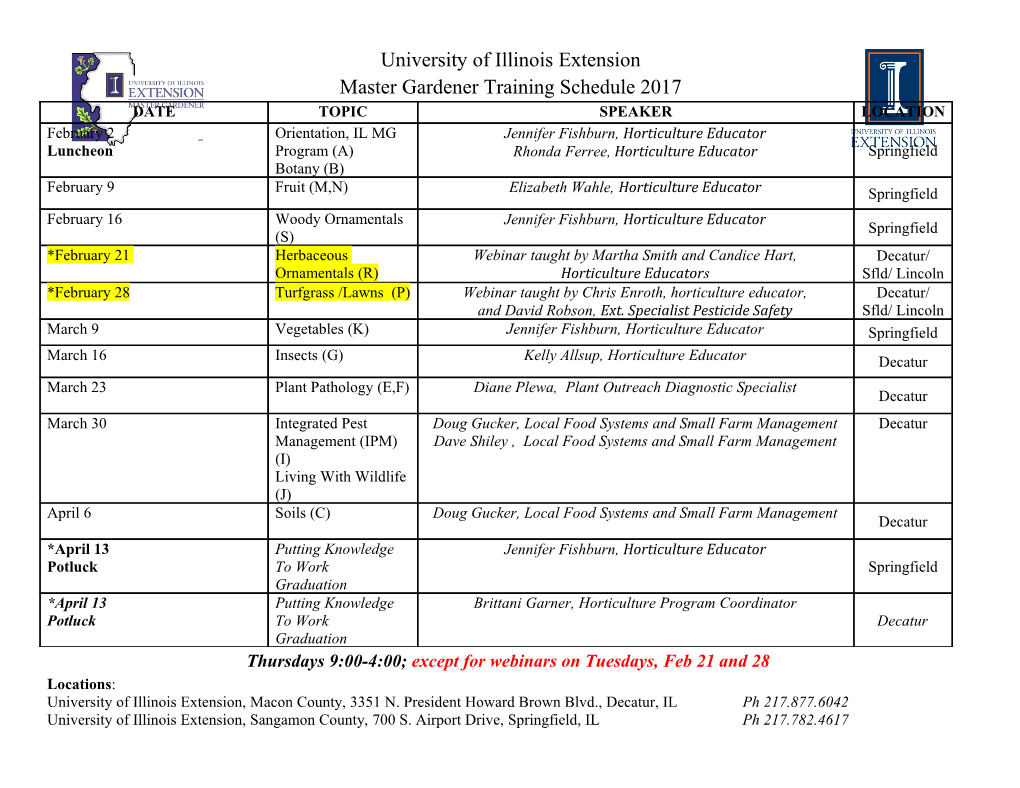
Article One-Pot Multi-Enzymatic Production of Purine Derivatives with Application in Pharmaceutical and Food Industry Javier Acosta 1, Jon del Arco 1, Sara Martinez-Pascual 1, Vicente Javier Clemente-Suárez 1,2 and Jesús Fernández-Lucas 1,2,* 1 Applied Biotechnology Group, European University of Madrid, c/ Tajo s/n, Villaviciosa de Odón, Madrid 28670, Spain; [email protected] (J.A.); [email protected] (J.d.A.); [email protected] (S.M.-P.); [email protected] (V.J.C.-S.) 2 Grupo de Investigación en Desarrollo Agroindustrial Sostenible, Universidad de la Costa, CUC, Calle 58 # 55-66, Barranquilla 080002, Colombia * Correspondence: [email protected]; Tel.: +34-912-115147 Received: 30 November 2017; Accepted: 28 December 2017; Published: 1 January 2018 Abstract: Biocatalysis reproduce nature’s synthetic strategies in order to synthesize different organic compounds. Natural metabolic pathways usually involve complex networks to support cellular growth and survival. In this regard, multi-enzymatic systems are valuable tools for the production of a wide variety of organic compounds. Methods: The production of different purine nucleosides and nucleoside-5′-monophosphates has been performed for first time, catalyzed by the sequential action of 2′-deoxyribosyltransferase from Lactobacillus delbrueckii (LdNDT) and hypoxanthine-guanine-xanthine phosphoribosyltransferase from Thermus themophilus HB8 (TtHGXPRT). Results: The biochemical characterization of LdNDT reveals that the enzyme is active and stable in a broad range of pH, temperature, and ionic strength. Substrate specificity studies showed a high promiscuity in the recognition of purine analogues. Finally, the enzymatic production of different purine derivatives was performed to evaluate the efficiency of multi-enzymatic system LdNDT/TtHGXPRT. Conclusions: The production of different therapeutic purine nucleosides was efficiently catalyzed by LdNDT/TtHGXPRT. In addition, the resulting by-products were converted to IMP and GMP. Taking all of these features, this bioprocess entails an efficient, sustainable, and economical alternative to chemical synthetic methods. Keywords: 2′-deoxyribosyltransferase; phosphoribosyltransferases; cascade reactions; purine nucleoside analogues; dietary nucleotides 1. Introduction Nucleoside analogues, NAs, form a very large family of compounds, with more than 40 presently used in medicine as antiviral and anticancer agents [1,2]. Actually, a variety of therapeutic nucleosides and nucleotides, which are in the WHO “List of essential medicines” are produced chemically, such as Abacavir (HIV therapeutic), Capecitabine (anticancer), Cytarabine (anticancer, antiviral), Vidarabine (antiviral), or Floxuridine (anticancer), among others. Due to the economic and social relevance of these kind of drugs, the availability of a stereo-selective and cheap synthetic method is relevant for the industry and developing countries. Furthermore, nucleoside-5′-monophosphates, NMPs, are often used as food additives or intermediates by the pharmaceutical industry. For example, some dietary nucleotides, such as inosinic acid (inosine-5′-monophosphate, IMP) or guanosinic acid (guanosine-5′-monophosphate, GMP), are common additives used as flavour enhancers in foods, since they induce an umami taste Catalysts 2018, 8, 9; doi:10.3390/catal8010009 www.mdpi.com/journal/catalysts Catalysts 2018, 8, 9 2 of 11 sensation [3]. As a result, current demand for nucleotides in the food additives market is increasing, and the production of nucleotides has been widely studied. NAs and NMPs have been traditionally synthesized by chemical methods through multistep processes requiring protection and de-protection steps for the labile groups, and isolation in almost every step due to the poor regio- or stereoselectivity of the reactions [4–8]. These drawbacks lead to a high price of these valuable compounds, limiting their application. Nowadays, the application of bioprocesses that are catalyzed by whole cells or enzymes in industry is gaining ground against traditional chemical synthetic processes. In this context, the enzymatic synthesis of NAs and NMPs shows many advantages, such as one-pot reactions under mild conditions, high stereo-, and regioselectivity, and an environmentally friendly technology [4–11]. Purine metabolism is a metabolic route of vital importance in all the living organisms, since purines are essential for the synthesis of nucleic acids (DNA and RNA), proteins, and other metabolites. In the de novo pathway cells use simple precursors like glycine, glutamine, or aspartate for the synthesis of the different purine nucleotides. On the contrary, the salvage pathway is composed by a group of reutilization routes by which the cell can satisfy its purine requirements from endogenous and/or exogenous sources of preformed purines. In this regard, numerous enzymes from purine salvage pathway have become valuable catalysts for mono or multi-enzymatic synthesis of nucleosides and nucleotides, such as nucleoside kinases (NKs) [12–15], phosphoribosyltransferases [7,9–11], nucleoside phosphorylases [4,8,16,17], 2′-deoxyribosyltransferases [5,18–20], among others. The use of multi-enzymatic systems in organic synthesis offers several advantages, such as the realization of more complex synthetic schemes, the ability to make reversible processes irreversible, to shift the equilibrium reaction in desired way, and the partial or total elimination of product inhibition problems or the prevention of the shortage of substrates by dilution or degradation in the bulk media [13]. In this regard, the aim of this work is the development of a novel multi-enzymatic system for the industrial production of different NAs and NMPs with application in pharmaceutical and food industry. To achieve this objective, an in vitro multienzymatic system composed by 2′-deoxyribosyltransferase from Lactobacillus delbrueckii (LdNDT) and the hypoxanthine-guanine-xanthine phosphoribosyltransferase from Thermus themophilus HB8 (TtHGXPRT), is developed. As shown in Figure 1, the sequential action of LdNDT and hypoxanthine-guanine-xanthine TtHGXPRT can efficiently catalyze the synthesis of NAs and NMPs in two steps. Figure 1. Multi-enzymatic system LdNDT/TtHGXPRT. B1 and B2: Purine bases; dN: 2′-deoxynucleoside; NMP: nucleoside-5′-monoposphate. Nucleoside 2′-deoxyribosyltransferases (NDTs, E.C. 2.4.2.6) catalyze the