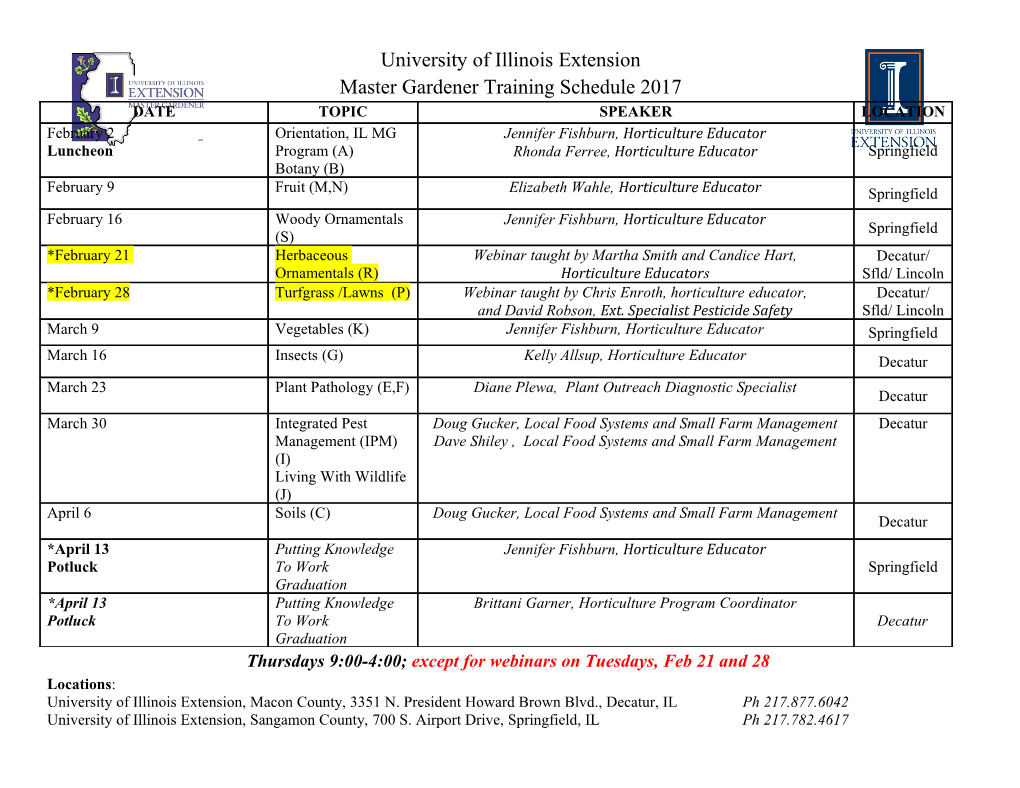
<p>Fundamentals Scribe: Myra Dennis Wednesday, September 16, 2009 (2nd hour) Proof: Ashley Brewington Dr. Miller PROTEIN SYNTHESIS Page 1 of 5</p><p>I. Protein Synthesis [S1] a. We’ll talk about non-ribosomal synthesis tomorrow. We’ll talk at great length about ribosomal synthesis because that’s what we all do in the animal kingdom, but there’s another protein synthesis that bacteria and fungi sometimes use to make materials such as cyclosporine, penicillin, gramacidin, a number of antibiotics. These are synthesized by a much different system (will talk about tomorrow). b. Protein synthesis basically comes down to how do you generate a polymer that has all the capabilities of a protein when you start out with a linear array of nucleotides. This was a big question in biology, microbiology, and biochemistry; everybody wanted to know how proteins were synthesized. It took about 5-10 years to work out this question. All this work was being done by Marshall Nuremberg and his colleagues – Dr. Miller saw this development. Alex Dounce talked about how bacteria synthesize proteins, though he was a little off target in terms of how mammalians synthesize proteins. We’ll talk about this later. c. Players in this game: mRNAs, tRNAs, amino-acyl tRNA synthetases, and ribosomes. d. mRNAs and tRNAs are the agents that really make the transformation between a nucleotide sequence and a peptide sequence (an amino acid sequence). So they are the ones that make the translation. And protein synthesis is called translation because you go from a nucleic acid polymer to an amino acid polymer. So it’s a totally different language that is developed in synthesizing a protein. e. We’ll look at look at mRNAs first, then will talk about tRNAs, then will talk about synthetases, then ribosomes, then eventually we’ll see how this whole thing is accomplished. II. Genetic Codes as They Occur in mRNA’s [S2] a. First of all, we have a code. There has to be a code to tell the system which amino acids go where and which amino acid is to follow which amino acid. And that code can be a variety of codes. It can be an overlapping code that requires 3 nucleotides to have a code. You can use this code in many ways. You can have a second amino acid put in by a code which operates by leaving the first nucleic acid out, and then incorporating 3 more. And then you can go on down the line and have a code that leaves the first 2 nucleotides out, and uses the 2nd, the 3rd, 4th, and the 5th for a code. This is the way many viruses and some bacteria synthesize their proteins, that’s how the code is attained because they use different phases of the nucleic acid polymer because they need more proteins than the total genome is capable of producing by using a non-overlapping code. So using an overlapping code allows you to have many more possibilities for protein synthesis than you would have with a non-overlapping code. b. A non-overlapping code is depicted here, and this is the type we use. We have the first 3 nucleotides are used to place one amino acid, then next 3 are used to place another one. There’s no overlap, every 3 nucleotides are utilized for 1 amino acid, and only 1 amino acid. So that basically is what the system calls for. c. We also have a continuous code, that is it is continuous without any interruptions, and there are no punctuations in a code for a human or mammalian. There’s not one code of 3 nucleotides that is used and then one nucleotide is skipped, then use the next 3, the one is skipped, and use the next 3. So there’s no interruption in the code, it’s continuous. It’s non-overlapping, and it’s continuous for all major organisms (animals, plants). d. Now that we know what we’re dealing with – a code system that has 4 different bases involved, and we use 3 of them each time. So we have total of 64 different codes. But we only have to have 20 amino acids placed, so that gives a system of degeneracy. III. General Structure of tRNA [S3] a. The tRNAs (which are used to identify the codes that are given in the mRNA – we’ve been talking about a mRNA