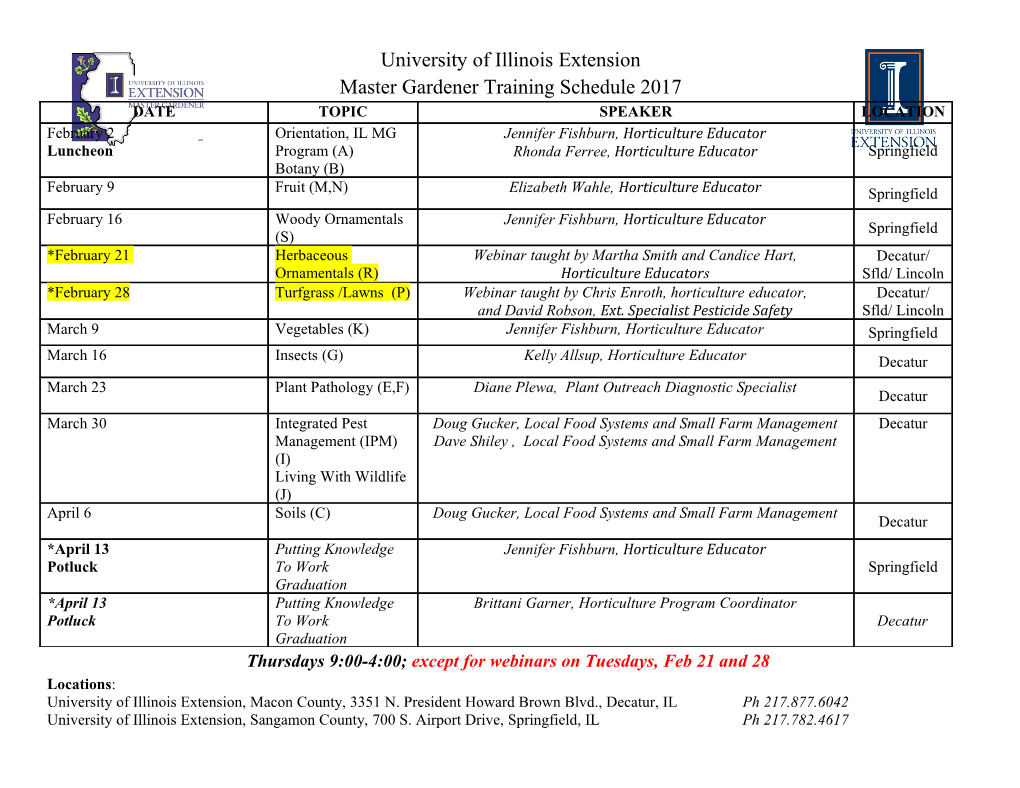
POST-TREATMENT HORIZONTAL HYDRAULIC FRACTURE MODELING WITH INTEGRATED CHEMICAL TRACER ANALYSIS, A CASE STUDY by Angela K. Dang c Copyright by Angela K. Dang, 2016 All Rights Reserved A thesis submitted to the Faculty and the Board of Trustees of the Colorado School of Mines in partial fulfillment of the requirements for the degree of Master of Science (Petroleum Engineering). Golden, Colorado Date Signed: Angela K. Dang Signed: Dr. Xiaolong Yin Thesis Advisor Golden, Colorado Date Signed: Dr. Erdal Ozkan Professor and Head Department of Petroleum Engineering ii ABSTRACT As early as the 1950s, the need to track water in various applications, including stream water, sanitary systems, ground water, and oil field water flooding, resulted in the develop- ment of chemical tracer technologies. While the utilization of tracer technologies to track water during reservoir development is mature, the use of chemical tracers in unconventional hydraulic fracturing operations is relatively new. With the increasing use of chemical trac- ers in unconventional settings, questions related to proper execution, interpretation, and integration of chemical tracer tests arise. The first part of this research discusses the design and analysis of a tracer study conducted in a section of the Wattenberg Field, Colorado. The results of the tracer study show that the preferential direction of cross-well tracer flow during hydraulic fracturing reflected the order of completion rather than the geology. However, large geologic features, such as a faulted graben, can facilitate cross-well transport of water-based chemical tracers during fracturing. Minor faults between wells can facilitate cross-well transport of oil-based chemical tracers during production. Furthermore, the percentage of recovered tracers from different intervals along the wellbore varies, which can reflect the contribution of that interval to the net production. Based on the analysis, suggestions on how to conduct a tracer study to obtain high-quality data are provided. The second part of this research shows how the results of the tracer study can be inte- grated into a post-treatment hydraulic fracturing model to evaluate production efficiency. Using the fractional production flowback results from the chemical tracer study, hydraulic fractures were calibrated to match the fractional production from each interval. The fracture model provides insight into what the hydraulic fractures look like, potential communication avenues for the chemical tracers, and production efficiency. iii TABLE OF CONTENTS ABSTRACT ......................................... iii LISTOFFIGURES ..................................... vi LISTOFTABLES ..................................... viii LISTOFSYMBOLS..................................... ix LISTOFABBREVIATIONS ................................ xi ACKNOWLEDGMENTS ................................. xiii CHAPTER1 INTRODUCTION ...............................1 1.1 DevelopmentHistory ............................... .2 1.2 ChemicalTracerData .............................. .7 1.3 HydraulicFractureModeling. .....9 1.4 Summary ......................................12 CHAPTER 2 EXTENT OF HYDRAULIC COMMUNICATION . 