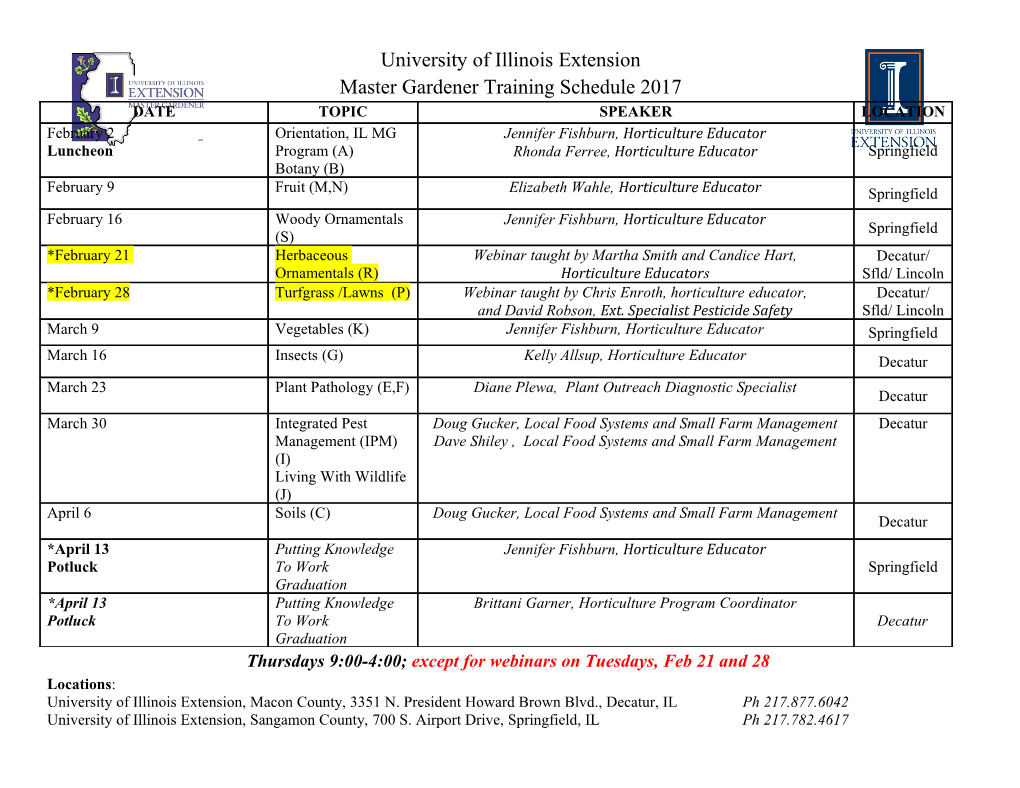
TIME-RESOLVED TERAHERTZ SPECTROSCOPY OF SEMICONDUCTOR QUANTUM DOTS by GEORGI DAKOVSKI Submitted in partial fulfillment of the requirements For the degree of Doctor of Philosophy Thesis advisor: Dr. Jie Shan Department of Physics Case Western Reserve University January, 2008 CASE WESTERN RESERVE UNIVERSITY SCHOOL OF GRADUATE STUDIES We hereby approve the dissertation of ______________________________________________________ candidate for the Ph.D. degree *. (signed)_______________________________________________ (chair of the committee) ________________________________________________ ________________________________________________ ________________________________________________ ________________________________________________ ________________________________________________ (date) _______________________ *We also certify that written approval has been obtained for any proprietary material contained therein. Table of Contents List of figures……………………………………………………………………5 Abstract………………………………………………………………………..10 1. Introduction to optical pump/terahertz probe spectroscopy………………12 1.1 Generation and detection of THz radiation……………………….13 1.2 Applications of THz-TDS…………………………………………...19 1.3 Outline of the thesis………………………………………………...20 2. Localized THz generation via optical rectification in ZnTe………………..22 2.1 Difference-frequency generation…………………………………..24 2.2 Experimental setup………………………………………………….26 2.3 Results………………………………………………………………..27 2.4 Numerical simulation and discussion……………………………..30 2.5 Conclusions………………………………………………………….39 1 3. Finite beam-size effects in optical pump/THz probe spectroscopy………………………………………………………………….41 3.1 Theoretical model…………………………………………………...42 3.2 Experimental setup………………………………………………….45 3.3 Results and discussion……………………………………………..46 3.4 Conclusions………………………………………………………….49 4. Introduction to semiconductor quantum dots……………………………...51 4.1 Fabrication and Characterization………………………………….53 4.2 Applications………………………………………………………….57 4.3 Electronic structure of semiconductor quantum dots…………....59 4.3.1 Parabolic band model……………………………………..61 4.3.2 Multiband model for CdSe QDs…………………………..66 4.3.3 Multiband model for PbSe QDs…………………………..71 4.4 Limitations on the use of the kp⋅ method…………………..…..76 5. Terahertz electric polarizability of excitons in CdSe and PbSe quantum dots………………………………………………………………….78 5.1 Response of a single exciton to THz radiation…………………...80 5.2 Experimental setup………………………………………………….81 5.3 Results and discussion……………………………………………..82 2 5.4.1 Calculations based on the parabolic band model……....88 5.4.2 Calculations based on the multiband models.……….....90 5.5 Conclusions………………………………………………………….92 6. Multiexciton Auger recombination in semiconductor quantum dots studied with THz-TDS……………………………………….94 6.1 Experimental setup………………………………………………….96 6.2 Results…………………..