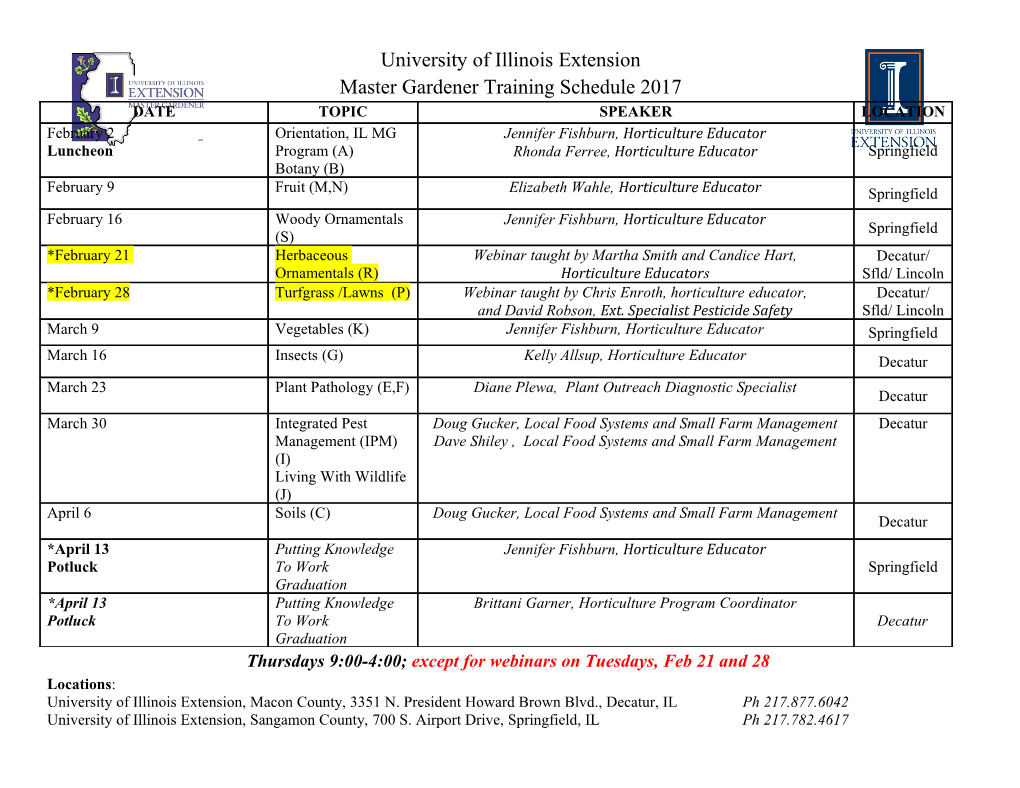
Brain diversity evolves via differences in patterning Jonathan B. Sylvestera, Constance A. Richa, Yong-Hwee E. Loha, Moira J. van Staadenb, Gareth J. Fraserc, and J. Todd Streelmana,1 aParker H. Petit Institute for Bioengineering and Biosciences and School of Biology, Georgia Institute of Technology, Atlanta, GA 30332; bP. Scott Center for Neuroscience, Mind and Behavior, Department of Biological Sciences, Bowling Green State University, Bowling Green, OH 43403; and cDepartment of Animal and Plant Sciences, University of Sheffield, Sheffield S10 2TN, United Kingdom Edited by Gene E. Robinson, University of Illinois, Urbana, IL, and approved April 11, 2010 (received for review January 11, 2010) Differences in brain region size among species are thought to arise within each compartment undergo neurogenesis, maturing and late in development via adaptive control over neurogenesis, as differentiating into functional neurons. Because brain patterning cells of previously patterned compartments proliferate, die, and/or demarcates one region from another, specific compartments may differentiate into neurons. Here we investigate comparative brain initiate, prolong, and/or terminate neurogenesis independently development in ecologically distinct cichlid fishes from Lake Malawi (1, 5, 6). Given the continuum of patterning and neurogenesis in and demonstrate that brains vary among recently evolved lineages brain development, vertebrate lineages might evolve brain di- because of early patterning. Divergence among rock-dwellers and versity by (i) varying the strength or timing of signals from the sand-dwellers in the relative size of the telencephalon versus the ANR and/or MHB; (ii) shifting the position of early patterning thalamus is correlated with gene expression variation in a regulatory boundaries; (iii) altering the timing, rate, or extent of neuro- six3 fezf2 shh irx1b wnt1 circuit (composed of , , , ,and )knownfrom genesis; or (iv) some combination thereof. Expectations from the model organisms to specify anterior-posterior (AP) brain polarity fi shh eld of evolutionary developmental biology suggest a focus on and position the -positive signaling boundary zona limitans intra- early patterning events, because such differences prefigure the thalamica (ZLI) in the forebrain. To confirm that changes in this co- diversity of animal body plans (7), jaws (8, 9), and dentitions (10). expression network are sufficient to produce the differences we In contrast, our understanding of the brain departs from this no- observe, we manipulated WNT signaling in vivo by treating rock- tion, as an extensive literature highlights the role of neurogenesis dwelling cichlid embryos with temporally precise doses of LiCl. Chem- fi “ ” ically treated rock-dwellers develop gene expression patterns, ZLIs, in brain diversi cation. Most prominently, the late equals large and forebrains distinct from controls and untreated conspecifics, model explains how the neocortex (i.e., telencephalon) has but strongly resembling those of sand-dwellers. Notably, endemic evolved to dominate the mammalian brain, and how individual Malawi rock- and sand-dwelling lineages are alternately fixed for lineages (e.g., primates) have