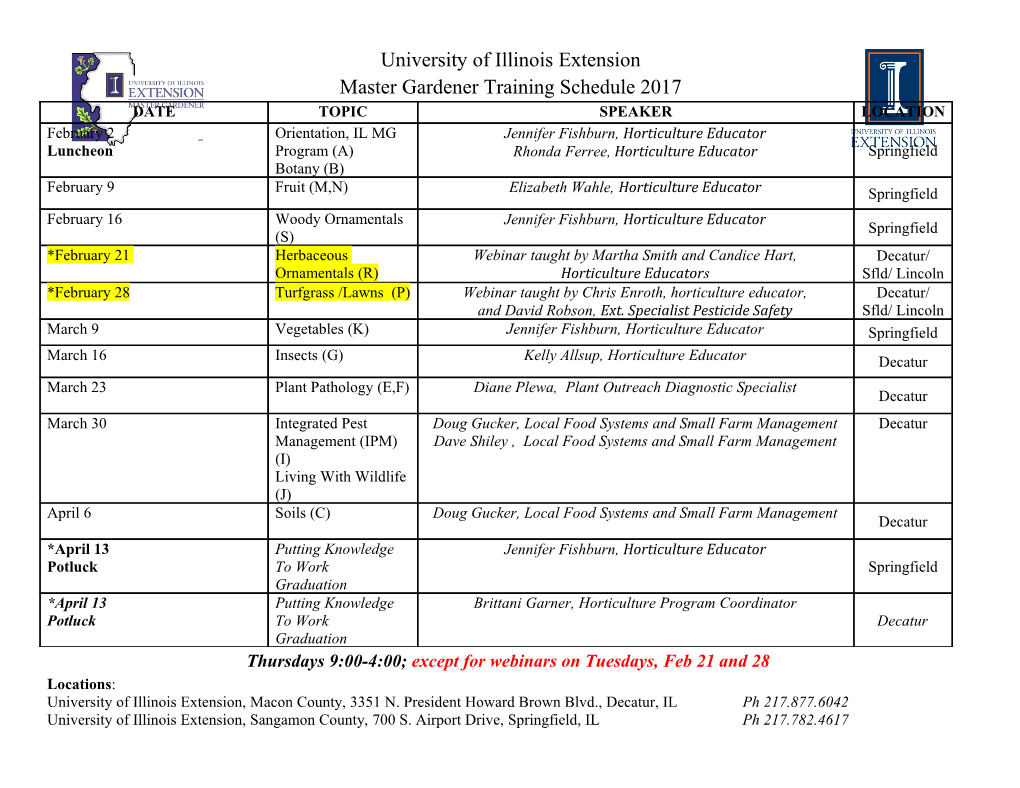
<p> 1</p><p>Appendix A. Fish nutrient excretion was not a confounding factor. </p><p>Under some conditions, nutrient excretion from fish can affect algal communities and therefore the zooplankton [1]. We use multiple lines of evidence to show that such nutrient excretion by fish did not confound our results. </p><p>We estimated nutrients excreted by fish during the experiment based on the minimal diet used as well as the fish’s basal metabolic demands. Our experimental protocol was designed to minimize fish nutrient excretion by starving the fish before placement in the cages. </p><p>Fish were rotated into the experiments weekly, which allowed us to feed the fish to ensure their health while not in the experiment, and to feed them minimally while in the experiment. Bluegill in our experiments were fed 200 Daphnia twice weekly, each Daphnia containing approximately 1 µg of N [2] and 0.1 µg of P [3]. Assuming that bluegill assimilate a minimum of 30% of the N and 37% of the P in their diet [4], we estimate that the three bluegill in each tank excreted 0.09 µg N l-1 d-1 and 0.01 µg P l-1 d-1 as a direct result of their consumption of Daphnia. We estimated nutrients excreted through basal metabolic demands using rates from a previous study of bluegill about the same size as those in our experiment (mean total length of 50.6 mm and weight of 1.96 g wet mass) that were subject to a 36 hour starvation period [5]. In this case, nutrient excretion due to the three fish in each tank was estimated as 1.40 g N l-1 d-1 and 0.15g P l-1 d-1. Summing these two sources, total excretion rates from fish are estimated as 1.49 g N l-1 d-1 and 0.16g P l-1 d-1. </p><p>Similar rates were obtained based on results from another study of bluegill [6] and from studies of other fish species that examined fish of size and diet used our experiment [7-9] supporting the robustness of our estimates. 2</p><p>We next provide several lines of evidence that indicate that fish nutrient excretion was negligible compared to other sources of nutrients in the experiment. First, nutrients added directly to the tank to provide nutrients for phytoplankton growth were added at levels that far exceeded the predicted influence of fish. Nutrient addition rates of 93.5 g N l-1 d-1, and 7.0 g P l-1 d-1 were used before the initiation of predator (fish) treatments, and over time reduced to 46.8 </p><p>g N l-1 d-1, and 3.5 g P l-1 d-1 after fish were added to the tanks (see main text). Even these lower supply rates at the end of the experiment far exceeded those of fish by 31 and 22 fold for N and P, respectively. </p><p>Second, the nutrient supply from the entire plankton community, including zooplankton and bacteria, far exceeded that of fish. Hudson et al. [10] and Nowlin et al. [11] demonstrated that P regeneration by the planktonic community dominates the net supply to plankton, and is strongly correlated in lakes over a nutrient gradient of 4-80 g/l of total phosphorous. Applying the strong relationship between P regeneration and total P found by Hudson et al. [10] to our average total P (40 ug P l-1) value yields a regeneration rate of 