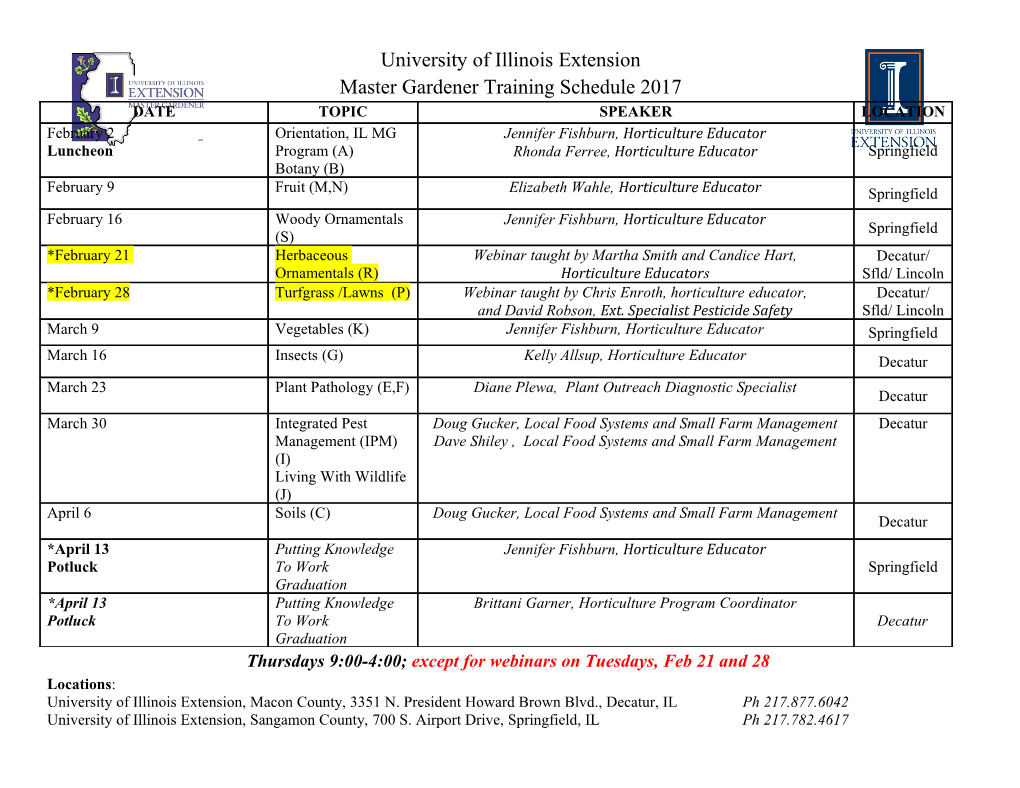
MATH5725 Lecture Notes∗ Typed By Charles Qin October 2007 1 Genesis Of Galois Theory Definition 1.1 (Radical Extension). A field extension K/F is radical if there is a tower of ri field extensions F = F0 ⊆ F1 ⊆ F2 ⊆ ... ⊆ Fn = K where Fi+1 = Fi(αi), αi ∈ Fi for some + ri ∈ Z . 2 Splitting Fields Proposition-Definition 2.1 (Field Homomorphism). A map of fields σ : F −→ F 0 is a field homomorphism if it is a ring homomorphism. We also have: (i) σ is injective (ii) σ[x]: F [x] −→ F 0[x] is a ring homomorphism where n n X i X i σ[x]( fix ) = σ(fi)x i=1 i=1 Proposition 2.1. K = F [x]/hp(x)i is a field extension of F via composite ring homomor- phism F,−→ F [x] −→ F [x]/hp(x)i. Also K = F (α) where α = x + hp(x)i is a root of p(x). Proposition 2.2. Let σ : F −→ F 0 be a field isomorphism (a bijective field homomorphism). Let p(x) ∈ F [x] be irreducible. Let α and α0 be roots of p(x) and (σp)(x) respectively (in appropriate field extensions). Then there is a field extensionσ ˜ : F (α) −→ F 0(α0) such that: (i)σ ˜ extends σ, i.e.σ ˜|F = σ (ii)σ ˜(α) = α0 Definition 2.1 (Splitting Field). Let F be a field and f(x) ∈ F [x]. A field extension K/F is a splitting field for f(x) over F if: ∗The following notes were based on Dr Daniel Chan’s MATH5725 lectures in semester 2, 2007 1 (i) f(x) factors into linear polynomials over K (ii) K = F (α1, α2, . , αn) where α1, α2, . , αn are the roots of f(x) in K Note. Consider tower of field extension F ⊆ K ⊆ L and f(x) ∈ F [x]. If L is a splitting field for f(x) over F , then it is a splitting field for K. If K is generated by roots of f(x) then the converse also holds. Theorem 2.1. Let F be a field and f(x) ∈ F [x]. Then there is a splitting field K of f(x) over F . Theorem 2.2. Let σ : F −→ F 0 be a field isomorphism and f(x) ∈ F [x]. Suppose K, K0 are splitting fields for f(x) and (σf)(x) over F and F 0 respectively. Then there is an isomorphism of fieldsσ ˜ : K −→ K0 which extends σ. This is referred to as the uniqueness of splitting fields. 3 Algebraic Closure Definition 3.1 (Algebraic Extension & Algebraic Closure). Some definitions. (i) A field extension K/F is algebraic over F if every α ∈ K is algebraic over F (ii) A field K is algebraically closed if the only algebraic extension of K is K itself (iii) K is an algebraic closure of F if it is an algebraic field extension of F such that K is algebraically closed Proposition 3.1. Let F ⊆ K ⊆ L be a tower field extensions then: (i) [L : F ] = [L : K][K : F ] (ii) L/F is algebraic if and only if both L/K and