further elaborated this region by an SNP in irx1b, a mediator of WNT signaling required for proper tipping the balance among neural cell proliferation, differentia- thalamus and ZLI. Together, these natural experiments in neuroanat- tion, and apoptosis (5, 6, 11, 12). Addressing the genetic and de- omy, development, and genomics suggest that evolutionary changes velopmental mechanisms of brain diversification in nature has in AP patterning establish ecologically relevant differences in the been difficult, however, because few systems offer the necessary elaboration of cichlid forebrain compartments. In general, variation combination of a wide range of brain phenotypes and tractable in developmental patterning might lay the foundations on which experimentation with embryos, against a background of genomic neurogenesis erects diverse brain architectures. and developmental similarity. cichlid | development | evolution | forebrain | WNT signaling Results and Discussion We used cichlid fishes from Lake Malawi to ask when and how rguably the most-studied vertebrate organ, the brain has brains develop diversity in recently evolved lineages. Cichlid adult Aplayed an important role in the evolution of our own species. brain variation is appreciable (Fig. 1A) and is correlated with Modifications of brain structure are responsible for novel behav- ecology and behavior (13–15). For example, algal scrapers exhibit iors that galvanized evolutionary radiation of the major vertebrate small optic lobes and large telencephala (and olfactory bulbs; Fig. groups (1). Following decades of research in model organisms, 1B), whereas planktivores have enlarged optic lobes (Fig. 1D). we now know a great deal about how the process of develop- “Sonar” hunters—species that feed by sensing vibrations—have ment makes a brain (2). We know much less about evolutionary large telencephala and cerebella. This diversity, similar to that mechanisms of brain diversification. observed across seven orders of mammals, including primates, The brain develops under the iterative influence of antagonistic insectivores, marsupials, cetaceans, and bats (16), has evolved anterior and posterior signaling molecules, inductive and repressive rapidly. Hundreds of Lake Malawi cichlid species have diverged transcription factors that receive those signals, and lineage re- from a common ancestor in the last 500,000 years; their genomes striction boundaries that define compartments (2, 3). Just after are highly similar and retain ancestral polymorphism (17). Malawi gastrulation, the initial anterior-posterior (AP) polarity of the brain cichlid brains are thus as different as those of long-diverged is established by a tug-of-war between posteriorizing signals (e.g., mammals [∼150 million years (18)], but their genomes are com- – wnt1) secreted from the midbrain hindbrain boundary (MHB) and parable to those of any two humans. WNT antagonists (e.g., six3, tlc) expressed from the anterior neural ridge (ANR). The MHB develops to demarcate the hindbrain from the fore- plus midbrain (Fig. S1). With the subsequent formation of Author contributions: J.B.S. and J.T.S. designed research; J.B.S. and C.A.R. performed re- the diencephalon–midbrain boundary and the zona limitans intra- search; J.B.S., Y.