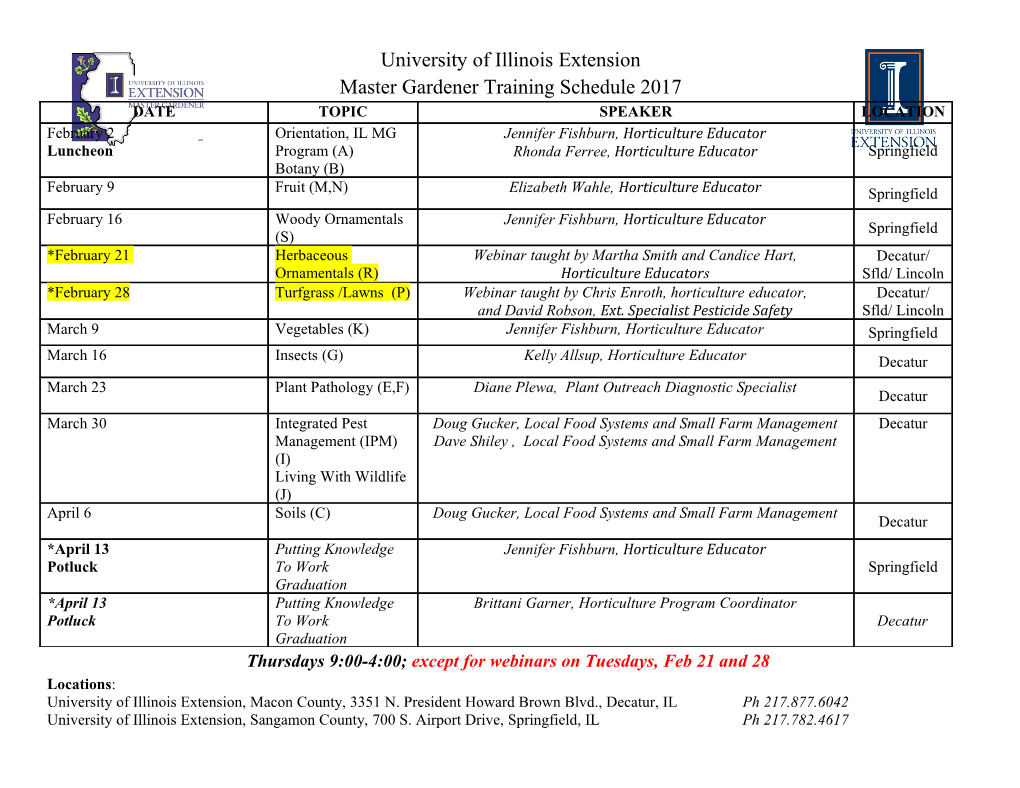
Water in the Earth’s Interior: Thermodynamics and kinetics of hydrogen incorporation in olivine and wadsleyite Von der Fakultät für Biologie, Chemie und Geowissenschaften der Universität Bayreuth Zur Erlangung der Würde eines Doktors der Naturwissenschaften -Dr.rer.nat.- genehmigte Dissertation vorgelegt von Sylvie Demouchy Aus Marseille (Frankreich) Die vorliegende Arbeite wurde von Oktober 2000 bis Juli 2004 am Bayerisches Geoinstitut der Universität Bayreuth unter Leitung von Prof. Dr. Steve Mackwell und Prof. Dr. Hans Keppler angefertigt. Vollständiger Abdruck der von der Fakultät für Biologie, Chemie und Geowissenschaften der Universität Bayreuth genehmigten Dissertation zur Erlangung des akademischen Grades eines Doktors der Naturwissenschfter (Dr. Rer. Nat.) Datum der Einreichnung der Arbeit am 01.06.2004 Datum des wissenschlaftlichen Kolloquium am 27.07. 2004 Prüfungsausschuss Vorsitzender Prof. Dr. K. Bitzer Erstgutachter Prof. Dr. S. Mackwell Zweitgutachter Prof. Dr. F. Seifert Prof. Dr. P. Morys PD. Dr. F. Langenhorst Amtierender Dakan: Prof. Dr. O. Meyer Water in the Earth’s Interior: Thermodynamics and kinetics of hydrogen incorporation in olivine and wadsleyite Von der Fakultät für Biologie, Chemie und Geowissenschaften der Universität Bayreuth Zur Erlandung der Würde eines Doktors der Naturwissenschaften -Dr.rer.nat.- genehmigte Dissertation vorgelegt von Sylvie Demouchy Aus Marseille (Frankreich) Bayreuth, 2004 Danksagung The author would like to thank Prof. Dr. S. Mackwell, Prof. Dr. H. Keppler and Prof. Dr. F. Seifert at Bayerisches Geoinstitut in Bayreuth University; Dr. Kate Wright and Andrew Walker at the Royal Institution in London (U.K.); Dr. Etienne Deloule at the CRPG in Nancy (France) and finally the european TMR Hydrospec network and an additional DFG grant for funding. Table of Contents Summary………………………………………………………………………………I Zusammenfassung…………………………………….…………………………….IV 1. Introduction ……………………………………………………………………….1 1.1 Water in the Earth’s interior: solubility and mobility ……………… ….1 1.2. Olivine ……….……………………...……..………………………..….6 1.2.1. Crystal chemistry…………...……..………………………..…..6 1.2.2. Hydrous defects…………...……..…………………………..….8 1.3. Wadsleyite…………...……..…………………………………….…..….12 1.3.1. Crystal chemistry…………...……..………………………..….13 1.3.2. Hydrous defects…………...……..………………………....….15 1.4. Aim of this thesis…………...……..……………………………….....….16 2. Experimental methods…………...……..…………………………………….….19 2.1. Starting materials and sample preparations …………...……..……..…...19 2.2 Chemical composition…………...……..……………………………..….21 2.3. High pressure apparatus…………...……..…………………………..….23 2.3.1. Piston-cylinder apparatus…………...……..……………..…....23 2.3.2. TZM rapid-quench cold-seal vessel…...……...……..……..….27 2.3.3. Multi-anvil press…………...……..………………………..….28 2.4. Infrared analysis…………...……..…………………………………..….32 2.4.1. Spectrometer and measurement parameters…………..…....….32 2.4.2. Calculation of water content using infrared spectroscopy....….35 2.5. Secondary ion mass spectrometry…………...……..………………...…38 2.6. Transmission electron microscopy...……..…………………...……..….42 3. Analysis of Diffusion…………...……..……………………………..………..….43 3.1. Theory of diffusion - Fick’s laws -……….