……………………………………………96 6.3 Discussion……………………………………………………….....100 6.4 Conclusions………………………………………………………...103 7. Response of multiple excitons in CdSe nanoparticles studied with terahertz time-domain spectroscopy……………………….104 7.1 Experimental setup………………………………………………..105 7.2 Results………………………………………………………………105 7.3 Data analysis……………………………………………………….108 7.4 Inclusion of the Coulomb interaction and discussion…………..109 7.5 Conclusions………………………………………………………...113 3 8. Size-dependence of degenerate two-photon absorption in semiconductor QDs…………………………………………………………114 8.1 TPA in bulk materials………………………………………………116 8.2 Optical pump/white-light probe technique……………………….117 8.3 Experimental setup and details…………………………………..118 8.4 Results………………………………………………………….......119 8.5 Model for TPA in QDs……………………………………………...125 8.6 Conclusions………………………………………………………...129 9. Summary……………………………………………………………………..130 References…………………………………………………………………………....133 4 List of Figures 1. Fig. 1.1 Standard THz-TDS spectrometer, based on an amplified laser system. THz radiation is generated through optical rectification and detected through electrooptic sampling in ZnTe. 2. Fig. 2.2.1 Sketch of the THz emitter employed in the measurement (not to scale): optical pump beam (800 nm) is focused onto a 20 μm ZnTe emitter ( (110) orientation) on a 500 μm ZnTe substrate ( (100) orientation). Translation of the lens allows variation of the effective size of the emitter. 3. Fig. 2.3.1 Normalized spectral density E( ω ) 2 versus the FWHM of the optical excitation a . Three representative frequencies are shown in symbols. Solid line is a fit to Eq. (2.4.16). Inset: size dependence of the total THz power (normalized) in the regime of two-photon absorption using 100% (filled) and 50% (open) of the available excitation power. 4. Fig. 2.3.2 Characteristic size of the emitter ac as defined in the text for the transition of spectral density behavior (from a0 to a−2 dependence) versus the THz wavelength λTHz . Symbols: experiment; line: simulation. 5. Fig. 2.4.1 Typical layered geometry utilized in our experiment. 6. Fig. 2.4.2 (a): Simulated spectral density E( ω ) 2 at 0.9, 1.4, and 1.9 THz (solid lines) versus the FWHM of the optical excitation a for a 30o collection 5 angle. (b): simulated spectral density E( ω ) 2 at 1.4 THz for collection angles of 30°, 15°, and 7.5°. Dashed lines illustrate a−2 dependence. 7. Fig. 3.1.1 Simulation of the radiation power at 0.45 (dashed line) and 1.15 THz (solid line) emitted from a source of a fixed peak polarization but varying sizes. The radiation is collected by off-axis parabolic mirrors of 64 mm in diameter and 120 mm in focal length as used in the experiment. Critical 4 2 sizes ac for the transition from the a to the a dependence are indicated for both frequencies. 8. Fig. 3.3.1 (a) Complex conductivity of photo-induced carriers in CdTe at room temperature as a function of frequency for pump sizes of 3.6mm FWHM (solid lines) and 0.7mm (dashed lines). Fit to the Drude model is shown for 3.6mm pump (grey lines). (b) Pump size dependence of σ( ω ) 2 at 0.45 (empty circles) and 1.15 THz (filled circle). Lines: fits to Eq. (3.1.1). 9. Fig. 3.3.2 Concentration (empty circles) (a) and mobility (empty squares) (b) of photo-induced carriers in CdTe inferred from the fit of the measurement to the Drude model over a range of pump beam sizes. Data that cannot be described by the Drude model are indicated by a ‘x’; line (a): fit of the extracted density to the model as described in the text; (filled circles) (a): peak carrier density inferred from our model. 