-H.E.L., M.J.v.S., and G.J.F. contributed new reagents/analytic tools; J.B.S., thalamica (ZLI), the forebrain and midbrain begin to follow sep- C.A.R., and Y.-H.E.L. analyzed data; and J.B.S. and J.T.S. wrote the paper. arate paths of development. The authors declare no conflict of interest. These initial boundaries and signaling centers (i.e., ANR, MHB, This article is a PNAS Direct Submission. ZLI) continue to direct additional patterning and morphogenesis Freely available online through the PNAS open access option. within the three major brain regions. For example, the forebrain 1To whom correspondence should be addressed. E-mail: todd.streelman@biology. differentiates into rostral (e.g., telencephalon, hypothalamus) gatech.edu. and caudal (e.g., thalamus) domains, mediated in part by the ZLI This article contains supporting information online at www.pnas.org/lookup/suppl/doi:10. (4). As brain compartments are patterned, proliferating cells 1073/pnas.1000395107/-/DCSupplemental. 9718–9723 | PNAS | May 25, 2010 | vol. 107 | no. 21 www.pnas.org/cgi/doi/10.1073/pnas.1000395107 Downloaded by guest on September 24, 2021 Fig. 1. Cichlid brains are diverse. (A) Cerebrotype box plots of mammals (blue), Lake Malawi cichlids (green), and Lake Tanganyika cichlids (tan), grouped by brain proportions of the telencephalon (forebrain), mesencephalon (midbrain), and cerebellum (hindbrain). The heavy line in the middle of the box isthe median value, the box itself is the 25–75% interval around the median, the bars are the 10–90% interval, and dots represent data points outside the 95% interval. Mammals have invested heavily in the neocortex (tel); cichlid brains are more proportional in their regional allocations. Despite an orderof magnitude difference in divergence time, the range of variation in brain proportions is comparable from mammals to cichlids. Brains of rock-dwelling (mbuna) cichlids. (B) L. fuelleborni (LF, algivore). (C) M. zebra (MZ, generalist). (D) C. afra (CA, planktivore) in lateral view, anterior is to the left. Black arrowheads, telencephala; gray arrowheads, optic tecta; white arrowheads, cerebella. (Scale bar: 2 mm.) Note that the major midbrain structure of fishes (optic tectum) is of uncertain homology to mesencephalic derivatives in mammals. We focused on species representing the range of ecotypes corresponding to adult structures evolved for contrasting ecol- in Lake Malawi. We studied brain development of three rock- ogical demands. dwelling (mbuna) cichlids—Labeotropheus fuelleborni (LF; obli- gate algal scraper), Maylandia zebra (MZ; generalist), and Cyn- Variation in Forebrain Patterning Prefigures Morphological Differences. otilapia afra (CA; planktivore)—as well as three sand-dwelling We sought to identify the developmental signals that initiate nonmbuna—Copadichromis borleyi (CB; planktivore), Mchenga differences in Malawi cichlid forebrains. Studies in developmental conophorus (MC, generalist), and Aulonocara jacobfreibergi (AJ; models—zebrafish, frog, and mouse—set the context for our ex- sonar hunter). Mbuna and nonmbuna
Details
-
File Typepdf
-
Upload Time-
-
Content LanguagesEnglish
-
Upload UserAnonymous/Not logged-in
-
File Pages6 Page
-
File Size-