carrying a code) are rather small nucleic acids that are about 75-80 nucleotides long. They are shaped in this cloverleaf form. They are bristling with identity factors. Identity factors can be all over the tRNA. Basically you have 5’ end of the nucleic acid which is capped by a phosphate group, and not much happens there, there’s very little going on at the 5’ end. What really counts is this 3’ end and the adenyl (I guess this is the same as “adenine”?) nucleotide at the very 3’ end. This is where the amino acid is going to be attached to the tRNA once the tRNA is charged with an amino acid. b. The other domains within a tRNA: the acceptor stem, some tRNAs are identified by the array of nucleotides in the acceptor stem. Some are identified by other arrangements within the entire molecule. But the molecule in general has some unique characteristics. It has some thimadine in it, which is rather strange for a nucleic acid of this type. And it has pseudo-uridine, and those are generally found in “this” particular loop. c. And the other loop, the D loop, has dihydrouridine in it, and it can have different sizes. It is a variable loop, which has all types of nucleotides. Fundamentals Scribe: Myra Dennis Wednesday, September 16, 2009 (2nd hour) Proof: Ashley Brewington Dr. Miller PROTEIN SYNTHESIS Page 2 of 5 d. Finally there’s the anti-codon, the 3 bases that are going to specify where that tRNA will sit on a mRNA. The genetic code that the messenger carries is a degenerate code, in the sense that there are several tRNAs for a given amino acid. So, very many tRNAs are used. e. Student question: Is that “ACC” on the 3’ end conserved? i. Yes, all tRNAs will have this particular sequence. Particularly the adenine base will be there. f. We have many tRNAs, and we have many codes, so a given amino acid can be placed in a polypeptide chain by recognizing different codes and by using different tRNAs. So that’s the degeneration system. We can have degeneracy because we have 64 different codes, but we really only need about 20 codes. So we can have a variety, we can have some wobbling (will talk about later on). So we can have some degeneracy there. IV. Major Identity Elements in Four tRNA Species [S4] a. Keep in mind that the next element of this whole system is going to the synthetases. This is a rudimentary diagram showing the identity elements for several types of tRNA. For instance, a tRNA for Phenylalanine (that means it would carry phenylalanine into the polypeptide chain, phenylalanine would be attached up here at the 5’ end of this tRNA. And this tRNA would be recognized as carrying phenylalanine by the synthetase enzyme because of this particular nucleotide here, and this one here, and the anitcodon at this point. b. And then the tRNA for methionine would be recognized by the synthetase in this fashion. c. And the tRNA for alanine would be recognized by nuclide specific right here, #3 and #70. d. And for serine by a large conglomerate of nucleotides in the stem as well as a couple of nucleotides in the D-loop. The reason I’m belaboring this is that every amino acid has only one synthetase. If there’s going to be methionine placed in a polypeptide chain there’s one synthetase that does that, and the synthetase must recognize all of the tRNAs that are going to carry methionine. And the synthetase looks at all those sequences of the nucleotides that identify the tRNA, and the tRNA is selected because the synthetase sees that particular configuration of nucleotides. V. Ribbon Diagram of tRNA Tertiary Structure [S5] a. This is where you can look at tRNA in its actual shape. The cloverleaf shape that you just looked at is pertinent for pedagogic and telling you how tRNAs work and how they look, but this is what the actual process leads to. We have 73, 76 or so nucleotides. b. You can trace down here from #1 to #10, then up here to #20, and then down to #32, then up to #42, up to #50, around to #60, and then to #70, and finally to 75, 76. So you can trace the tRNA and it’s better to look at it this way because when you realize how it’s going to be used, it is really