10. Fig. 4.1 Linear absorption of colloidal CdSe/ZnS nanoparticles of varying sizes dissolved in hexane. 6 11. Fig. 4.1.1 a) High resolution TEM image of PbSe QDs, revealing the lattice structure; b) Low resolution image, showing an ensemble of QDs [88]. 12. Fig. 5.3.1 THz electric-field waveform transmitted through an unexcited suspension of 3.1-nm-radius PbSe QDs (black solid line) and the photoinduced change in this waveform (grey solid line). Inset: dependence of the pump-induced response on the pump-probe delay time in the first 10 ps. (b) Spectral dependence of the photoinduced complex dielectric response of the QD suspension (solid grey lines). Dashed lines in (b) represent a model with Δε'const= and Δε'' = 0 and the dashed line in (a) is the change in the THz electric-field waveform predicted by the model. 13. Fig. 5.3.2 Schematics of the electric polarizability of an exciton strongly quantum confined in a QD of radius R to an externally applied electric field F . Within the parabolic-band effective mass model as described in the text, in the absence of the field (left), the ground-state exciton possesses a spherically-symmetric electron and hole distribution centered at the center of the dot (only the electron distribution shown) which result in a zero net dipole moment. The externally applied electric field perturbs the exciton wave function and the charge spatial distribution (right), which leads to a net dipole moment P in the QD. 14. Fig. 5.3.3 Polarizability of photoinduced excitons strongly quantum-confined in CdSe and PbSe QDs as a function of the QD radius. Details about the 7 experimental results (symbols) and the theoretical results based on a parabolic-band effective mass model (dotted lines) and multiband effective mass models (solid lines) are described in the text. 15. Fig. 6.2.1 THz electric-field waveform transmitted through an unexcited suspension and the photoinduced change in the waveform (red line) for an average of <>=N.15 excitons per QD. Inset: spectral dependence of the photo-induced complex dielectric response of the QD suspension (solid lines). Dashed lines in the inset represent a model with Δε'const= and Δε'' = 0 ; dashed line in the main panel is the change in the THz electric-field waveform predicted by the model. 16. Fig. 6.2.2 Pump-dependence of different average exciton populations in a 3.3-nm CdSe QD. 17. Fig. 6.2.3 Dependence of the normalized real part of the induced complex dielectric response (by the molar concentration) on the average number of excitons per QD (black dots). Red line: fit to a power law, y = Ax p , p ≈ 05. 18. Fig. 6.3.1 Size-dependence of multiexciton recombination lifetimes obtained via THz-TDS (red symbols). Black symbols: data obtained via transient absorption spectroscopy. Straight lines: fits to a R3 dependence. 19. Fig. 7.2.1 Dependence of the normalized real part of the induced complex dielectric response (by the molar concentration) on the average number of excitons per QD (red dots). Black dots: recalculated exciton population, 8 accounting for the Auger recombination. Solid line: model of non-interacting carriers. 20.
Details
-
File Typepdf
-
Upload Time-
-
Content LanguagesEnglish
-
Upload UserAnonymous/Not logged-in
-
File Pages149 Page
-
File Size-