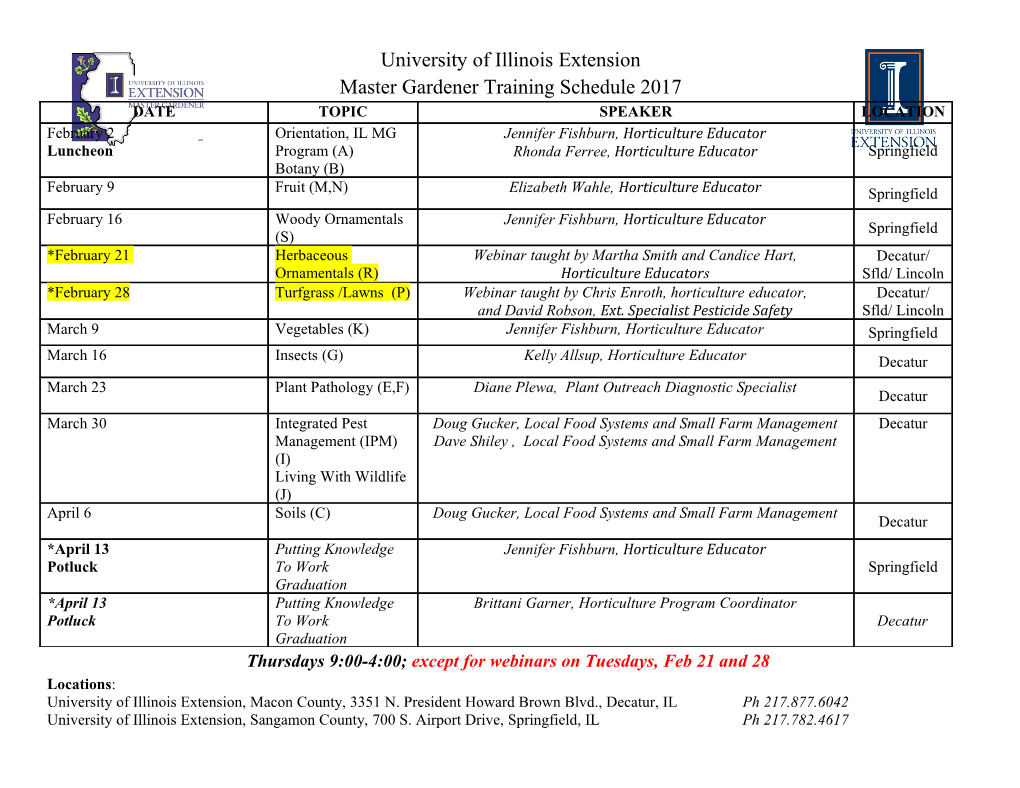
Weightlessness and Rocket Propulsion 1 Risks of Long Term Space Flight and How to Mi?gate Them 2 How do we measure radiaon? In SI units, the amount of radiaon absorbed is measured in Grays (Gy): 1 Gy = 1 joule of energy absorbed per kilogram of material (Another unit you see is “rad”, where 1 rad = 0.01 Gy) For human dosage, we typically talk about mGy. Radiaon deposits energy in uniQue ways into biological systems. milliSieverts (mSv) describes the biological eQuivalent radiaon dosage. (an older unit called the “rem”) This number has the same units as Grays, but it does not measure the same thing. Sieverts take into account the type of radiaon and the biological damage it can cause. 3 How do we measure radiaon? Let’s look at some typical numbers: An average six month stay aboard the ISS: Dose will be higher for astronauts • 80 mSv (solar max) par?cipang in space walks. (Note: • 160 mSv (solar min) NASA avoids spacewalks during • 1 mSv is approximately eQuivalent to 3 high risk periods) chest X-rays (Note: the radiaon is different) • NASA limits exposure to 200 mSv/yr • NCRP limits flight crews to 500 mSv/yr Typical radiaon dose on Earth is 2 mSv/yr due to background radiaon. hap://www.epa.gov/rpdweb00/understand/calculate.html This will vary with al?tude, proximity to nuclear power, local geology, and other 4 factors. What is NASA doing to protect astronauts? NASA follows the protec?on prac?ces that are recommended by the US Naonal Academy of Space Science Board and the US Council on Radiaon Protec?on and Measurement. Astronauts wear dosimeters: • Keeps track of radiaon dosage • Understanding where the space staon has beaer shielding • Serves as a warning device for increased solar radiaon Healthy diet and exercise including an?oxidants. Researching amount of radiaon and biological effects to help protect future astronauts. 5 Outside the Earth’s Magnetosphere The ISS is s?ll protected by the Earth’s magnetosphere. The Mars Radiaon Environment Experiment (MARIE) was placed on board Mars Odyssey to study the amount of radiaon on the journey from Earth to Mars. Mars has no large scale intrinsic magne?c field to protect astronauts on the surface or in orbit. Mars also has a thinner atmosphere, which stops a smaller amount of the Sun’s electromagne?c radiaon and high energy par?cles. MARIE con?nues to study the amount of radiaon in while orbi?ng Mars. 6 Shielding: Materials Aluminum is a poor material for radiaon shield, but we build spacecra out of it. Lead produces many secondaries. Hydrogen is a possibility, but leaking and cryogenic tanking are major difficul?es. On Lunar and Mar?an surfaces we can burrow beneath the surface. Polyethylene (C2H4)n is much, much beer. • Light elements have fewer neutrons. • C decays to helium nucleus instead of neutrons. • NASA scien?sts have developed a s?ff version (RXF1) 7 Shielding: Electrostac Uses large electric fields to repel posi?vely charged par?cles. Larges voltages are reQuired. 1. Poten?al arcing issues. 2. How do we generate these large voltages? 3. How much power is reQuired? Can this system stop X-rays and gamma rays? Currently deemed unfeasible due to arcing issues. 