…...……..………………….43 3.2. Diffusion in one dimension within a finite slab from an infinite source...44 3.3. Mechanisms of diffusion…………...……..……………………….....….45 3.4. Ionic diffusion in olivine…………...……..…………………..……...….48 3.5. Hydrogen diffusion in olivine…………...……..……….....……….. .….49 3.6. Hydrogen diffusion in a crystalline aggregate…………...…………...…51 4. Results…………...……..……………………………….……………………...….53 4.1. Hydrogen diffusion in olivine…………...……..……….....………..….53 4.1.1. Introduction…………...……..……………………………..….53 4.1.2. Results and discussion…………...……..…………………..….53 4.1.3. Conclusions…………...……..……………………………..….65 4.2. Hydrogen diffusion in forsterite…………...……..………………..….67 4.2.1. Introduction…………...……..……………………………..….67 4.2.2. Infrared spectra and water contents………………………...….68 4.2.3. Diffusion rate and anisotropy…….……...……..…………..….72 4.2.4. Discussion ………………………………………...…….…….75 4.2.5. Conclusions…………...……..……………………………..….79 4.3. Dehydration profiles in mantle-derived olivine ……….…...……..….81 4.3.1. Introduction…………...……..……………………………..….81 4.3.2. Geological setting and sample characterization……..……...…81 4.3.3. Infrared spectra and water contents…..………...……..……….85 4.3.4 Diffusion profiles in olivine…...……..…………………….….93 4.3.5 Mössbauer analysis………...……...……………………….….99 4.3.5. Discussion and implications for the upper mantle…..……….101 4.3.6. Conclusions…………...……..………………………..…..….105 4.4. Temperature and pressure-dependence of water solubility in iron- free wadsleyite…………...……..………………………...………..….107 4.4.1. Introduction…………...……..……………………………….107 4.4.2. Results…………...……..……………………………..…..….109 4.4.3. Discussion …………...……..……………………………..…117 4.4.4. Conclusions…………...……..……………………..……..….123 4.5. Computer simulation of hydrous defects in iron-free wadsleyite.....125 4.5.1. Introduction…………...……..…………………..………..….125 4.5.2. Modelling solids and simulation of defects…………...…..….125 4.5.3. Hydrous defects…………...……..………………………..….132 4.5.4. Results…………...……..……………………………..…..….133 4.5.5. Discussion …………...……..…………………...………..….136 4.5.6. Conclusions…………...……..………..…………………..….139 5. Conclusion…………...……..……………………..…………………...……..….141 6. References…………...……..……………………..…………………...……..….143 Appendix Appendix 1: Kröger-Vink notation used for point defects …………………Appendix-i Appendix 2: X-ray diffraction patterns for forsterite…………………..… Appendix-iii Appendix 3: TEM documentation……………………………………...… Appendix-iv Appendix 4: GULP results………………………………………………... Appendix-v Erklärung Abstract (1) Hydrogen diffusion in olivine The kinetics of hydration of dry single crystals of San Carlos olivine was determined by performing experiments under water-saturated conditions. The experiments were performed at 1.5 GPa, 1000°C for 5 hours in a piston cylinder apparatus, or at 0.2 GPa, 900°C, for 1 and 20 hours in TZM cold-seal vessels. Polarized Fourier- transform infrared spectrometry (FTIR) was employed to quantify the hydroxyl distributions in the samples after the experiments. The new data obtained show a strong anisotropy of diffusion, with the diffusion coefficient D[100] >D[010] > D[00 1] at 900°C for short duration experiments. This initial mechanism of diffusion possibly involved a redox-exchange between proton and polaron. After longer