carrying the amino acid that it’s going to transfer to the growing polypeptide chain right here. So it’s shaped in a way that it can hand off the amino acid to the growing polypeptide chain in the ribosome, and that’s the reason for the L shaped. And the anti-codon which allows it to go to a specific point on a mRNA is located right down here (at very bottom). So it’s like the system you had in the old days of mail carrying, when the mail baggage of a train would be hanging out in an L-shape from a platform, and the train could grab it right away as it passes down the line. So mechanically it’s a very good system to be built this way. You attain this system by taking this arrangement here and folding the T5C group over and bringing the D loop back underneath. Fold up the TC loop and fold down the D loop and finally obtain this kind of structure. VI. How Is an Amino Acid Matched with Its Proper tRNA? [S6] a. How does the system work? The system really depends on a second genetic code, and that second code is found in the aminoacyl-tRNA synthetases. Those large beasts (very complicated enzymes) are responsible for the accuracy and precision with which protein synthesis is done, because they have to discriminate between the tRNAs and the amino acids. So here you have a pool of 20 amino acids and a pool of hundreds of tRNAs, and the synthetase has to accept the right amino acid and the right tRNA and put them together and then edit and see if it’s properly done, and then release the tRNA to the ribosome. b. So it’s a monumental job - probably the most difficult task in biochemistry - to take (from a pool of amino acids) the proper amino acid and the proper tRNA. When you have multiple components of each factor, there are multiple amino acids and multiple tRNAs. Not only multiple tRNAs, but multiple tRNAs for the same amino acid. So a synthetase must take leucine synthetase (takes leucine and charges it on a tRNA) and will always take leucine, and only leucine. But then has a choice of about 5 different tRNAs to attach leucine to. So it’s doing a major job – basically what enables biosynthesis of proteins to proceed properly. VII. The Aminoacyl-tRNA Synthetase reaction [S7] a. Here’s what the synthetase does. At the top is the overall reaction, take an amino acid and the proper tRNA and using ATP, which is split twice: ATP to eventually AMP and pyrophosphate. So 2 high energy phosphate bonds are used in the process. And it will then esterify the amino acid to a tRNA molecule. Fundamentals Scribe: Myra Dennis Wednesday, September 16, 2009 (2nd hour) Proof: Ashley Brewington Dr. Miller PROTEIN SYNTHESIS Page 3 of 5 The amino acid is going to be attached to a tRNA molecule through its carboxyl group. In chemical terms, the amino acid has been esterified by a big huge tRNA molecule. That’s the overall reaction. b. The nitty gritty, looking at it step-by-step: first have the enzyme, ATP, and the amino acid. And the enzyme would split ATP into AMP and pyrophosphate and the amino acid is attached then to adenine monophosphate in a mixed anhydride bond. This is the remnants of ATP, it’s AMP, and the phosphoric acid here (on ATP) is now used to make an anhydride bond with the acid coming from the amino acid. So have a C double bond O, phosphate double bond. This is called a mixed anhydride. That is activating the amino acid, makes the amino acid very active. The amino acid is now bound (not by an ester bond) but by an anhydride bond - very high energy. What really makes that reaction go is that you have cleaved off two of the phosphates from ATP, and generated pyrophosphate, which is phosphate-phosphate. c. You can cleave that again which makes that reaction really go. Get cleavage of the second high energy phosphate bond. So 2 total high energy bonds have been used to make this anhydride bond. One of the reasons for that is the anhydride bond is going to be used to esterify the amino acid to the tRNA, and that’s going be used to transfer the amino acid to the growing peptide chain. The investment of energy is made right here by the use of 2 high energy phosphate