8 Shielding: Magne?c Magne?c shielding could work in a similar way to Earth’s magne?c field deflects the solar wind. The problem is that amounts of power are reQuired to generate the necessary magne?c fields to divert cosmic rays. The magne?c field also needs to be generated by large coils. Because of these technical challenges it is not being funded. 9 Shielding: Plasmas Magnetosphere concepts like M2P2 could be used to protect astronauts from harmful space radiaon. The plasma concepts work to overcome the limits of magne?c systems, which reQuire large power supplies and coils. Currently being inves?gated in the UK 10 Weightlessness We oien say astronauts in space are “weightless.” More accurately, we call this “freefall.” We’re being sloppy. Gravity is s?ll there, but the floor and the astronaut are both falling at the same rate, so the astronaut never hits the floor. As the astronaut falls, he also moves forward; the Earth surface is curving away at the same rate. They are in orbit. Acceleraon due to gravity is about 3% less than at the Earth’s surface. We’ll call LEO a “microgravity environment.” 11 Microgravity Influence: Blood Circulaon Puffy Face Syndrome: • On Earth, the heart must work to get blood to the head, but it does not have to work had to get blood to the legs. • In space, less blood flows to the legs. • Oien accompanied by nasal conges?on, headaches, swollen/ red eyes, dizziness, nausea 12 Microgravity Influence: Blood Circulaon Puffy Face Syndrome: • Heart pumps more blood with each beat. • Kidneys respond by producing more urine. • Pituitary gland produces more an?-diure?c hormones, which makes an astronaut less thirsty. • This combo helps rid the head and chest of excess fluids. • Adap?ng typically takes 2 or 3 days. • On the ground, this return to normal Quickly. 13 Microgravity Influence: Blood Circulaon Kidneys also decrease produc?on of erythropoie?n, which is a hormone that s?mulates red blood cell produc?ons in the bones. Since the volume of blood fluid has decreased, the number of red blood cells also decreases to keep the rao the same as it was on Earth. Upon return to Earth, the red blood cell count returns to normal. 14 Microgravity Influence: Muscle Structure • Muscle Atrophy: con?nuously loose muscle mass and tone due to less use (especially in the leg). • Body adopts a more fetal posi?on. • Slow-twitch endurance muscle fibers decrease, while fast-twitch muscle fibers increase. • Astronauts try to mi?gate the effects by taking medicaon and exercising freQuently. • This could pose issues for long duraon Muscle cells before (A) and aer (B) a 17 day period space flights. in space: slowed protein produc?on, irregulari?es, increased fragility, and increased fat stores (L). 15 Microgravity Influence: Bone Structure • Osteoblasts are bone cells that lay down new minerals. In space they slow down. (No one knows why.) • Osteoclasts are bone cells that chew up the mineral layers. They don’t slow down in space. • This imbalance increases calcium in the blood, which can lead to kidney stones. • Bone degradaon: bones can loose up to 10% of mass and become weaker at a rate of 1 % per month due to lack of minerals. • Aier a 2-3 month mission, astronauts will regain normal bone density within 2-3 years. • Drugs are being studied to mi?gate the problem. 