duration experiment, the anisotropy of diffusion is different with D[001 ]>D[010] ≈ D[1 00]. For this second stage of diffusion a model of hydrogen-metal vacancy associated defects is proposed, where the vacancies are the slower diffusing species with the diffusion laws: D [100], [010] = 10-(5.6±3.2) exp [-(175 ± 76)/RT] VMe D [001] = 10-(1.4±0.5) exp [-(258 ± 31)/RT] VMe (2) Hydrogen diffusion in forsterite The kinetics of hydration linked to magnesium-vacancy diffusion within dry synthetic forsterite single crystals was determined by performing similar experiments and analyses as in the previous section. The experiments were performed at 1.5 GPa, 1000°C for 3 hours in piston cylinder apparatus, or at 0.2 GPa, 900-1110°C, for 3-20 hours in TZM cold-seal vessels. The chemical diffusion coefficients are marginally slower than in iron-bearing olivine for the same diffusion process, but the anisotropy of diffusion is the same, with the [001] axis the fastest direction of diffusion and [100] the slowest. Fits of the diffusion data to an Arrhenius law yield similar activation energies for each of the crystallographic axes; a global fit to all the diffusion data gave an activation energy around 211 ± 18 kJmol-1. Thus hydration likely occurs by coupled diffusion of protons and octahedrally coordinated metal vacancies. The I diffusion rates are fast enough to modify water contents within xenoliths ascending from the mantle but they are probably too slow to permit a total equilibration in a new dry or wet environment. (3) Dehydration profiles in natural mantle-derived olivine within basalt First evidence for water diffusion in a natural mantle-derived olivine are presented from peridotite samples incorporated in basalt. The samples are olivine crystals within lherzolite xenoliths from the Quaternary alkali basalts of the Pali-Aike volcanic field in Patagonia. Water content and distribution was studied using unpolarized and polarized FTIR spectrometry and analyses shows that olivine, Cr-diopside and orthopyroxene contain a significant amount of water, with up to 13 wt ppm H2O for olivine and up to 250 wt ppm H2O in the pyroxenes. In contrast, analysis of optically clear-parts of small garnet crystals indicates that they are dry. Oriented Infrared profiles show that olivine grains larger than 0.5 mm have hydroxyl-depleted rims. These water concentration profiles suggest that partial dehydration occurred during the ascent of the xenolith-bearing magma to the Earth’s surface, confirming that dehydration is occurring in the nature. From a combination of analyses of natural xenoliths with experimental diffusion works, ascent duration of the host magma is estimated to several hours, suggesting a fast rise up to the surface. (4) Temperature and pressure dependence of water solubility in iron-free wadsleyite Previous experimental studies indicate that the maximum solubility of water in wadsleyite may vary as a function of pressure and temperature. Therefore wadsleyite samples were synthesized using a multi-anvil press. One series of experiments were performed at a fixed pressure of 15
Details
-
File Typepdf
-
Upload Time-
-
Content LanguagesEnglish
-
Upload UserAnonymous/Not logged-in
-
File Pages182 Page
-
File Size-