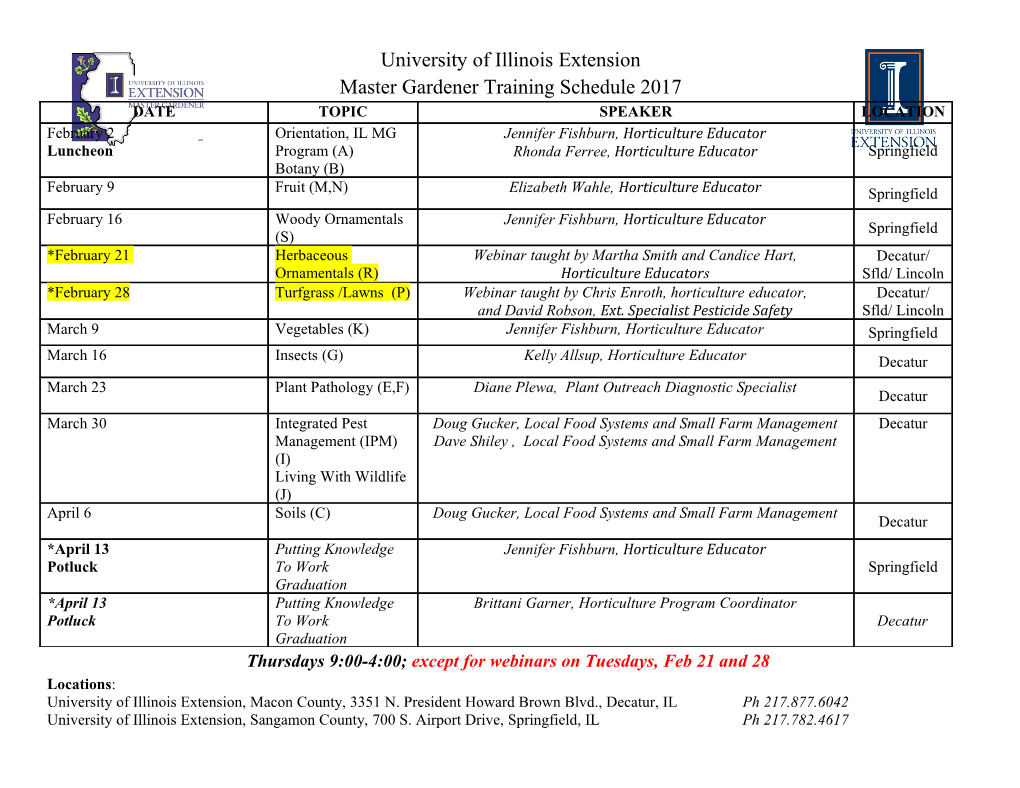
PHYSICAL REVIEW D 101, 015028 (2020) SU(5) grand unified theory with A4 modular symmetry † ‡ Francisco J. de Anda ,2,* Stephen F. King,1, and Elena Perdomo1, 1School of Physics and Astronomy, University of Southampton, SO17 1BJ Southampton, United Kingdom 2Tepatitlán’s Institute for Theoretical Studies, C.P. 47600, Jalisco, M´exico (Received 1 April 2019; published 30 January 2020) We present the first example of a grand unified theory (GUT) with a modular symmetry interpreted as a family symmetry. The theory is based on supersymmetric SUð5Þ in 6d, where the two extra dimensions are compactified on a T2=Z2 orbifold. We have shown that, if there is a finite modular symmetry, then it can i2π=3 only be A4 with an (infinite) discrete choice of moduli, where we focus on τ ¼ ω ¼ e , the unique solution with jτj¼1. The fields on the branes respect a generalized CP and flavor symmetry A4 ⋉ Z2 which is isomorphic to S4 which leads to an effective μ − τ reflection symmetry at low energies, implying maximal atmospheric mixing and maximal leptonic CP violation. We construct an explicit model along these lines with two triplet flavons in the bulk, whose vacuum alignments are determined by orbifold boundary conditions, analogous to those used for SUð5Þ breaking with doublet-triplet splitting. There are two right-handed neutrinos on the branes whose Yukawa couplings are determined by modular weights. The charged lepton and down-type quarks have diagonal and hierarchical Yukawa matrices, with quark mixing due to a hierarchical up-quark Yukawa matrix. DOI: 10.1103/PhysRevD.101.015028 I. INTRODUCTION of the discrete non-Abelian group symmetry such as A4 and S4 which may be identified as a remnant symmetry of the The flavor puzzle, the question of the origin of the three extended Poincar´e group after orbifolding. families of quarks and leptons together with their curious Some time ago it was suggested that modular symmetry, pattern of masses and mixings, remains one of the most when interpreted as a family symmetry, might help us to important unresolved problems of the Standard Model provide a possible explanation for the neutrino mass (SM). Following the discovery of neutrino mass and mixing, matrices [13,14]. Recently it has been suggested that whose origin is fundamentally unknown, there are now neutrino masses might be modular forms [15], with con- almost 30 undetermined parameters in the SM, far too many straints on the Yukawa couplings. This has led to a revival for any complete theory. The lepton sector in particular of the idea that modular symmetries are symmetries of the involves large mixing angles that suggest an explanation in extra-dimensional spacetime with Yukawa couplings deter- terms of discrete non-Abelian family symmetry [1,2]. mined by their modular weights [16]. However to date, no Furthermore, such discrete non-Abelian family symmetries attempt has been made to combine this idea with orbifold have been combined with grand unified theories (GUTs) in GUTs in order to provide a unified framework for quark order to provide