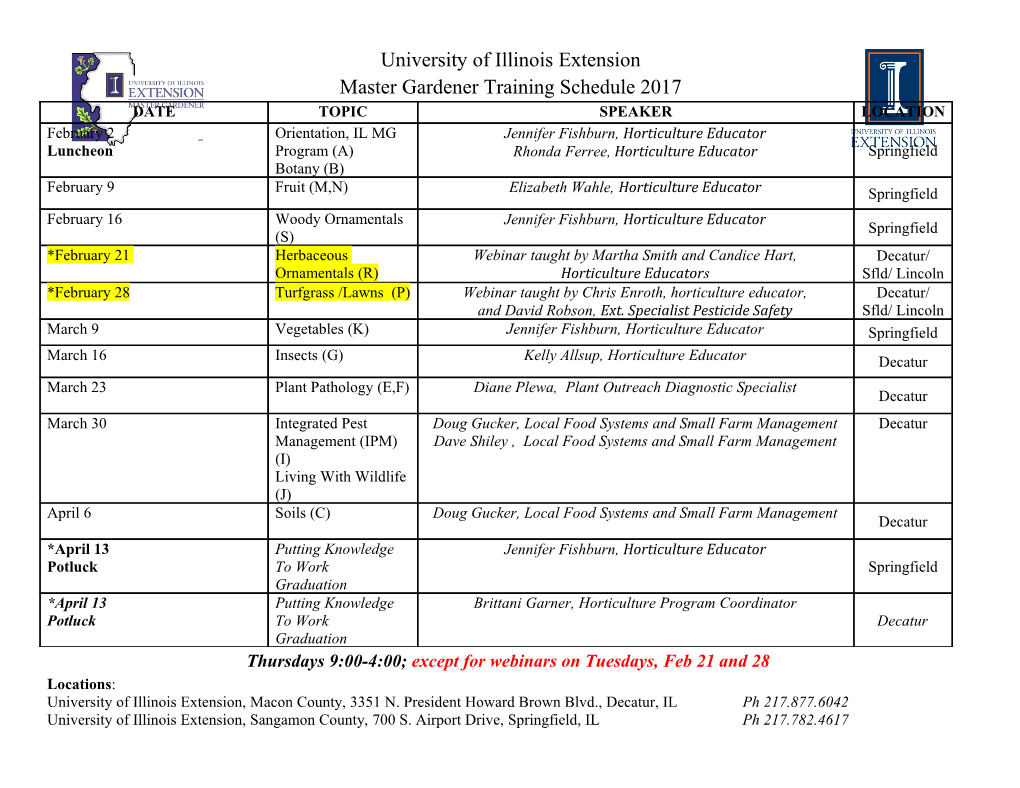
Deep Space Navigation NASA Technology Roadmaps Review Robotics, Communications, and Navigation Workshop 29 March 2011 Lincoln J. Wood Jet Propulsion Laboratory, California Institute of Technology Topics to be Covered • Information about speaker • General comments on roadmap • Comments on roadmap Section 2.1.4 • Radio metric tracking technologies • Frequency and timing technologies • Comments on roadmap section 2.1.6 • Communications technologies (brief overview) LJW - 2 Information about Speaker • Education – B.S., Cornell University, Engineering Physics – M.S., Ph.D., Stanford University, Aeronautics and Astronautics • With Jet Propulsion Laboratory, Caltech, since 1977 – Various program management, line management, and technical analysis responsibilities in space navigation and mission design – Currently, Principal Engineer, Mission Design & Navigation Section • Associate Editor of – Journal of Guidance, Control, and Dynamics, 1983-1990 – Journal of the Astronautical Sciences, 1980-1983 • Technical committee member – American Astronautical Society (AAS) Space Flight Mechanics Committee, 1980-1997; Chairman, 1993-1995 – AIAA Astrodynamics Technical Committee, 1985-1988; Chairman, 1986-1988 • Associate Fellow, AIAA; Senior Member, AAS and IEEE LJW - 3 Information about Speaker (Cont’d) • Author or coauthor of 70+ journal articles or conference papers on space navigation, trajectory optimization, or control theory • Pertinent recent publications include – Wood, L. J., “Interplanetary Navigation,” Encyclopedia of Aerospace Engineering, Vol. 5, edited by R. Blockley and W. Shyy, John Wiley & Sons, Ltd., Chichester, UK, 2010, pp. 3071-3084. – Wood, L. J., “The Evolution of Deep Space Navigation: 1989-1999,” in Advances in the Astronautical Sciences: The F. Landis Markley Astronautics Symposium, Vol. 132, edited by J. L. Crassidis, et al., Univelt, San Diego, 2008, pp. 877-898. – Wood, L. J., “The Evolution of Deep Space Navigation: 1962-1989,” in Advances in the Astronautical Sciences: Guidance and Control 2008, Vol. 131, edited by M. E. Drews and R. D. Culp, Univelt, San Diego, 2008, pp. 285-308. LJW - 4 General Comments on Draft Communication and Navigation Systems Roadmap • Section 2.1.4 touches, at least briefly, on many important topics within positioning, navigation, and timing – A few important topics are not addressed (see also presentations by MiMi Aung and Al Cangahuala) – A few statements made are not correct (see subsequent slides) • Section 2.1.6 discusses several revolutionary concepts – Comments with regard to practicality and breadth of applicability of X- ray and neutrino-based navigation are made later in this package LJW - 5 Comments on Section 2.1.4 • Text as written (p. 15, ¶2): “Position determination performance is better than 10m at near-Earth distances, and is 10s of km at the distance of Mars.” • Comments on text: – Position determination performance is typically a few km on approach to Mars, using only line-of-sight (Doppler and range) data, with variations due to mission geometry and nongravitational forces acting on spacecraft – Once in orbit around Mars, orbit determination accuracies are typically better – Broad generalizations