bonds to make this amino acid anhydride. d. Once you have that anhydride, and the appropriate tRNA, the tRNA (if use Class I synthetases) is going to attack the anhydride bond by virtue of the oxygen at carbon #2 on the ribosome moiety. You get this nucleophilic attack where AMP is eliminated and you wind up with the amino acid esterified to the tRNA. This is the acid, this is the alcohol, and you have tRNA and the amino acid is hooked to the tRNA by the ribosome (2nd carbon atom) of the last nucleotide (adenine) in the tRNA at #73 or 76 or so. That’s not suitable ultimately, and when that happens there is a transfer of the ester from carbon #2 on the ribosome moiety to carbon atom number 3. Just an easy transfer. There’s another small enzyme that does that. e. The other possibility here is done by Class II synthetases. Certain amino acids are done by Class I, and some are done by Class II. (He said it’s not important for us to know which amino acids are involved in Class I or II). We do have 2 classes of synthetases. The second class has the esterification process go directly to carbon #3 on the ribose of the tRNA. That completes the esterification of the amino acid to the tRNA in one step rather than 2 steps if you use a Class II synthetase. f. We’ve charged the amino acid, and used that charging process to provide the energy for the esterification of the amino acid to a tRNA. Remember this is done by that synthetase that has recognized this amino acid and this tRNA. Because if it’s not done properly, don’t get the right sequence of amino acids in the polypeptide chain. So the synthetase has done a monumental job. Once these reactions are done, the synthetase has performed its duty and the tRNA is ready for acceptance into a peptide synthesizing system. VIII. Codon – Anticodon Pairing [S8] a. The way peptides are synthesized is that the tRNA, by virtue of its anticodon, will find its right place on a mRNA. The mRNA is depicted here as a long line going from the 5’ to the 3’ end. I’ve called this (on the left) amino end of the mRNA, and I call this the carboxyl end (on the right). I do this because the first amino acid placed on the message will be the N-terminus of the polypeptide chain. In other words, the polypeptide chain is synthesized from its N-terminus to its C-terminus. So the message is always read 5’ to 3’. So you go from the 5’ to the 3’ end and the first amino acid you put on the message is carried there by a tRNA and that will be the amino terminal amino acid of the polypeptide chain. b. What makes the system work is the coupling of the anticodon with the codon. The anticodon is now traveling in the 5’ to 3’ range. All nucleic acids when they’re associated side-by-side are anti-parallel. So the anticodon is presented from the tRNA in the 5’ to 3’ array. And the message of course is presented in the 5’ to 3’. That means there are different types, anti-parallel arrangements. The third base in the code on the mRNA is coupling with the 1st base on the anticodon. The second base in both the codon and anticodon are together, they never change. But the 1st base in the message is coupling with the 3rd base in the anticodon. We’re talking about the 1st, 2nd, and 3rd bases in the messenger and the anticodon. They are anti-parallel. So you have to keep that in mind as to how this coupling will be arranged. If you know the code, you don’t necessarily know the anticodon if you forget the anti-parallel arrangement. c. The number of tRNAs from each amino acid is not the same as the number of codons. This is because some anticodons can recognize numerous codons. This is part of the degeneracy system. And the specificity is determined primarily by the 1st two bases. IX. Wobble Position Rule [S9] a. It’s not really a hypothesis, actually a law. b. [Went back to slide 8] – The 3rd base of the codon and the 1st base of the anticodon provide the wobble position. The first 2 bases in codon and the last 2 bases of anticodon are very strict, very precise, very close union between the bases found in these particular positions. But here, there can be a little bit