a complete description of all quark and and lepton masses and mixings. lepton (including neutrino) masses and mixings [3]. In this paper we present the first example in the literature It is well known that orbifold GUTs in extra dimensions of a GUT with a modular symmetry interpreted as a family (ED) can provide an elegant explanation of GUT breaking symmetry. The theory is based on supersymmetric SUð5Þ and Higgs doublet-triplet spitting [4]. Similarly, theories in 6d, where the two extra dimensions are compactified on involving GUTs and flavor symmetries have been formulated i2π=3 a T2=Z2 orbifold, with a twist angle of ω ¼ e . Such in ED [5–12]. These EDs can help us to understand the origin constructions suggest an underlying modular A4 symmetry with a discrete choice of moduli. This is one of the main *[email protected] differences of the present paper as compared to recent † [email protected] works with modular symmetries which regard the modulus ‡ [email protected] τ as a free phenomenological parameter [15,16].We construct a detailed model along these lines where the Published by the American Physical Society under the terms of fields on the branes are assumed to respect a flavor and the Creative Commons Attribution 4.0 International license. CP A ⋉ Z Further distribution of this work must maintain attribution to generalized symmetry 4 2 which leads to an the author(s) and the published article’s title, journal citation, effective μ − τ reflection symmetry at low energies, imply- and DOI. Funded by SCOAP3. ing maximal atmospheric mixing and maximal leptonic CP 2470-0010=2020=101(1)=015028(17) 015028-1 Published by the American Physical Society DE ANDA, KING, and PERDOMO PHYS. REV. D 101, 015028 (2020) 0 violation. The model introduces two triplet flavons in the ω1 ω ab ω1 → 1 ; bulk, whose vacuum alignments are determined by orbifold 0 ¼ ω2 ω2 cd ω2 boundary conditions, analogous to those used for SU 5 ð Þ ab breaking with doublet-triplet splitting. There are also two where ∈ SLð2; ZÞð2Þ right-handed neutrinos on the branes whose Yukawa cd couplings are determined by modular weights. The charged lepton and down-type quarks have diagonal and hierarchi- or equivalently if a; b; c; d ∈ Z and ad − bc ¼ 1. These are cal Yukawa matrices, with quark mixing due to a hierar- called modular transformations and form the modular chical up-quark Yukawa matrix. group Γ [15]. Without loss of generality, the lattice vectors The remainder of the paper is organized as follows. In may be rescaled as 2 Sec. II we discuss the orbifold T =Z2 and its symmetries, as follows. In Sec. II A, we give a review of modular trans- ω1 1 1 → ¼ ; ð3Þ formations while in Sec. II B we describe how the orbifold ω2 τ ω2=ω1 T2=Z2 is only consistent with modular A4 symmetry and a choice of modulus. In Sec. II C, we explicitly show the such that the torus is equivalent to one whose periods T =Z ω ei2π=3 A orbifold 2 2 with twist angle ¼ and modular 4 are 1 and τ ¼ ω2=ω1 and we can restrict τ to the upper half- symmetry. In Sec. II D we study the remnant symmetry after plane H ¼ Imτ > 0. The