15 2.1 Introduction.................................... 15 2.2 TracerStudyDesignandDataCollection . ....... 16 2.3 AnalysisMethods.................................. 19 2.3.1 MassPercentCalculations . 19 2.3.2 CommunicationIndex . 21 2.4 Results........................................22 2.4.1 FlowPreference............................... 24 2.4.2 FlowbackFraction ............................. 26 iv 2.4.3 Communicationv.Distance . 29 2.4.4 Communicationv. InjectionLocation . ..... 30 2.5 Summary ......................................35 CHAPTER 3 MECHANISM OF HYDRAULIC COMMUNICATION . 40 3.1 Introduction.................................... 40 3.2 Hydraulic Fracture Modeling Fundamentals . ....... 40 3.3 LogProcessingandGridSetup . 44 3.4 HydraulicFractureModeling. .... 47 3.4.1 Post-TreatmentHistoryMatching . 51 3.4.2 FractureGeometryAnalysis . 55 3.4.3 FractureEfficiency ............................. 56 3.5 Summary ......................................60 CHAPTER 4 CONCLUSIONS AND RECOMMENDATIONS . 61 REFERENCESCITED ................................... 64 APPENDIX - MASS PERCENT CALCULATIONS . 67 v LIST OF FIGURES Figure1.1 TheWattenbergField. ....3 Figure 1.2 A generic stratigraphic column for the Wattenberg Field...........4 Figure 1.3 Cross-sectional view of the horizontal well spacing in the study section . 6 Figure1.4 Anoverviewofthetracertypes . ......8 Figure 1.5 Schematic illustrating the five definitions of fracture half-length . 11 Figure 1.6 Schematic of the tracer study design with seismic overlay . 13 Figure 2.1 Schematic of the water-based chemical tracer studydesign . 17 Figure 2.2 Schematic of the oil-based chemical tracer study design .......... 18 Figure 2.3 An example of raw data from a chemical tracer study . .........20 Figure 2.4 Proposed mechanism for flow preference. .........22 Figure2.5 Averagebreakdownpressures. ...... 24 Figure2.6 30-dayaveragecasingpressure . ........25 Figure 2.7 Mass percent recovered v. distance (CFT 1500) . .........31 Figure 2.8 Mass percent recovered v. distance (CFT 1700) . .........32 Figure 2.9 Mass percent recovered v. distance (CFT 1900) . .........33 Figure 2.10 Mass percent recovered v. distance (CFT 2500) . ..........34 Figure 2.11 Mass percent recovered v. distance by injection location. 36 Figure 2.12 Tracer concentration decline for 1N, 5C, 9N and 11N ...........37 Figure 2.13 Tracer concentration decline, Eagle Ford v. Bakken ............38 Figure 3.1 Lateral grid showing the base model of the total closure stress (psi) . 45 vi Figure3.2 Determiningtotalstress,modelv. DFIT . ........46 Figure 3.3 Lateral grid showing the base model for the brittlenessfactor . 48 Figure 3.4 Lateral grid showing the base model for the total closure stress (2N) . 49 Figure 3.5 Lateral grid showing the base model for the total closure stress (6N) . 50 Figure 3.6 Transverse grid showing the absence of stress shadowingeffects . 51 Figure 3.7 Treating Pressure (psi) history match for a single stageinwell2N . 52 Figure 3.8 Net pressure (psi) extends beyond the vicinity of thewellbore. 54 Figure 3.9 The two types of fracture designs for 2N and 6N . .........56 Figure 3.10 Lateral grid showing the brittleness factor . ..............59 Figure A.1 Schematic of a wellbore with a multiple tracer design. ...........67 vii LIST OF TABLES Table 2.1 Summary of Well-to-Well Interactions . ...........23 Table 2.2 Average Concentration of OFT Recovered in NeighboringWells. 