of play, a little “hanky-panky,” with respect to how these items come together. Fundamentals Scribe: Myra Dennis Wednesday, September 16, 2009 (2nd hour) Proof: Ashley Brewington Dr. Miller PROTEIN SYNTHESIS Page 4 of 5 c. The first 2 bases form a strong Watson-Crick base pair union, that’s with codons and anticodons. If the first base of the anticodon is either a C or an A, binding will be very specific. But if the first base of the anticodon is a U or a G, binding is less specific, and 2 different codons can be read by that tRNA. If the 1st base of the anticodon is inosine (a rare nucleotide), binding is even less specific, and 3 different anticodons can be read by that tRNA. Next slide shows what he means by this. X. Illustration of Rules [S10] a. If the first base of the anticodon is either C or A, then there will only be one codon read because of the strength of the C and G arrangement, and A and U. If the first base of the anticodon is a U or a G, then you can have a codon with A or G in the 3rd position of the codon, combining with U. And you can have you can C or U in the third base of the codon combining with G of the anticodon. So C or A in the anticodon (first position) will bind only with G or U in the third position of the codon. If you have U or G in the first position of the anticodon then the arrangement’s a little looser. U in the 1st position of the antidocon will take on A or G. G in the 1st position of the anticodon is very happy to combine with C or U. b. Now look at that. G and C in this arrangement is very strict and specific (1st row). No other arrangement will be accepted. However, we can have G and C coupling together happily in the wobble position. The difference is that the G here (2nd row, on right) is in the anticodon, and the C is in the codon. In the strict position, G has to be in the codon, and C in the anticodon – that comes out of the stereochemistry of how the message interacts with the tRNA, something we don’t need to go into. c. Keep this in mind - you have a wobble position that allows a great deal more flexibility. And have greater flexibility if inosine is the first base in the anticodon. It can interact with A, U, or C as the third base in the codon. d. Why has this come about? Proteins are synthesized at different rates. During the synthesis of a protein, every time you add a new amino acid to a polypeptide chain in a ribosome, you have to kick the old tRNA out. The tRNA that has brought in the preceding amino acid is no longer necessary, and that has to be thrown out of the ribosome. Throwing that out of the ribosome takes a little time, it’s an effort that involves another high energy phosphate bond from GTP rather than ATP. So if you want to really go “lickity-split!” and make protein really quickly, you will use tRNA that come in and don’t bind so tightly to the message. Because the less firmly it’s bound to the message, the easier it is to kick it out. So that’s the basic rational for that described here. e. The loose fit allows sufficient specificity in association, but also allows a quick release, rapid dissociation. I’ve talked to you about collagen synthesis. In your early years, you’re synthesizing collagen by the minute, all the time very rapidly, in order to have growing bone and virtually every organ in the body. In that case, the anticodons used are those that allow quick release. To make collagen synthesis, you will use anticodons that have the first base in the anticodon as U or G. So consequently you get ability to synthesize protein very quickly. Bacteria don’t have such restrictions. Bacteria can synthesize an amino acid protein in a few seconds. To make a collagen chain of 1,000 amino acids, it takes about 5-6 minutes even with anticodons that have G or U in first position. So we make proteins generally much slower than bacteria do. XI. Ribosomes [S11] a. Ribosomes are large globs (globules). b. Composed of 2 unequal units: a large component and a small component. c. Let’s talk about prokaryotes first. Prokaryotes have 2 unequal units. One is 30S. “S” means Svedberg constant, an indication