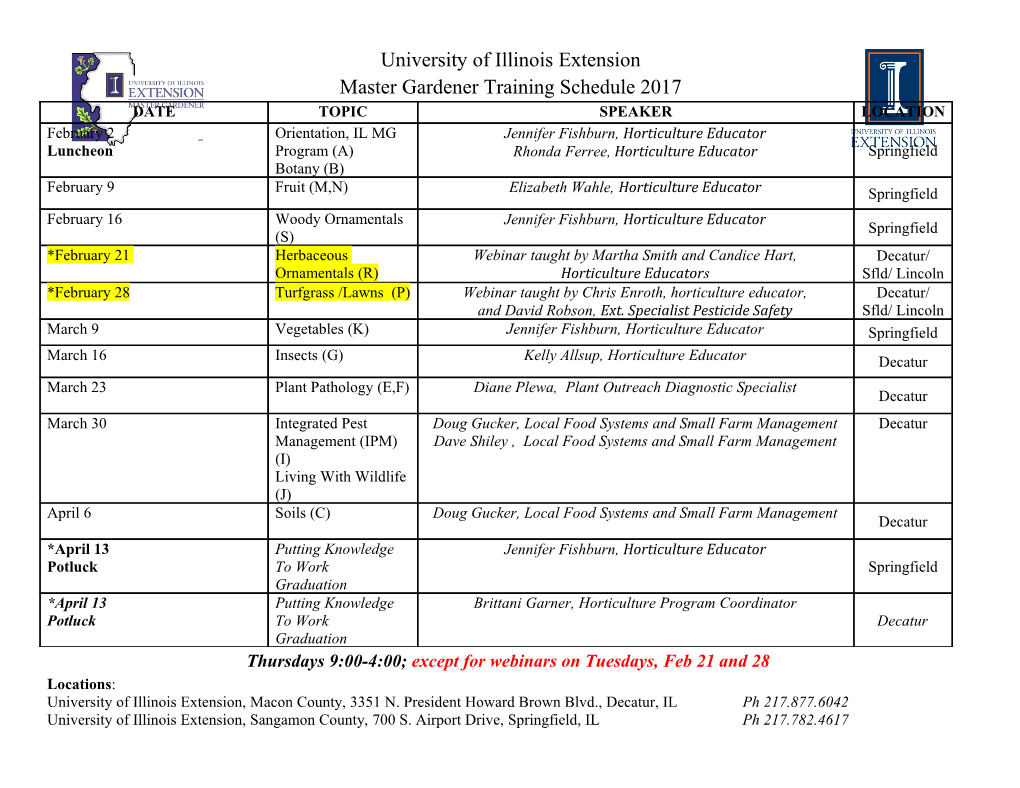
Sediment transport and deposition at river mouths: A synthesis L. D. WRIGHT Coastal Studies Unit, Department of Geography, The University of Sydney, Sydney, N.S.W., Australia 2006 ABSTRACT Yoshida (1967, 1969, 1971), Waldrop and Farmer (1973), Scruton (1956, 1960), Komar (1973), Garvine (1974,1975), Wright (1970, River-mouth process studies and comparisons of river-mouth 1971), Wright and Coleman (1971, 1972, 1973, 1974), and forms from contrasting environments suggest that sediment disper- Wright and others (1973). Synthesis of the above studies suggests sal and accumulation patterns are governed by three basic effluent that river-mouth variability can best be understood by considering forces and by tide- or wave-induced processes. Neglecting river-mouth systems as the resultants of varying contributions from modifications by tides or waves, effluent behavior and consequent a few primary and modifying forces. The primary river-mouth depositional patterns depend on the relative dominance of (1) out- forces are related directly to the interactions between effluent and flow inertia, (2) turbulent bed friction seaward of the mouth, and basin waters, and they rely on the river outflow for their driving (3) outflow buoyancy. Inertia-dominated effluents are charac- energy. These primary forces and their depositional products are terized by fully turbulent jet diffusion, exhibit low lateral spreading modified to varying degrees by tides and waves. angles and progressive lateral and longitudinal deceleration, and produce narrow river-mouth bars. Under most natural circum- PRIMARY PROCESSES AND FORMS stances, inertial effects are equaled or exceeded by either turbulent bed friction or effluent buoyancy. When the tidal range and incident wave power of the receiving Shallow depths immediately basinward of a river mouth enhance basin are negligible or small relative to the strength of river out- the effects of bed friction, causing more rapid deceleration and flow, river-dominated configurations result (Wright and Coleman, lateral expansion. Triangular "middle-ground" bars and frequent 1972, 1973). River-dominant situations characterize river mouths channel bifurcation result. Low tidal ranges, fine-grained sediment in microtidal lakes, estuaries, enclosed or semi-enclosed seas, or loads, and deep outlets favor strong density stratification within the regions fronted by flat offshore slopes which attenuate wave lower reaches of the channels. Under such circumstances, effluents power. In such cases, one or more of three primary forces will are dominated by the effects of buoyancy for at least part of the dominate: (1) inertia and associated turbulent diffusion; (2) turbu- year. Buoyant effluents produce narrow distributary mouth bars, lent bed friction; or (3) buoyancy. The associated effluent processes elongate distributaries with parallel banks, and few bifurcations. are illustrated in Figures 1 through 3. The role played by each In macrotidal environments where tidal currents are stronger force depends on factors such as the discharge rate and outflow than river flow, bidirectional currents redistribute river sediments, velocity of the stream, water depths in and seaward