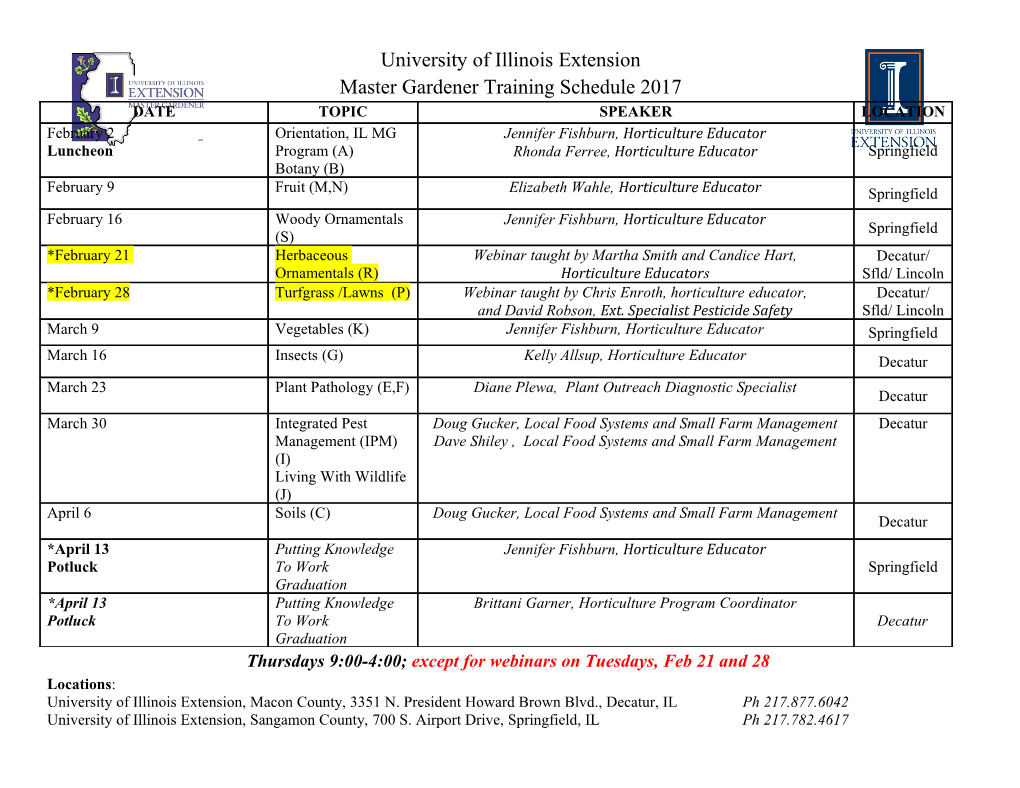
DOI: 10.1002/cbic.201500667 Reviews 1 1 2 2 3 Chemical Protein Modification through Cysteine 3 4 4 5 Smita B. Gunnoo and Annemieke Madder*[a] 5 6 6 7 7 8 8 9 9 10 10 11 11 12 12 13 13 14 14 15 15 16 16 17 17 18 18 19 19 20 20 21 21 22 22 23 23 24 24 25 25 26 26 27 27 28 28 29 29 30 30 31 31 32 32 33 33 34 34 35 35 36 36 37 37 38 38 39 39 40 40 41 41 42 42 43 43 44 44 45 45 46 46 47 47 48 48 49 49 50 50 51 51 52 52 53 53 54 54 55 55 56 56 57 57 ChemBioChem 2016, 17,2–27 2 2016 Wiley-VCH Verlag GmbH & Co. KGaA, Weinheim ÝÝ These are not the final page numbers! Reviews 1 The modification of proteins with non-protein entities is impor- for site-selective modification, including a unique nucleophilici- 1 2 tant for a wealth of applications, and methods for chemically ty, and a low natural abundance—both allowing chemo- and 2 3 modifying proteins attract considerable attention. Generally, regioselectivity. Native cysteine residues can be targeted, or 3 4 modification is desired at a single site to maintain homogenei- Cys can be introduced at a desired site in a protein by means 4 5 ty and to minimise loss of function. Though protein modifica- of reliable genetic engineering techniques. This review on 5 6 tion can be achieved by targeting some natural amino acid chemical protein modification through cysteine should appeal 6 7 side chains, this often leads to ill-defined and randomly modi- to those interested in modifying proteins for a range of appli- 7 8 fied proteins. Amongst the natural amino acids, cysteine com- cations. 8 9 bines advantageous properties contributing to its suitability 9 10 10 11 11 12 12 1. Introduction 13 modification can be promoted by exploiting the differential re- 13 14 activity of the N terminus, for example,[10] or by the use of 14 15 Our bodies are able and required to modify proteins post- enzyme recognition sequences.[11] Overall, however, the use of 15 16 translationally with a wealth of moieties both site-selectively most endogenous amino acids for modification purposes is dif- 16 17 and site-specifically for a myriad of essential processes includ- ficult to apply in a general manner due to higher levels of oc- 17 18 ing cell growth, repair and death. The ability to mimic these currence, leading to modification of multiple sites. 18 19 processes &&outside of an in vivo environment “ex vivo”?& A wealth of UAAs can be incorporated into proteins by use 19 20 & allows researchers to &&recapitulate “mimic”?&& nature, of the amber suppression methods pioneered by Schultz and 20 21 to explore biosynthetic pathways, to manipulate and to deci- Chin.[7,12,13] Such UAAs include photocaged cysteine resi- 21 22 pher natural protein function, and to attach affinity or fluores- dues.[14,15] The methodology, based on incorporating orthogo- 22 23 cent tags for imaging. Chemical protein modification contrib- nally reactive functionalities, is very elegant and a sure-fire way 23 24 utes to the enhancement of therapeutic properties,[1,2] as well to ensure reactivity at a single site. Yet it can be more time- 24 25 as in diagnostic applications in, for example, surface immobili- consuming than the use of endogenous amino acids. Alterna- 25 26 sation for biosensor design.