5.4 g P l-1 d-1, which far exceed </p><p>(by 33 fold based on [7]) that predicted for fish in the experiments. Using the results of Nowlin et al. [11] yields an even higher estimate. Further, there was additional contribution to the total nutrient regeneration by snails, hydra, and microorganisms on the sides and bottoms of the tanks, further reducing the contribution of fish excretion. A similar analysis yields the same results for </p><p>N excretion. </p><p>Third, a second method of estimating organism nutrient regeneration indicates that it far exceeded that of fish. There has been much research performed on zooplankton nutrient regeneration. Urabe et al. [12] examined nutrient regeneration by a natural assemblage of zooplankton in a natural lake monthly from June to September. In this study two parameters 3 known to affect nutrient excretion rate were similar to that in our tanks; the mean temperature was 23˚C, and total N:P ratio was 17:1. Further, zooplankton were dominated by copepods in some months, and by cladocerans in others, and therefore covered a large range of communities similar to ours. Therefore, this is an ideal study to use to estimate the mass specific nutrient supply by zooplankton, which averaged a nutrient regeneration rate per unit dry weight of zooplankton of 2.9 ug P mg-1 DW d-1. Appling this rate to the mean dry weight density of zooplankton in the fish treatment tanks (0.378 mg/l) leads to an estimate of 1.1 g P l-1d-1. This value far exceeds that of that estimated from fish by 6.9 fold. This value is also a rather conservative estimate based on other studies. For example Carrillo et al. [13] and Attayde & </p><p>Hansson [14] have reviewed the literature on nutrient excretion rates of zooplankton, and the median value (15 ug P mg-1 DW d-1) represents even larger predicted nutrient supply rate from zooplankton. Further, estimated zooplankton excretion using published equations that estimate excretion as function of zooplankton size and temperature [15,16] yielded equivalently high estimates nutrient regeneration by zooplankton (both over 10 g P l-1d-1). Therefore, analysis using published zooplankton excretion rates indicates that the contribution of P excretion from fish was very much lower than that of zooplankton. As in the previous analysis, the fact that zooplankton only represent one organism of many organisms regenerating nutrients (e.g. microplankton, snails) further reduces the predicted negligible contribution from fish. A similar analysis yields the same results for N excretion. </p><p>Fourth, nutrient analyses showed no effect of fish on total phosphorous, total nitrogen, and the TN:TP ratio. Water samples were collected on September 2 and 3 by collecting 50 ml of water, 5 cm below the surface in the middle of each tank of four of the six replicates. September </p><p>2 was chosen because it was a day nutrients were added to tanks. By measuring nutrients before 4 these manipulations (on Sept 2), and the day after (on Sept 3), we hoped to span the largest range of nutrient levels in the tanks. Nutrient concentrations were determined using standard automated colorimetric procedures on a Technicon