of the river producing sand-filled, funnel-shaped distributaries and causing mouths, the amount and grain size of the sediment load, and the linear tidal ridges to replace the distributary mouth bar. Powerful sharpness of density contrasts between the river and basin waters. waves promote rapid effluent diffusion and decleration and pro- High outflow velocities, small density contrasts, and deep water duce constricted or deflected river mouths. immediately seaward of the mouth permit inertial forces to domi- nate, causing the effluent to behave as a fully turbulent jet (Fig. 1). INTRODUCTION When bed-load transport is large and water depths seaward of the mouth are shallow, turbulent diffusion becomes restricted to the River mouths are the dynamic dispersal points of river-derived horizontal while bottom friction increases deceleration and expan- sediments which contribute to delta formation. They are con- sion rates (Fig. 2). Where the river mouth is deep relative to the sequently the most fundamental elements of deltaic systems. riverine discharge, sea water enters the mouth as a salt wedge, and River-mouth processes involve a variety of interactions between the buoyancy of the lighter river water becomes dominant; the riverine and marine waters. These processes acting in combination effluent then spreads and thins as a relatively discrete layer (Fig. 3). determine the patterns by which effluents from river mouths Bates (1953) referred to outflows from river mouths having neglig- spread, decelerate, and deposit their sediment load. The geometries ible density contrasts as "homopycnal," whereas the term of river-mouth sediment accumulations, together with the as- "hypopycnal" was applied to buoyant effluents. In addition, Bates sociated distributions of grain size and primary sedimentary struc- distinguished a third effluent type, "hyperpycnal" outflows, in tures, reflect these effluent diffusion patterns. which the issuing water is denser than and plunges beneath the The depositional morphologies and sedimentary sequences of basin water. Field data on the last type are sparse. river-mouth systems are among the most varied of all coastal ac- cumulation forms. Multivariate analysis of 34 major deltaic sys- Dynamic Conditions tems (Wright and others, 1974) suggests the existence of a finite number of river-mouth types. In addition, there have been numer- For homopycnal effluents (that is, negligible buoyancy), the jet ous theoretical, laboratory, and field investigations of river-mouth structure depends on the ratio of the inertial to viscous forces as processes, including studies by Credner (1878), Gilbert (1884), indexed by the Reynolds number R„ at the outlet: Samoilov (1956), Bates (1953), Crickmay and Bates (1955), Axel- son (1967), Bonham-Carter and Sutherland (1963), Borichansky Ro = Uo [bo (b0l2)]l I v (1) and Mikhailov (1966), Mikhailov (1966, 1971), Jopling (1963), Takano (1954a, 1954b, 1955), Bondar (1970), Kashiwamura and where Un is the mean outlet velocity, h0 and b„ are respectively the Geological Society of America Bulletin, v. 88, p. 857-868, 8 figs., June 1977, Doc. no. 70614. 