K/F are algebraic (iii) Finite field extensions are algebraic (iv) If K = F (α1, α2, . , αn) where α1, α2, . , αn are algebraic over F , then K is finite over F Note. If α is transcendental, then [F (α): F ] = ∞ as irreducible polynomial with root α does not exist. Lemma 3.1. Let F be a field. There exists a set S such that for any algebraic field extension K/F , |K| < |S|. Remark. Either K is countable or |K| = |F |. 2 Definition 3.2 (Splitting Field). A field extension K/F is a splitting field for {fi(x)}i∈I over F if: (i) every fi(x) factorises into linear factors over K (ii) K is generated is the field generated by F and all the roots of all the fi(x)’s Q Note. If I is finite, K is just the splitting field for i∈I fi(x). Proposition 3.2. In field extension K/F , let {αj}j∈J ⊆ K. The subfield of K generated by F and the αj’s is: (i) intersection of all the subfields of K containing F and all the αi’s (ii) union of all F (αj1 , αj2 , . , αjn ) where {αj1 , αj2 , . , αjn } ⊆ J Theorem 3.1. Let F be a field and {fi(x)}i∈I ⊆ F [x] − 0. (i) There exists a splitting field K for {fi(x)}i∈I over F (ii) Suppose there is a field isomorphism σ : F −→ F 0 and K0 is a splitting field for 0 0 {(σfi)(x)}i∈I over F . There is a field isomorphismσ ˜ : K −→ K which extends σ. 4 Field Automorphisms Proposition-Definition 4.1 (Field Homomorphism Over F ). Let K, K0 be field extensions of field F . We say a homomorphism σ : K −→ K0 fixes F or σ is a field homomorphism over F if σ(α) = α for any α ∈ F . Such homomorphisms are linear over F . If furthermore K = K0 and σ is an automorphism then we say σ is a field automorphism over F . Proposition-Definition 4.2 (Galois Group). Let K/F be a field extension of F . Let G be the set of field automorphisms of K over F . Then G is a group when endowed with composition as group multiplication. It is called the Galois group of K/F and is denoted by Gal(K/F ). Lemma 4.1. Let f(x) ∈ F [x]. K/F is a field extension and α ∈ K is a root of f(x). Let σ : K −→ K0 be a field homomorphism over F . Then σ(α) is also a root of f(x). Remark. Any field homomorphism σ : F (α1, α2, . , αn) −→ K over F is determined by values σ(α1), σ(α2), . , σ(αn) since σ fixes F . Corollary 4.1. Let K be a splitting field for f(x) ∈ F [x] over field F . Let α1, α2, . , αn be roots of f(x), so that K = F (α1, α2, . , αn). Then any σ ∈ G = Gal(K/F ) per- mutes the roots of α1, α2, . , αn and so gives an injective group homomorphism G,−→ ∼ Perm({α1, α2, . , αn}) = Sn. Lemma 4.2. Let F be a field and f(x) ∈ F [x]. Let K the splitting field for f(x) over F . 