about conventional radio metric orbit determination accuracies are difficult to make because of indirect nature of deducing three- dimensional position and velocity from line-of-sight measurements LJW - 6 Comments on Section 2.1.4 (Cont’d) • Text as written (p. 15, ¶2): “The Deep Space Network (DSN) employs a high- accuracy Very Long Base Line (VLBI) method that yields position determination performance of 1km at Mars, a few kilometers at Jupiter, and 100s of km at distances beyond Jupiter.” • Comments on text: – VLBI denotes Very Long Baseline Interferometry; particular form of VLBI of interest (figure below) is called Delta-Differential One-Way Ranging (ΔDOR) – ΔDOR can yield position determination performance as good as 250 m at Mars (Mars Exploration Rover results), a few kilometers at Jupiter, and errors that increase linearly with distance beyond Jupiter, but only when used in conjunction with Doppler and ranging data – ΔDOR data are very useful augmentation to Doppler and range data, but are not replacement for these ay el d ft ra ec ac sp Correlator Baseline B Bcos()/c LJW - 7 Comments on Section 2.1.4 (Cont’d) • Text as written (p. 15, ¶1): “NASA’s current PNT state-of-the-art relies on both ground-based and space-based radiometric tracking, laser ranging, and optical navigation techniques (e.g. star trackers, target imaging).” • Comment on text: – Optical navigation uses science imaging systems rather than star trackers, because of superior angular resolution and other characteristics of former – In addition, dedicated optical navigation camera was flown on Mars Reconnaissance Orbiter as technology demonstration on approach to Mars • Mass of 2.8 kg • 24 µrad pixel size, 1024x1024 pixels • Gimbal (plus electronics and bracket) would increase mass by 2.2 kg LJW - 8 Comments on Section 2.1.4 (Cont’d) • Text as written (p. 15, ¶2): “Optical navigation methods yield position determination performance of 1 km at near-Earth distance and 10s of km at Mars distance.” • Comment on text: – Accuracy of optical navigation data depends on distance from target body being imaged, not on distance from Earth – Except when viewing natural body subtending many pixels, optical navigation accuracy is better characterized in imaging system-dependent angular terms (e.g., 2-5 µrad) than in km LJW - 9 Tracking Accuracy Improvement over Time • Ability to determine spacecraft position from DSN radio metric data has improved many orders of magnitude over 50 years, due to major capability additions shown and many incremental improvements • Far-sighted technology investments were needed to achieve this • Technology investments are needed to ensure continued success in future LJW - 10 Ongoing Radio Metric Tracking Improvements • Range: Calibration improvements and hardware upgrades (transponders) can be used to push accuracy down to ~10 cm for missions such as BepiColombo (needed for relativity and solid core gravity mapping investigations) – not funded yet • ΔDOR: Steady improvements in bandpass separation and width and nonlinear dispersion calibration, recording bit rates, and quasar catalog density and accuracy have reduced absolute angular accuracy to ~200 m on impact plane at Mars, as of today • Phase Tracking: R&D support for Phoenix using Very Long Baseline Array (VLBA) obtained absolute (quasar-relative) angular accuracies of ~150 m on impact plane at Mars (after the fact) • Relative Tracking: Using VLBA phase tracking, Phoenix was tracked with respect to Mars orbiters, 90 days prior to arrival, with accuracies of ~20 m in R&D mode; DSN may be able to provide similar S/C-relative tracking 60 days prior to Mars arrival, with typical ΔDOR turnaround times of hours, rather than months – experiments are in preparation • Further information on radio metric tracking technologies may be obtained from [email protected] LJW - 11 Frequency and Timing Technologies – General Comments on Roadmap • Section 1.4 does good job of capturing “Top Technical Challenges” categories • Avoid communication & navigation becoming constraints to missions (1.4.1-2) – Major TRL 4-7 advances are needed to make system building blocks available to PNT architecture designs • Operable frequency standards/clocks for use in space are particularly needed – Lower TRL component technology pipeline should be supported at appropriate level • Minimize latency impact (1.4.3) – Need new level of PNT system autonomy – Need accurate/stable & reliable frequency standards in space environment • Minimize size, weight, and power (SWAP) and improve performance (1.4.4) – Availability of pipeline of space qualified parts/components is critically important – SWAP advances are technologies in themselves • Provide integrity of information delivery across solar system (1.4.5) – Frequency & timing autonomy results from high clock accuracy/stability plus reliability – Lesser clock accuracy/stability encounters limits imposed by remote time/frequency transfer • Lower life cycle cost of services (1.4.6) – Focus on standardized, long-term PNT system infrastructure (relays, satellite constellations at moon or Mars, beacons, etc.) – Timescales extended through solar system; distributed timing nodes • Validate with flight missions (1.4.7) – Need well designed validation of family of oscillators/frequency standards/time transfer LJW - 12 Frequency and Timing Technologies – Responses to Questions Sent to Presenters • What are top technical challenges? – Advance TRL of space frequency standards/clocks to flight (stability, SWAP, & reliability) • Latency to deep space means clock accuracy/stability must stand alone – Atomic timekeeping is currently not performed beyond Earth orbit (GPS) • With less stable oscillators, must rely on remote frequency/time transfer technologies – Limits imposed by transmission media: fiber (local), atmosphere, space environment – Limits imposed by distance – SNR improves with higher transmission frequency – Credible system infrastructure designs/architectures – realistic, meaningful space requirements are needed – Broad component advances and availability are needed for oscillators/USOs, space qualified lasers, packaging • E.g., laser-cooled microwave or optical clock technology is not feasible for space until qualified lasers exist • What technology gaps does roadmap not cover? – Communication, navigation,
Details
-
File Typepdf
-
Upload Time-
-
Content LanguagesEnglish
-
Upload UserAnonymous/Not logged-in
-
File Pages19 Page
-
File Size-