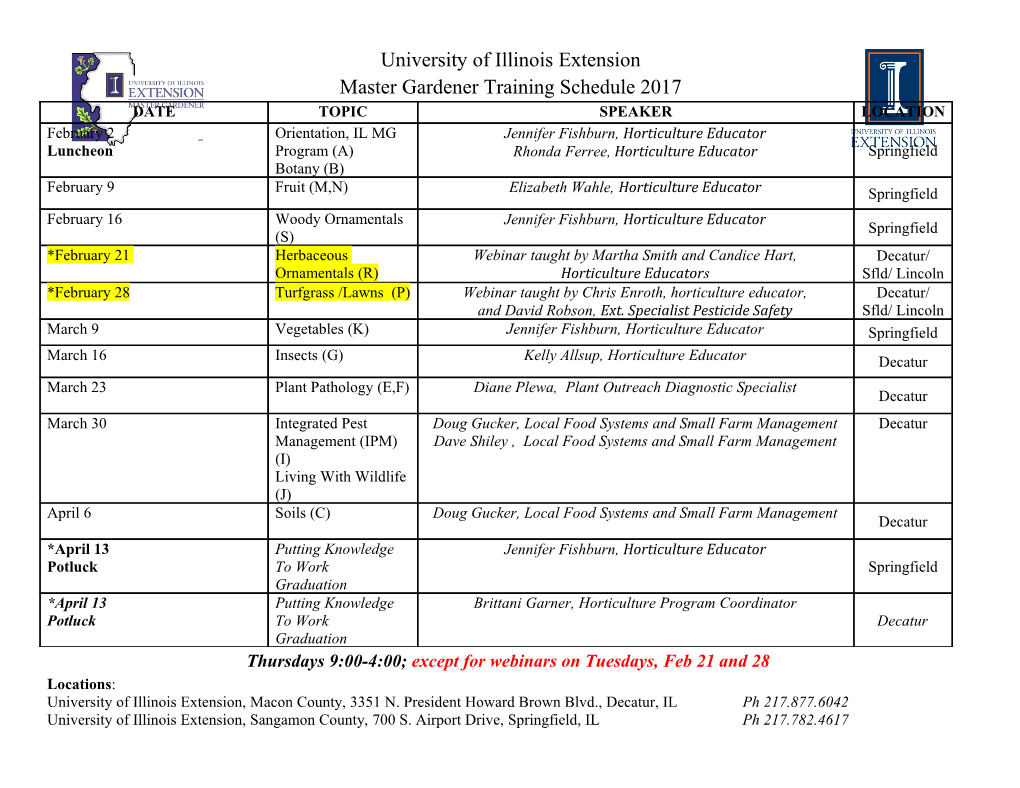
© 2010 Jong Nam Kim GENETIC AND BIOCHEMICAL ANALYSIS OF NITROGEN METABOLISM IN RUMINAL ANAEROBIC BACTERIA: A COMPARISON BETWEEN RUMINOCOCCUS ALBUS 8 AND PREVOTELLA RUMINICOLA 23 BY JONG NAM KIM DISSERTATION Submitted in partial fulfillment of the requirements for the degree of Doctor of Philosophy in Animal Sciences in the Graduate College of the University of Illinois at Urbana-Champaign, 2010 Urbana, Illinois Doctoral Committee: Professor Roderick I. Mackie, Chair Associate Professor Isaac K. O. Cann Associate Professor Bruce W. Fouke Assistant Professor Juan J. Loor ABSTRACT These studies were conducted to improve the understanding of the genetic regulation and biochemical pathways utilized by the rumen bacteria, Ruminococcus albus 8 and Prevotella ruminicola 23 during growth on, and metabolism of, different nitrogen sources. Both R. albus 8 and P. ruminicola 23 were grown using different nitrogen sources; ammonia, urea, or peptides for R. albus 8 and ammonia or peptides for P. ruminicola 23 as the sole nitrogen source. Gene expression levels and enzyme activities were analyzed by RT-qPCR, and enzyme assays, as well as microarray and proteomic analyses for P. ruminicola. The results varied according to nitrogen source and also by growth phase. For R. albus 8 growth on ammonia or urea led to the synthesis of glutamate from ammonia by the NADPH-dependent glutamate dehydrogenase (NADPH-GDH). This glutamate was then transformed to glutamine by glutamine synthetase (GS). The later transformation was especially evident during early log phase, while the further transformation from glutamate to α-ketoglutarate by the up-regulated NADH-dependent glutamate dehydrogenase (NADH-GDH) was more evident during late exponential phase. During this phase an ammonium transporter was also up-regulated, apparently as an attempt to enhance ammonia uptake from the medium as the ammonia concentration became low (< 1 mM). Three different types of GS from P. ruminicola 23 were heterologously expressed in E. coli and characterized. Two different GS type III proteins (GSIII-1; ORFB01459 and GSIII-2; ORFB02034) showed GS biosynthetic activities, while no GSI biosynthetic activity was detected. We have defined the optimal conditions for enzymatic activity and these were found to be consistent with the parameters of the rumen habitat of P. ruminicola 23. Finally, we analyzed the ii effects on transcription and assayed key enzymatic activities on limiting (0.7 mM) and non- limiting (10 mM) concentrations of ammonia during growth in chemostat culture. These studies revealed an important role for GSIII-2 (ORFB02034) in P. ruminicola 23 when ammonia was excess. Changes in the global gene expression profile of P. ruminicola 23 in response to variations in the available nitrogen source (ammonia or peptides) were analyzed by microarray and related to changes in enzymatic activity and the proteome. In total, 110 genes (3.8% of the genome) were transcriptionally upregulated during growth on ammonia, while 120 genes (4.2 % of the genome) were transcriptionally upregulated during growth on peptides. P. ruminicola 23 grown on ammonia induced genes which are predicted to be involved in amino acid biosynthesis, molecular transport and several that affect the cell envelope. Growth on peptides induced genes whose products are involved in DNA metabolism, protein fate, protein synthesis and transcription. GS-GOGAT (GSIII-2: 22.5, GOGAT large subunit: 26.3, and small subunit: 22.4 fold) pathways was also upregulated when grown on ammonia. The greatest transcriptional up- regulation was observed for the ammonium transporter, amt (47.0 fold) and the nitrogen regulatory protein PII (46.5 fold) during growth with ammonia. In contrast, growth on peptides resulted in the up-regulation of more than 17 ribosomal proteins but no up-regulation of other nitrogen metabolism pathways. Our results provide a whole genome transcriptional overview of the responses by P. ruminicola 23 to two different nitrogen sources and are supported by proteomic and biochemical evidence. Collectively leading to an overall improvement in the understanding of the genetic responses and biochemical pathways used by P. ruminicola 23 to obtain and utilize different nitrogen sources. iii The response of P. ruminicola 23 to growth-limiting or non-limiting concentrations of ammonia were analyzed by microarray and related to changes in enzymatic activity. The results demonstrated dramatic changes in gene expression and enzymatic activity between the two conditions. In total, 166 genes (5.8% of the genome) were transcriptionally upregulated during growth on non-limiting concentration of ammonia, while 287 genes (10.0% of the genome) transcriptionally upregulated during growth on limiting concentration of ammonia. Specifically, growth in non-limiting