Auto Analyzer II according to methods detailed in Davis</p><p>& Simmons [17]. Total nitrogen (TN) was determined using the persulfate digestion method </p><p>4500-N (C) [18] followed by analysis for NO3. Total phosphorus (TP) was determined by persulfate digestion after the method of Menzel & Corwin [19], followed by analysis for soluble reactive phosphorus. The effect of fish treatment and day on total phosphorous (TP), total nitrogen (TP), and TN:TP ratio was analyzed using a repeated-measures ANOVA after confirming that the data met assumptions of normality and homogenetity of variances, based on </p><p>Levene’s tests. Fish treatment did not significantly affect TP (F1,6 = 0.06, p = 0.81), TN (F1,6 = </p><p>0.10, p = 0.77), or the TN:TP ratio (F1,6 = 0.11, p = 0.76). The day of sampling was a significant factor for TP (p=0.001) and TN (p=0.004), and a marginally significant factor for TN:TP (p = </p><p>0.054), although these differences were very small. Average values for each response on the two consecutive days were, respectively, 42 and 49 ug l-1 TP, 850 and 925 ug l-1 TN, and 20.5 and </p><p>19.0 TN:TP. </p><p>Fifth, the amount of nutrients in an active form that can be utilized by phytoplankton excreted by fish was estimated to be low compared to the total amount in the tanks. Although we only measured total N and P, in a cattle tank experiment that used a methodology similar to ours </p><p>(containing a natural assemblage of zooplankton and snails), Hall et al. [20] measured soluble reactive phosphorus (SRP). They added N and P in several ratios including 13:1, which was very close to our supply ratio. They used two nutrient supply rates, the higher of which yielded zooplankton density and TP nearly identical to that in the fish treatments in our experiment. The </p><p>SRP level in this treatment was 10 g l-1. This level is 62.5 times the estimated daily 5 phosphorous contribution from fish in the experiment, indicating that any contribution from fish was negligible. An analysis of NH4 yielded similar results for nitrogen. </p><p>In summary, the nutrient release from fish was negligible because we supplied much more nutrients then the fish excreted on a daily basis, and regeneration by other organisms in the tank far exceeded any contribution from the fish.</p><p>REFERENCES</p><p>1 Vanni, M. J. 2002 Nutrient cycling by animals in freshwater ecosystems. Annu. Rev. Ecol. </p><p>Syst. 33, 341-370. </p><p>2 Sterner, R. W., Hagemeier, D. D., Smith, W. L., & Smith, R. F. 1993. Phytoplankton nutrient</p><p> limitation and food quality for Daphnia. Limnol. Oceanogr. 38, 857-871. </p><p>3 Vijverberg, J., & Frank, T. T. 1976. The chemical composition and energy contents of </p><p> copepods and cladocerans in relation to their size. Freshw. Biol. 6, 333-345. </p><p>4 Glaholt, S. P., & Vanni, M. J. 2005. Ecological response to simulated benthic-derived </p><p> nutrient subsidies mediated by omnivorous fish. Freshw. Biol. 50, 1864-1881.</p><p>5 Mather, M. E., Vanni, M. J., Wissing, T. E., Davis, S. A., & Schaus, M. H. 1995 </p><p>Regeneration of nitrogen and phosphorus by bluegill and gizzard shad: Effect of feeding </p><p> history. Can. J. Fish. Aquat. Sci. 52, 2327-2338.