modular transformations on the compactification on the T2=Z2 orbifold, while Sec. II E rescaled basis vectors which leave the lattice invariant are connects this remnant symmetry and the modular symmetry. given by1 In Sec. II F we discuss the enhanced A4 ⋉ Z2 on the branes. In Sec. III, we present the field content of the SUð5Þ GUT 1 1 ω0 aτ b → ; τ0 2 þ : with A4 modular symmetry and a Uð1Þ shaping symmetry, where ¼ ¼ ð4Þ τ τ0 ω0 cτ þ d including the Yukawa sector and the specific structure for the 1 effective alignments that the modular symmetry can gen- A SLð2; ZÞ transformation on the modulus parameter τ and erate, resulting in the low energy form of the SM fermion its negative are equivalent, as can be seen from Eqs. (2) and mass matrices which we show can lead to a very good fit to (4). Therefore, we can use the infinite discrete group the observables. Finally in Sec. IV we present our con- PSLð2; ZÞ¼SLð2; ZÞ=Z2, generated by clusions. In order to make the paper self-contained, some necessary background information is included in the S∶ τ → −1=τ T∶ τ → τ 1; Appendixes. In Appendix A we show the explicit proof and þ ð5Þ that only the A4 modular symmetry is consistent with the branes, with specific choices of modulus. We supplement to describe the transformations that relate equivalent Γ¯ the general A4 group theory in Appendix B, the consistency tori. This is also called the modular group satisfying Γ¯ Γ= 1 2 conditions for generalized CP symmetry consistent with A4 ¼ f g. The generators of the infinite-dimensional in Appendix C and the general theory for modular forms in modular group can also be written as Appendix D. Finally we show sample fits of the observed data in Appendix E. 01 10 S ¼ ;T¼ : ð6Þ −10 11 II. ORBIFOLDING AND SYMMETRIES They satisfy the presentation A. Review of modular transformations In this subsection we present the general theory of modu- Γ¯ ≃ fS; TjS2 ¼ðSTÞ3 ¼ Ig=f1g; ð7Þ lar transformations. The structure of the extra-dimensional torus is defined by the structure of the lattice by where S; T ∈ SLð2; ZÞ. We will be considering the finite-dimensional discrete M z ¼ z þ ω1;z¼ z þ ω2; ð1Þ subgroups by imposing an additional constraint on T , where M is a positive integer, where ω1;2 are the lattice basis vectors. The variable z z x ix x ¯ 2 3 M refers to the complex coordinate ¼ 5 þ 6, where 5 ΓM ≃ fS; TjS ¼ðSTÞ ¼ T ¼ Ig=f1g; ð8Þ and x6 are the two extra-dimension coordinates. The torus is then characterized by the complex plane C modulo Λ Λ 1Where we have relabeled the integers a → d, b → c, c → b, a two-dimensional lattice ðω1;ω2Þ, where ðω1;ω2Þ ¼ d → a to be consistent with conventional notation. fmω1 þ nω2;m;n∈ Zg, i.e., T2 ¼ C=Λ ω ;ω . The lattice 2 ð 1 2Þ The modular group Γ refers to SLð2; ZÞ, while Γ¯ is used for is left invariant under a change in lattice basis vectors PSLð2; ZÞ and takes into account the equivalence of an SLð2; ZÞ described by the general transformations matrix and its negative.
Details
-
File Typepdf
-
Upload Time-
-
Content LanguagesEnglish
-
Upload UserAnonymous/Not logged-in
-
File Pages17 Page
-
File Size-