0 Suppose α and α are roots of an irreducible factor f0(x) of f(x) over F . Then there is a 0 σ ∈ G = Gal(K/F ) such that σ(α) = α . In particular, G acts transitively on roots of f0(x). 3 5 Fixed Fields Proposition-Definition 5.1 (Fixed Field). The fixed field of G in K is KG = {α ∈ K : σ(α) = α, for any σ ∈ G} and it is a subfield of K. Definition 5.1 (Prime Maps). Let K/F be a field extension. G = K/F. We define two maps, both called prime. {subgroups of G} ←→ {intermediate fields of K/F }; H 7−→ H0 = KH ; 0 K0 7−→ K0 = Gal(K/K0). Lemma 5.1. Let H1,H2,... denote subgroups of G and K1,K2,... the intermediate fields of K/F . Priming reverses inclusions: 0 0 (i) H1 ⊆ H2 =⇒ H1 ⊇ H2 0 0 (ii) K1 ⊆ K2 =⇒ K1 ⊇ K2 00 00 Lemma 5.2. H1 ⊆ H1 and K1 ⊆ K1 . 00 Definition 5.2 (Closed Subsets). We H1 is a closed subgroup of G if H1 = H1 and K1 is 00 00 00 a closed intermediate field of K/F of K1 = K1 . The closure of H1 and K1 are H1 and K1 respectively. 0 000 0 000 Lemma 5.3. H1 = H1 and K1 = K1 . Theorem 5.1. Priming induces a well defined bijection {closed subgroups of G} ←→ {closed 0 H 0 intermediate fields of K/F }; H 7−→ H = K ; K0 7−→ K0 = Gal(K/K0). 6 Two Technical Results 0 0 Theorem 6.1. Let K1 ≤ K2 be intermediate fields. Then [K1 : K2] ≤ [K2 : K1] = n if n < ∞. 0 0 0 0 Lemma 6.1. Let σ, τ ∈ K1 then σK2 6= τK2 (distinct cosets) =⇒ σ(α) 6= τ(α), i.e. [K1 : 0 K2] ≤ number of distinct σ’s ≤ n. 0 Lemma 6.2. Let σ, τ ∈ G be such that σH1 = τH1. Then for any α ∈ H1, we have σ(α) = τ(α). 0 Remark. As a result, given any left coset C ⊆ H1 ⊆ G and α ∈ H1, we can unambiguously define C(α) = σ(α) where σ is any element of C. 0 0 Theorem 6.2. Let H1 ≤ H2 ≤ G. Suppose n = [H2 : H1] < ∞. Then [H1 : H2] ≤ [H2 : H1]. 4 7 Galois Correspondence Corollary 7.1. Two corresponding results. (i) Let H1 ≤ H2 ≤ G. If H1 is closed and [H2 : H1] < ∞ then H2 is closed and [H2 : H1] = 0 0 [H1 : H2] (ii) Given intermediate fields K1 ≤ K2 ≤ K. Supposed K1 is closed and [K2 : K1] < ∞ 0 0 then K2 is closed and [K2 : K1] = [K1 : K2] Definition 7.1 (Galois Extension). An algebraic field extension K/F is Galois if F is closed, i.e. F = F 00 = KGal(K/F ). Proposition 7.1. Let K/F be a finite field extension. (i) G = Gal(K/F ) is finite (ii) K/F is Galois =⇒ [K : F ] = |G| (iii) If |G| ≥ [K : F ] then K/F is Galois Theorem 7.1 (Fundamental Theorem Of Galois Theory). Let K/F be a finite Galois exten- sion and G = Gal(K/F ).
Details
-
File Typepdf
-
Upload Time-
-
Content LanguagesEnglish
-
Upload UserAnonymous/Not logged-in
-
File Pages18 Page
-
File Size-