefore, caffeine was added to the sediments so that the change in carbon content could be measured quantitatively. In order to create a high concentration of caffeine in the sediments, 35 grams of caffeine (Sigma-Aldrich) was added to 3,5 L of wet sediment from Little Pond. This resulted in a caffeine concentration of 10,000 ppm. Each MFC was filled with 550 mL of the caffeine amended sediment. Fuel Cell Construction Six MFCs were created for this experiment, two for each MFC design (Control, Passive, and Driven) (Figure 2). Each MFC was constructed out of a 1 L wide mouth polypropylene plastic jar that was 15.24 cm tall and 9.2 cm wide. For the Passive and Driven MFCS, two holes were drilled into the side of the jar, one 20 cm from the bottom and one 80 cm from the bottom. These two holes allowed the wiring from the anode and cathode to be accessed outside of the cell. Additionally, two holes were drilled into the lid of each fuel cell in order to measure carbon dioxide fluxes using the LiCor 4200. LuerLocks were hot glue gunned into the holes to control the flow of air between the cell and the LiCor. When carbon dioxide was not being monitored, the jars remained open without the lids on to ensure the water did not become anaerobic over time. The Control MFCs had the same lid design, but no holes were drilled into the side of the jars. Anode and Cathode Construction The anode and cathode were constructed out of plain weave carbon fiber mesh (carbonfiber cloth, 0-90 plain weave, Jamestown Distributors, Bristol, RI). The carbon fiber was heated in a muffler oven for one hour at 450 ˚C, then cut into circles with 3 inch diameters. In the Control MFCs, the carbon fiber circles were placed directly into the fuel cells at the same depths as the passive and control MFCs. In the Passive and Driven MFCs, the anode and cathodes were attached to titanium wires (RBA Depot, 22 ga., Gr.1 - 99.5%, Amazon) using silver epoxy (MG Chemicals Silver Epoxy Adhesive, 8331-14G, Amazon). Silver epoxy was chosen because it is conductive and would not interfere with the flow of electrons. Nanosilver is toxic to bacterial communities, by releasing silver ions which can cause cell lysing (Seltenrich, 2013). However, the silver expoxy is made of larger silver particles and was used in small portions so as not to affect the bacteria significantly. Titanium wire was chosen because it will not rust in aquatic environments. 4 Applying External Voltage and Monitoring Current To create the Driven MFCs, an additional 2 V were provided to the fuel cells. A D2A (digital- to-analog) board was used to drive the voltage (WTDAC-M, Weeder Technologies, Walton Beach FL), and a A2D (analog-to-digital) board (WTAIN-M, Weeder Technologies) was used to read the voltage drop across the resistor (Fig. 2). The A2D board was also used to monitor the voltages of the passive MFCs in experiment. From the voltage drop across the resister, current was calculated using the following equation: 푉 퐼 = 푅 Where I represents current, V represents voltage, and R represents resistance. In this experiment, a 47 Ω resistor was used. pH Surface pH was measured every day using a Accument AB 15/15+ bench-top pH meter, starting on Day 4 of the experiment. Starting on Day 15, the pH at the bottom of the fuel cells was also measured every day to observe the proton gradient present in the MFCs. Samples were taken from the fuel cells using a 10mL glass pipette, then bubbled for 10 minutes with nitrogen gas to keep the samples anoxic before the pH measurement was taken. On the last day of the experiment, the pH of each MFC was measured in the surface water, the top sediments, the middle sediments, and the bottom sediments.
Details
-
File Typepdf
-
Upload Time-
-
Content LanguagesEnglish
-
Upload UserAnonymous/Not logged-in
-
File Pages26 Page
-
File Size-