[3] It is important not to underesti- tively, unnatural tags can be incorporated through chemoenzy- 26 27 mate the potential involved in the marrying of two or more matic methods.[9] Here, site-specific reactivity is guaranteed, 27 28 bio-macromolecular and/or small-molecule functionalities to but the use of enzymes is more resource-dependent. 28 29 form structurally and functionally diverse bioconjugates. It can be argued that modification through cysteine residues 29 30 Proteins, as an example of a class of bio-macromolecules, combines the advantages of the approaches described above. 30 31 are robust and powerful entities; however, their structure and The presence of multiple accessible free cysteine residues 31 32 function can be sensitive to general conditions employed in within a protein is likely to result in multi-site modification, but 32 33 conventional organic syntheses. These include raised tempera- cysteine has a low natural abundance, which means that selec- 33 34 tures and the use of organic solvent, two factors likely to per- tivity on a general level is more likely. In addition, cysteine resi- 34 35 turb protein structure. As well as ambient reaction conditions, dues can be readily introduced by use of facile mutagenesis 35 36 high conversion rates and minimal generation of side products techniques prior to expression, allowing control over the site 36 37 are desired, in order to avoid tedious purification steps. Modifi- of modification. The ease of incorporation and associated ex- 37 38 cation at a single site is therefore generally preferred, to avoid pression yields are specifically dependent on the protein’s 38 39 heterogeneity. structure and behaviour. Another characteristic of cysteine is 39 40 Existing methods for chemical protein modification include that it has a unique nucleophilicity, in comparison with other 40 41 the use of naturally occurring amino acids such as lysine, reactive side chains. Under physiological conditions, it has 41 42 serine, histidine and tyrosine,[4–6] and also of unnatural amino a high propensity to form the nucleophilic thiolate ion with 42 43 acids (UAAs), incorporated by use of genetically engineered a general pKa of 8.2. This, though, is highly dependent on its 43 44 bacterial strains[7,8] and/or enzymatic methods.[9] These meth- conformation and its environment in terms of buffer and 44 45 ods although useful, do have drawbacks. neighbouring residues, and can be even lower.[16,17] As well as 45 46 The use of naturally occurring amino acids does allow the a unique reactivity, cysteine also has an incredibly diverse reac- 46 47 avoidance of demanding genetic engineering. Although lysine tivity profile, as exemplified by the abundance of post-transla- 47 48 modification is commonly used and facile, the residue’s high tional modifications (PTMs) it undergoes.[18] It is able to inter- 48 49 level of occurrence in proteins means that multiple sites can convert between multiple oxidation states, often negates the 49 50 be modified in an unpredictable manner. This leads to hetero- need for co-factors in vivo, and is essential in cellular redox 50 51 geneity and/or the blocking of functional sites by the newly in- regulation. Cysteine is able to partake in exchange, radical and 51 52 troduced group. Site-specificity during endogenous amino acid atom-, electron- and hydride-transfer reactions, and also to 52 53 bind to metals.