857 Downloaded from http://pubs.geoscienceworld.org/gsa/gsabulletin/article-pdf/88/6/857/3429515/i0016-7606-88-6-857.pdf by guest on 01 October 2021 INTERTIA - DOMINATED EFFLUENT(fully turbulent ¡et) in* & 6 n 0 ^ 9 (o * (b 0 -Q -0 mou ® d Uo fully turbulent & U max' ö ö ^ 6 0 effluent 3 s & & r _ â 6 a ó ^ channeîannel 2 <5 ® fi a ® ® 6 bank \ SSSSi zone of ¡ji&ii-iëiiii ^>5flow establishment?: Plan view U max Longitudinal cross section Figure 1. Spreading, diffusion, and deceleration pattern of fully turbulent axial river-mouth jet. FRICTION -DOMINATED EFFLUENT(plane turbulent ¡et with pronounced turbulent bed shear) ...¿xgggambi ënt SiSSíí^ water wï? rapid seaward deceleration Figure 2. Spreading, dif- Plan view fusion, and deceleration pattern of a plane turbulent jet with pronounced turbu- lent bed friction. mouth Uo Longitudinal cross section Downloaded from http://pubs.geoscienceworld.org/gsa/gsabulletin/article-pdf/88/6/857/3429515/i0016-7606-88-6-857.pdf by guest on 01 October 2021 SEDIMENT TRANSPORT AND DEPOSITION AT RIVER MOUTHS 859 EFFLUENTreloHv. supero« on of lighter effluent S / drives secondary flows laterally homogeneous effluent -u- (fresh water) Transverse cross section U max U max .. I approximately constant dece'erates — » F1 < 1 ^JSsältS^ ^fwedge^ intrusion Longitudinal cross section Figure 3. Spreading, mixing, deceleration, and secondary flow patterns of buoyant river-mouth effluents. depth and width of the outlet, and v is the kinematic viscosity. crease in importance as F' exceeds 1. Hayashi and Shuto (1967, Pearce (1966) found that the jet becomes fully turbulent when R0 1968) found that fully turbulent effluent diffusion occurs when F' exceeds 3,000. In controlled laboratory situations, at the mouths of equals or exceeds 16.1 very small, tranquil streams, or at the mouths of rivers which are supersaturated with suspended fine sediments (for example, the Inertia-Dominated Effluents: Turbulent Jets Hwang Ho), outflows may be viscous; however, at most large river mouths, R0> 3,000, and homopycnal outflows should be fully tur- Gilbert's (1884) classic discussion on deltaic sedimentation is bulent. based implicitly on the concept of turbulent jet diffusion. The ideal The boundary layers at most natural nonstratified river mouths model of a fully turbulent, homopycnal outflow with negligible are turbulent, and the vertical flux of momentum is dominated interference from the bottom is probably the simplest and rarest largely by Reynolds stresses. When the mouth is fronted by shallow river-mouth model. This type of effluent is normally associated water and when bed shear stress r0 is large, turbulent bed friction with steep gradient streams entering deep fresh-water lakes, exerts a pronounced influence on effluent expansion and decelera- although it may occasionally prevail initially at newly created river tion patterns. mouths along the open coast. The theory of turbulent jets is dis- In the case of hypopycnal outflows from highly stratified river cussed in detail by Albertson and others, (1950), Schlichting mouths, buoyancy inhibits turbulence. In addition, the underlying (1968), Abramovich (1963), and Stolzenbach and Harleman salt water isolates the
Details
-
File Typepdf
-
Upload Time-
-
Content LanguagesEnglish
-
Upload UserAnonymous/Not logged-in
-
File Pages12 Page
-
File Size-