</p><p>6 Torres, L. E., & Vanni, M. J. 2007. Stoichiometry of nutrient excretion by fish: </p><p> interspecific variation in a hypereutrophic lake. Oikos 116, 259-270.</p><p>7 Cui, Y., & Wootton, R. J. 1988. Bioenergetics of growth of a cyprinid, Phoxinus phoxinus: </p><p> the effect of ration, temperature and body size on food consumption, faecal production </p><p> and nitrogenous excretion. J.Fish Biol. 33, 431-443. 6</p><p>8 Persson, A. 1997. Phosphorus release by fish in relation to external and internal load in </p><p> a eutrophic lake. Limnol. Oceanogr. 42, 577-583. </p><p>9 Braband, Å., Faafeng, B. A., & Nilssen, J. P. M. 1990. Relative importance of phosphorus </p><p> supply to phytoplankton production: fish excretion versus external loading. Can. J. Fish. </p><p>Aquatic Sci. 47, 364-372. </p><p>10 Hudson, J. J., Taylor, W. D. & Schindler, D. W. 1999 Planktonic nutrient regeneration and </p><p> cycling efficiency in temperate lakes. Nature 400, 659-661. </p><p>11 Nowlin, W. H., Davies, J-M. & Mazumder, A. 2007 Planktonic phosphorus pool sizes and </p><p> cycling efficiency in coastal and interior British Columbia lakes. Freshw. Biol. 52, 860-877.</p><p>12 Urabe, J., Nakanishi, M. & Kawabata, K. 1995. Contribution of metazoan plankton to the </p><p> cycling of nitrogen and phosphorus of Lake Biwa. Limnol. Oceanogr. 40, 232-241.</p><p>13 Carrillo, P., Reche, I. & Cruz-Pizarro, L. 1996 Intraspecific stoichiometric variability and </p><p> the ratio of nitrogen to phosphorus resupplied by zooplankton. Freshw. Biol. 36, 363-374. </p><p>14 Attayde, J. L. & Hansson, L. 1999 Effects of nutrient recycling by zooplankton and fish on </p><p> phytoplankton communities. Oecologia 121, 47-54.</p><p>15 Sereda, J. M., Hudson, J. J. & Mcloughlin, P. M. 2008 General empirical models for </p><p> predicting the release of nutrients by fish, with a comparison between detritivores and non-</p><p> detritivores. Freshw. Biol. 53, 2133-2144.</p><p>16 Ejsmont-Karabin, J. 1984 Phosphorus and Nitrogen Excretion by Lake Zooplankton(Rotifers </p><p> and Crustaceans) in Relationship to Individual Body Weights of the Animals, Ambient </p><p>Temperature and Presence or Absence of Food. Ekologia Polska 32, 3-42. 7</p><p>17 Davis, C.O. & Simmons, M.S. 1979 Water chemistry and phytoplankton field and </p><p> laboratory procedures. Special Report No. 70. Ann Arbor, MI: University of Michigan, </p><p>Great Lakes Research Division. </p><p>18 American Public Health Association (APHA) 1998. Standard methods for the examination of</p><p> water and wastewater. Washington, DC: American Public Health Association.</p><p>19 Menzel, D.W. & Corwin, N. 1965 The measurement of total phosphorus liberated in </p><p> seawater based on the liberation of organically bound fractions by persulfate oxidation. </p><p>Limnol. Oceanogr. 10, 280-281.</p><p>20 Hall, S. R., Leibold, M. A. & Lytle, D. A. 2007 Grazers, producer stoichiometry, and the </p><p> light : nutrient hypothesis revisited. Ecology 88, 1142-1152.</p>
Details
-
File Typepdf
-
Upload Time-
-
Content LanguagesEnglish
-
Upload UserAnonymous/Not logged-in
-
File Pages7 Page
-
File Size-