[19] Its evolutionary diversity undoubtedly pro- 53 54 [a] Dr. S. B. Gunnoo, Prof. Dr. A. Madder vides inspiration for the protein chemist developing cysteine- 54 Organic & Biomimetic Chemistry Research Group based methodology at the bench. 55 Department of Organic and Macromolecular Chemistry, Ghent University 55 56 Krijgslaan 281, 9000, Gent (Belgium) This review focuses on methodologies involving cysteine as 56 57 E-mail: [email protected] a precursor for chemical modification of proteins since 2009, 57 ChemBioChem 2016, 17,2–27 www.chembiochem.org 3 2016 Wiley-VCH Verlag GmbH & Co. KGaA, Weinheim These are not the final page numbers! ÞÞ Reviews 1 when Chalker et al. reviewed the same topic.[20] Since then, nucleophilic side chains such as lysine and histidine, and sus- 1 2 there has been exponential growth both in new cysteine- ceptibility of reagents to hydrolysis; hence the need to devel- 2 3 based chemistries and in more applied reports based on exist- op alternative methodologies as outlined in the remainder of 3 4 ing cysteine methodologies. Native chemical ligation (NCL)[21] is this review. However, despite these drawbacks, there are many 4 5 not discussed in great detail, but has been reviewed else- examples of the use of haloalkyl substituents in alkylating or 5 6 where.[22,23] Although NCL is an elegant method for the total arylating cysteine residues within proteins over the last few 6 7 synthesis of proteins (ligating peptide fragments together) and years (Scheme 1). In many cases the common ease and reliabil- 7 8 has seen many applications, our interests lie in conjugating ity of these reactions means that the need for modification op- 8 9 non-protein moieties to proteins through cysteine residues. timisation is potentially minimised, and an application can be 9 10 We have opted for a systematic review of new developments, realised in a quicker timeframe. One example is the incorpora- 10 11 classified according to the specific sulfur-based chemistry used. tion of the nitrile group into proteins by using benzyl com- 11 12 pounds with either bromo or nitrile substituents for the devel- 12 13 opment of infrared probes.[24] 13 14 14 15 2. Modification by Substitution 15 16 2.1. Alkylation through SN2 reaction with haloalkyl reagents 16 17 17 18 One of the earliest methods used for modifying cysteine resi- 18 19 dues within proteins is by substitution with haloalkyl sub- 19 strates bearing groups of interest. Iodide and bromide groups 20 [20] 20 21 in particular are commonly used. Indeed, substitution with 21 22 iodoacetamide is routinely used to “cap” cysteine residues in 22 23 protein digestion prior to analysis. Drawbacks associated with 23 24 haloalkyl substitution are potential crossreactivity with other 24 25 25 26 Smita Gunnoo is a postdoctoral re- 26 27 searcher at Ghent University in the lab- 27 28 oratory of Prof.
Details
-
File Typepdf
-
Upload Time-
-
Content LanguagesEnglish
-
Upload UserAnonymous/Not logged-in
-
File Pages26 Page
-
File Size-