26 Table 2.3 CFT Flowback Fraction from Specified Intervals . ..........27 Table 2.4 Approximate Mass of CFT Injected and Recovered . .........27 Table 2.5 OFT Flowback Fraction from Specified Intervals . ..........28 Table 2.6 Percentage Contribution per Interval per Stage . ..............29 Table2.7 ListofNon-DuplicatedCFTs . ..... 35 Table3.1 FractureTypesandLengthsfor2N . ....... 53 Table3.2 FractureTypesandLengthsfor6N . ....... 53 Table 3.3 Fractional Flow Contribution at 100% Efficiency, TFFL = 525ft . 57 Table 3.4 Calibrated Fractional Flow Contribution, TFFL = 257 ft ..........57 Table3.5 CalculatedFractureEfficiency . ........58 viii LIST OF SYMBOLS actualnumberofstagesinintervali . ....... nact,i bottomholeinitialshut-inpressure . ....... Pbh,ISIP brittlenessfactor .................................. ... BRIT closurepressure(psi)............................... ...... Pc communicationindex................................. .... CI concentrationoftraceri . ...... wi out concentration of tracer in flowback from interval i . ............ Ci in concentration of tracer injected into interval i . ............. Ci cumulativeproductionofwater(w)andoil(o) . ........ Vw/Vo densityofwater..................................... ... ρw effective flowing [hydraulic] fracture length for interval i .............. EFFLi effectiveporosity ................................... .... φE fractionalflowcontributionforintervali . ............ fi hydraulicfracturehalf-length . ......... xf massoftracer....................................... m in/out mass of tracer injected into (in)/produced from (out) intervali........... mi R masspercentrecoveredfromintervali . ........ mi netpressure........................................ Pnet numberofflowingstagesinintervali . ....... nf,i overburdenpressure(psi) . ...... Pob ix Poisson’sratio ..................................... .... ν Poisson’sratioforBRFcalculation . ...... PRBRIT Poisson’sratio,composite . ..... PRC porepressure(psi) .................................. .... Pp regional horizontal strain (microstrain) . ............. ǫx regional horizontal tectonic stress (psi) . ............. σt total flowing effective [hydraulic] fracture length . ............ TFFL vericalBiot’sporoelasticconstant . ........... αv vertical Biot’s poroelastic constant power factor . .......... V BPower vertical Biot’s poroelastic constant scaling factor . ............ V BScale volume of fluid injected (in)/produced (out), total . ............Vin/out in/out volume of fluid injected into (in)/produced from (out) intervali ...........Vi in volume of fluid injected per stage into interval i . ........... Vi Young’smodulus(MMpsi). .... E Young’smodulusforBRFcalculation. .... YMBRIT Young’smodulus,staticcomposite . ..... YMSC x LIST OF ABBREVIATIONS barrelofoilequivalent .. .... BOE barrelofoilperday .................................. BOPD brittlenessfactor .................................. BRF chemicalfractracer(water-based).
Details
-
File Typepdf
-
Upload Time-
-
Content LanguagesEnglish
-
Upload UserAnonymous/Not logged-in
-
File Pages82 Page
-
File Size-