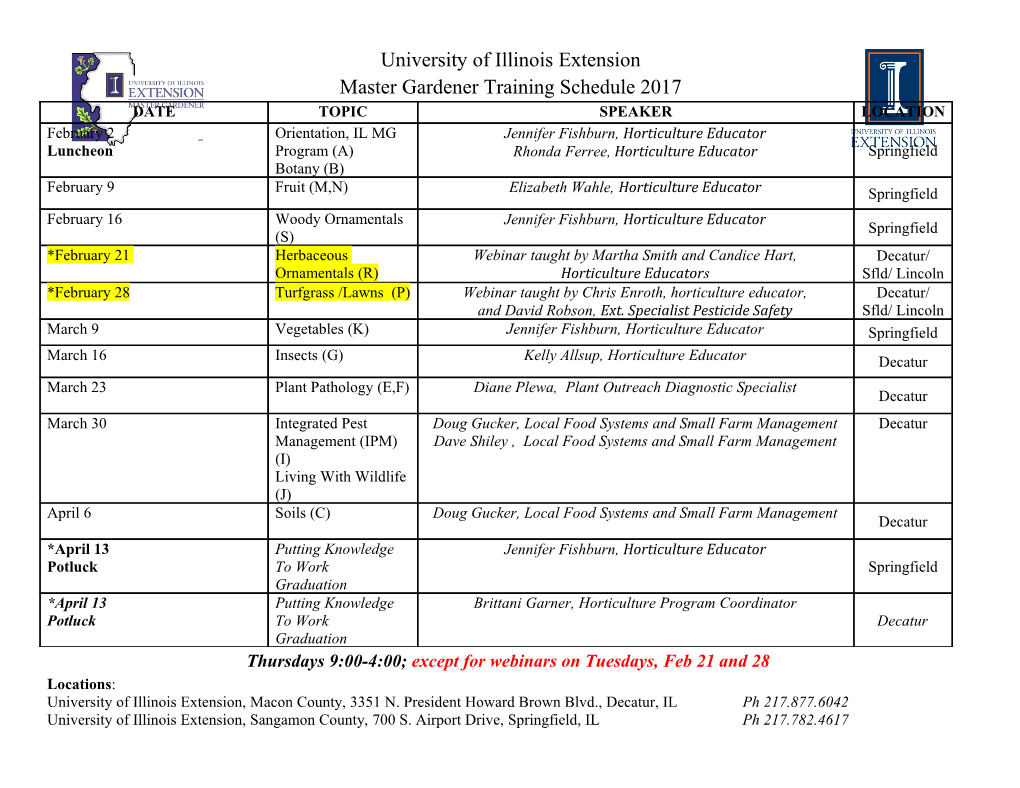
White Paper The Use of Robust Biomarkers in the Early Clinical Development of N-methyl-D-aspartate (NMDA) Receptor Antagonist Biotrial has extensive early clinical development experience with a high output of over 20 CNS studies per year. The experience and capabilities of Biotrial Neuroscience (now also incorporating the know-how of the legacy CNS CRO Forenap), enables the in-depth cognitive and neurophysiological assessment of NMDA antagonists. The aim of the present document is twofold. Firstly, to characterize the pharmacological effects of non-competitive NMDA antagonists in psychiatry and pain indications and to validate the use of ketamine as a positive control in the development of these compounds with this mechanism of action. Secondly, to present Biotrial’s strategy and rationale for the integration of surrogate endpoints, biomarkers and PD models into a Phase I program, in order to establish early proof of concept of new NMDA antagonist candidates. Pharmacological Effects of Non-Competitive NMDA Antagonists: The Example of Ketamine Glutamatergic antagonists, such as ketamine, acting at the NMDA open channel have a long history of use in neuropsychiatry – independently of their anesthetic or pain attenuating properties. The mechanism of action and pharmacological effects of ketamine have been extensively studied over the last 20 years. Ketamine was initially profiled as a reversible model of psychosis1, producing positive and negative symptoms of schizophrenia as well as cognitive impairment. As of today there are approximately one hundred publications on the symptoms, brain connectivity, imaging and cognitive function alterations induced by ketamine. The main hypothesis to explain the effect of ketamine was the reduction of the glutamatergic burst in the prefrontal cortex, secondarily enhancing AMPA α-(amino- 3-hydroxy-5-methyl-4-isoxazolepropionic acid receptor) signaling. The mammalian rapamycin (mTOR) signaling pathway and its subsequent release of brain-derived neurotrophic factor (BDNF), possibly allowing the formation of new synapses, has also been reported as a potential target. Lastly, evidence shows activation of the early activity gene (Arc), which is known to down-regulate AMPA receptors. AMPA receptors are, in turn, part of oscillation-forming thalamo-cortical loops2,3, which may partially explain the increased effects on qEEG signals observed in animals4. Psychiatry Applications More recently, reports of long-lasting improvements of depressive symptoms with ketamine5 have led to the development of related compounds with less of the psychotomimetic effects and the assumed antidepressant effect based on animal data, such as AZD6765 (Lanicemine), a low-trapping NMDA receptor antagonist6,7. Comparable effects should be achieved with NR2B antagonists (traxoprodil and MK-0657) or with mGluR2 and mGluR5 Negative Allosteric Modulators8. A recent meta-analysis of 5 studies which assessed the effects of ketamine in Major Depressive Disorders (MDD) in 134 depressed patients9, showed that ketamine was still effective 7 days post dose and that the data across the 5 studies were homogenous (Fig. 1). This is a first step in validating the use of ketamine in Phase I development as a positive control for NMDA activity. Figure 1: Forests plots from Lee et al. 2015 on Day 1 (left panel) and Day 7 (right panel). Numerous animal models have been used which showed the rapid antidepressant effect of ketamine10. Interestingly, the effect of ketamine was blocked by pre-treatment with rapamycine, thus confirming the involvement mTOR signaling in the antidepressant effect of ketamine. 2 Pain Applications Apart from its use in psychiatry, ketamine as an NMDA antagonist prototype has been tested and used in several pain therapeutic protocols. It is used as an add-on to opioids or as a sparing agent in cancer pain or acute pain11,12. Ketamine also reduces morphine-induced hyperalgesia, through a mechanism mediated by the phosphorylation of the NMDA receptor linked to the activation of Protein Kinase C13,14. Many trials have also been conducted with dextromethorphan or memantine, however ketamine seems the most efficacious. NMDA antagonists also have the potential to provide release from neuropathic or orofacial pain, especially combined with opioids15,16 2003) even though clinical data is currently limited17. Biomarkers and Surrogate Endpoints of NMDA Antagonist Activity in Non-Clinical and Early Clinical Development By observing the effects of NMDA antagonist activity, as outlined above, it is possible to identify various biomarkers that could be of use, prior to planning a Phase IIb trial, in order to measure target engagement. Methods exist for psychiatry and pain applications, several of which are translational (T): • High amplitude spontaneous gamma oscillations