transglycosylation reaction between a 2′-deoxynucleoside donor and a nucleobase acceptor. According to their substrate specificity, NDTs can be classified into two classes: type I (PDT), specific for purines (Pur↔Pur), and type II (NDT), which catalyze the transfer between purines and/or pyrimidines (Pur↔Pur, Pur↔Pyr, Pyr↔Pyr) [5,19,20] (Figure 1). Catalysts 2018, 8, 9 3 of 11 Purine and pyrimidine phosphoribosyltransferases (PRTs) catalyze the reversible transfer of the 5-phosphoribosyl group from 5-phospho-α-D-ribosyl-1-pyrophosphate (PRPP) to purine and pyrimidine nucleobases or derivatives in the presence of Mg2+ (Figure 1). The coupled system LdNDT/TtHGXPRT allowed for the enzymatic production of different NAs by transglycosilation reaction using 2′-deoxynosine or 2′-deoxyguanosine as donors, and different modified purine nucleobases as acceptors. Interestingly, TtHGXPRT can slightly shift the equilibrium reaction of the NDT-catalyzed transglycosylation reaction in desired way, and partially eliminate undesired by-products (hypoxanthine or guanine) from bulk-media using PRTs to synthesize highly valuable nucleoside-5′-monophosphates (NMPs), commercially available as food additives (IMP or GMP). 2. Results 2.1. Production, Purification and Substrate Specificity of Recombinant LdNDT The ndt gene, encoding a 2′-deoxyribosyltransferase from Lactobacillus delbrueckii, was cloned and over-expressed in E. coli BL21 (DE3), as described above. The recombinant N-terminal His6-tagged LdNDT was purified using affinity chromatography. After this process, N-terminal His6-tagged was removed from LdNDT using thrombin to avoid precipitation of the protein. To classify our recombinant protein, we carried out the enzymatic synthesis of nucleosides using different ribo- and 2′-deoxyribonucleosides as donors and several purine and pyrimidine bases as acceptors (Table 1). In this regard, uridine (Uri), adenosine (Ado), cytdine (Cyd), 2′-deoxyadenosine (dAdo), 2′-deoxyuridine (dUri), 2′-deoxycytidine (dCyd), thymidine (dThd), 2′-deoxyinosine (dIno) and 2′-deoxyguanosine (dGuo) were tested as donors, and adenine (Ade), uracil (Ura), cytosine (Cyt), thymine (Thy) and hypoxanthine (Hyp) were used as acceptors. LdNDT did not show any activity on Uri, Cyd and Ado (data not shown). LdNDT is type II NDT, active over purine and pyrimidine 2′-deoxynucleosides and bases with a strong preference for dCyd and dUrd as best donors, followed by Thd. In turn, the most and least preferred acceptors are Ade, Cyt and Ura. Table 1. Substrate specificity of LdNDT upon synthesis of natural nucleosides. Acceptor Specific Activity (IU/mg Protein) Donor Ade Ura Cyt Thy Hyp dAdo -- 43 ± 5 83 ± 8 45 ± 2 7.5 ± 1 dUrd 187 ± 2 -- 165 ± 6 135 ± 5 106 ± 7 dCyd 189 ± 7 104 ± 6 -- 48 ± 8 101 ± 8 Thd 101 ± 4 113 ± 3 113 ± 6 -- 88 ± 8 dIno 22 ± 8 40 ± 4 64 ± 7 40 ± 0 -- dGua 116 ± 9 54 ± 4 80 ± 2 22 ± 4 20 ± 3 Reaction conditions: 0.3 μg of enzyme were incubated at 50 °C and and 300 rpm for 5 min with 10 mM substrates in 50 mM MES buffer, pH 6.5 in a final volume of 40 μL. 2.2. Biochemical Characterization
Details
-
File Typepdf
-
Upload Time-
-
Content LanguagesEnglish
-
Upload UserAnonymous/Not logged-in
-
File Pages11 Page
-
File Size-