16 Microgravity Influence: Bones and Muscle Structure Astronauts oien experience back pain while in microgravity. Pain might be associated with relaxaon of muscles and ligaments in the spine. 17 Microgravity Influence: Balance and Orientaon The brain ordinarily receives a variety informaon on its environment: 1. Eyes (visual) 2. Muscles and tendons (propriocepve apparatus) 3. Sensors which detect fluid movement in the inner ear (ves?bular apparatus) The brain is used to terrestrial informaon. It has trouble processing the informaon from a microgravity environment. Crew of STS-90 18 Spacewalk Issues While in their spacesuits, astronauts breathe low pressure, pure oxygen. Prior to using the suit, astronauts must purge their body of nitrogen by slowly transi?oning between the air in the shuale/ISS and the pure oxygen of the spacesuit. Aier a spacewalk, pressure can be restored Quickly. If the nitrogen purge is not done, astronauts are subject to decompression sickness. Decompression sickness is damage to ?ssues caused by nitrogen bubbles in the cells and blood stream. 19 Some Soluons NASA Uses We already men?oned that NASA astronauts exercise (up to 2 hours/day) and take medicaon to help with muscle atrophy. To help with the loss of fluids, astronauts use a device called lower body negave pressure. This device uses vacuum cleaner like suc?on to help bring fluid to your legs. Astronauts might spend 30 minutes/day in this device. Much of the research on the ISS is devoted to understanding how long term space flight effects the human body, and what can be done to mi?gate these effects. 20 Propulsion Methods 21 Conserva)on of Momentum Our rocket has three parts 1. Payload (science, human, life support, communicaons…) 2. Structure (rocket, pumps, tanks, structural support…) 3. Fuel (expelled for propulsion) We want a way to calculate the change in speed of the spacecra due to firing our rocket. Momentum: Mass ?mes velocity (p = mv) Momentum is conserved: ini?al momentum eQuals final momentum 22 Conserva)on of Momentum ini?al momentum = final momentum (rocket is at rest) 0 = mcraftvcraft + m fuel (− v fuel ) So this shows the basic tradeoff mcraftvcraft = m fuelv fuel for rockets. To go faster you must: m fuelv fuel 1. Maximize fuel speed vcraft = 2. Maximize fuel mass 3. Minimize craft mass mcraft 23 Conservation of Momentum (Rocket Style): Actually it’s worse than it appears. m fuelv fuel vcraft = mcraft Rockets don’t work by throwing all of their fuel off at once. To get into orbit would kill an astronaut! So, each part of the fuel ejected is pushing against the payload and the remaining fuel!!!! 24 An Example of a High G launcher is a Railgun • A railgun uses electromagne?c forces to accelerate a payload • You have already seen an example of one – ring launcher • One example: 1.6 km barrel launches projec?les are 7.5 km/s (that’s almost 2000 g’s).
Details
-
File Typepdf
-
Upload Time-
-
Content LanguagesEnglish
-
Upload UserAnonymous/Not logged-in
-
File Pages33 Page
-
File Size-