concentrations of ammonia induced genes involved in amino acid biosynthesis, while ammonia limiting conditions caused the induction of genes involved in DNA metabolism, protein fate and the manipulation of the cell envelope. Links were observed between carbohydrate and nitrogen metabolism, such as between the reverse TCA cycle and glutamate biosynthesis during growth on non-limiting concentration of ammonia. Interestingly, P. ruminicola 23 grown on ammonia showed that ammonia assimilation pathways of NADPH- GDH and GS-GOGAT are major metabolic pathways on non-limiting concentration of ammonia. Especially, GSIII-2 and the ammonium transporter were significantly up-regulated on ammonia and these gene expression results reflected the enzyme assay results. Our studies provide a more detailed understanding of the genetic and enzymatic regulatory mechanisms of nitrogen metabolism undertaken by R. albus 8, a key fiber degrading ruminal Firmicute, and P. ruminicola 23, a predominant ruminal organism important in hemicellulose and protein metabolism. iv To my beloved wife and daughter v ACKNOWLEDGEMENTS This work would not have been possible without the wisdom and guidance from my thesis advisor Dr. Roderick Mackie. I thank my advisor, Dr. Mackie, for his invaluable guidance, great ideas, great patience and his support throughout the course of my research. Dr. Mackie always provided me with everything I needed to complete my research and wonderful opportunity as a scientist. I am also grateful for the mentoring I have received from Dr. Isaac Cann provided excellent advice and expertise especially for protein purification and characterization. I am also grateful for the mentoring I have received from Dr. Bryan White. I would also like to thank my committee members, Dr. Bruce Fouke and Dr. Juan Loor for their time and interest in my work. I appreciate to my colleagues and friends from and beyond the Department of Animal Sciences for their help and friendship. Thanks to Dr. Anthony Yannarell for his guidance and support. Special thanks also to Dr. Carl Yeoman for being a good friend, for his guidance, and for scientific discussion. Other members of the lab were also greatly appreciated along the way. Thank to former graduate student Dr. Li-jung Lin and current graduate students Raymond Morales and Emily Wheeler for their help and kindness. I also thank to my friends for their support and friendship: Dr. Seongwon Seo, Dr. Dong yong Kil, and Min Ho Song. Finally, I would like to thank to my family for their support. Thanks, Jihye, for your patience and encouragement this long process with me. I want to thank my parents, parents-in- law, sister, and sisters-in-law for all their support. I would also like to thank my daughter, Yaerin, for her adorable smile, sweet kiss, and love. vi TABLE OF CONTENTS LIST OF FIGURES ...................................................................................................................... ix LIST OF TABLES ....................................................................................................................... xii CHAPTER 1. LITERATURE REVIEW .........................................................................................1 INTRODUCTION ........................................................................................................................1 DEGRADATION OF PROTEIN IN THE RUMEN ................................................................... 2 PROTEIN SYNTHESIS IN THE RUMEN ................................................................................. 6 AMMONIA AND UREA ASSIMILATION IN THE RUMEN ................................................. 6 GLUTAMATE DEHYDROGENASE ........................................................................................ 8 GLUTAMINE SYNTHETASE ................................................................................................... 9 GLUTAMATE SYNTHASE ..................................................................................................... 11 PHENOTYPIC DESCRIPTION OF THE TWO STUDY ORGANISMS: Prevotella ruminicola AND Ruminococcus albus ...................................................................................... 12 Prevotella ruminicola ................................................................................................................ 12 Ruminococcus albus................................................................................................................... 13 PROSPECTS FOR RESEARCH ............................................................................................... 14 REFERENCES .........................................................................................................................
Details
-
File Typepdf
-
Upload Time-
-
Content LanguagesEnglish
-
Upload UserAnonymous/Not logged-in
-
File Pages242 Page
-
File Size-