and increased connectivity (T) • Mismatch Negativity (MMN) reduction (T), reduction of the P3a and P3b components in animals and of the P300 in humans (T) • Increase in neurotrophic factor BDNF plasma levels (T) • Clinical assessment of psychotomimetic effects (both negative and positive symptoms) and deficit in working memory • Shift of emotional processing with Facial Emotion Recognition Task (FERT) • Bold imaging of various regions in resting state • Wind-up, i.e. temporal summation of stimuli leading to pain in normal or sensitized skin (T) • Reversal of acutely induced remifentanil hyperalgesia (T) • Induced gamma produced either by auditory stimuli or visual stimuli. Both are up and running and have undergone a validation process inclusive of test-retest reliability (see Fig. 5) • Thermal grill illusion Biotrial has the ability to measure all of these biomarkers and is therefore perfectly positioned to assist clients with the early development of NMDA antagonist compounds. These models are up and running and some are compatible with the single and multiple dose study design combined with an add-on arm. 3 The Use of Ketamine as Positive Control in the Development of NMDA Antagonists The reference drug ketamine has been extensively studied and a dosing regimen of a bolus + infusion was tested for its cognitive, psychometric and electrophysiological effects (Fig. 2). Figure 2: Clinical data (Brief Psychiatric Rating Scale total and Scale for Assessment of Negative Symptoms) in 12 male subjects in a 4-way crossover study conducted at Forenap. An application of this was the use of ketamine as a positive control in the development of lanicemine, as shown in Fig. 3, where the dissociative symptoms observed with ketamine were not observed with Lanicemine. Figure 3: Effects of ketamine and lanicemine on Clinician Administered Dissociative State Scale (from Sanacora7 et al. 2015) conducted at Forenap. Similarly, when qEEG was used in a study with lanicemine which also used ketamine as a positive control, the first step was to test the lack of nystagmus (involuntary eye movement) at the dose used, as nystagmus artificially creates gamma oscillations18. In this study19 there was no effect on optokinetic parameters and a dose-dependent increase in the gamma-1 and gamma-2 bands was observed. The magnitude of the ketamine effect can be achieved in the range between doses of 75 mg and 150 mg of lanicemine (Fig. 4). Induced gamma is also of relevance and a paradigm was set up and tested for its reproducibility (Fig. 5). In addition, Lanicemine was tested in MDD patients at a single dose of 100 mg, which induced a reduction in the blood oxygen level-dependent (BOLD) 4 signaling in the subgenual cingulate gyrus (NB subgenual cingulate and visual cortical responses to sad, but not happy, facial stimuli are correlated with changes in symptom score) and reduction of negatively valenced stimuli (angry face pictures) induced activation of the amygdala20 as an acute PD endpoint. Figure 4: Effects in healthy subjects of ketamine and lanicemine19. When tested in efficacy trials, dosing three times a week produced an effect7 while a single infusion of lanicemine failed to produce an antidepressant effect21. The sponsor made the strategic decision to halt CNS activities, which included halting the compound’s development, despite a promising profile. Figure 5: Method validation for evoked gamma oscillation and resting gamma oscillation in healthy subjects. 5 In another study the effect of ketamine on mismatch negativity, a ketamine-sensitive biomarker, was in part reversed by glycine 0.8 mg/kg, which binds at the co-agonist site19 (Fig. 6). Figure 6: Partial reversal of the effects of ketamine on mismatch negativity by oral glycine in the healthy male subject. Integrating Biomarkers and PD Models into Phase I Programs In proposing a strategy for an early development program for an NMDA antagonist, we would recommend the use of adaptive CNS methodologies to investigate target engagement as well as pharmacological and clinical activity, in addition to standard safety, tolerability and pharmacokinetic assessments. When and where possible, these methods would be included in the Phase I Single (SAD) and Multiple (MAD) dose studies. However, for certain tests such as pain models or advanced CNS methods, SAD and MAD designs may not provide enough sensitivity or allow for comparison with ketamine. In such cases we would recommend the inclusion of an additional arm in an integrated protocol. A list of possibilities (non-exhaustive), per study type and indication, would be: First-in-Human Studies • Use of qEEG on a limited number of electrodes in the SAD study in an incomplete block design with
Details
-
File Typepdf
-
Upload Time-
-
Content LanguagesEnglish
-
Upload UserAnonymous/Not logged-in
-
File Pages9 Page
-
File Size-