of molecular weight. A smaller value of S means generally a lower molecular weight substance. A 30S subunit is the small one for a prokaryote and the 50S is the larger one for a prokaryote. d. For mammals, we have larger ribosomal unit. 40S units for the small one, and 60S for the larger ones. The combined units are roughly 70 or 80S for prokaryotes, and a total of 90 or a 100S for eukaryotes. Each of us has a system of ribosomes that resemble the prokaryotic ribosomes. There are in the mitochondria. Mitochondria have a series of genes, ribosomes, tRNAs which are used to synthesize proteins that are indigenous to mitochondria. One of the reasons for the hypothesis that our mitochondria are really symbiotic prokaryotes that somehow got involved with some cell types and gave rise to the lineage of eukaryotic cells. Mitochondria definitely have ribosomes that resemble more closely bacterial and lower organism ribosomes than the eukaryotic ribosomes. e. Let’s look at 30S prokaryotes ribosome. The 30S ribosome has 16S ribonucleic acids and 20 total proteins (not 20S, just 20). In a eukaryote, with the higher molecular weight ribosome, the ribonucleic acid is heavier (18S) and has 35 proteins. The large subunit (50S in the prokaryote) would have 23S and 5S ribonucleic acids, and the bigger eukaryotic one would have 28S and 5.8S ribonucleic acids, and a total of 50 proteins. So you can see that our ribosomes are much bigger and more complex. But the complexity really pales into simplicity. For the big piece (50S) of a mammalian ribosome, if you take the Fundamentals Scribe: Myra Dennis Wednesday, September 16, 2009 (2nd hour) Proof: Ashley Brewington Dr. Miller PROTEIN SYNTHESIS Page 5 of 5 ribonucleic acids there and the proteins, put them into a flask, they will reassemble themselves without any other input of energy or chemicals or manipulation, into a full functioning ribosome. Just by themselves. Big and small components work that way both for mammalian and prokaryotic ribosomes. It’s a very good demonstration of the self-assembly processes of large polymers. Many proteins, many different ribonucleic acid species, and they are self-assembled into ribosomes. f. The ribonucleic acid that is found in mammalian ribosomes comes from genes in the nucleolus. There’s a special arrangement whereby the ribosomal RNA molecules are synthesized from genes in nucleoli in mammals. It’s not the case in prokaryotes because don’t have a nucleus. g. Ribosomes are about 2/3 ribonucleic acid, and they make up about 20% of the cell’s mass. Most cells make proteins of one type or another. XII. Structure of the E. coli Ribosomal Subunits and 70S Ribosome [S12] a. Textbooks always fall into the notion that you can explain protein synthesis best by using the E. coli representation, the bacterial type of ribosome. So this is a picture of the bacterial ribosome, but all you need to do to transfer this knowledge to a mammalian ribosome is to enlarge it to a certain extent. b. The small piece has a variety of components. There’s a head and a base. The important thing for the small piece is the decoding center. That’s where the message is going to lie across the center and that’s where the tRNA is going to come in and be recognized as being the proper tRNA. The coding arrangement we just saw is going to take place right there in the small piece (small white hole in the top middle of the purple picture in the top right). c. The larger component of the ribosome has signal marks called L1, L7, L12. What really matters to us is this is where the peptidly transfer center is found. If you take these 2 pieces and put them together, this would be the coupling of 2 pieces to give you the full ribosome (picture in top left). And that’s looking at it from the backside of the small piece. If turn about 90 degrees (bottom right), will see that the small component interfaces with the large component. In the middle, there’s an opening through which the mRNA goes. The decoding center is going to be in the 30S ribosomal part here, and the transfer center is going to be in the heavy piece here, it makes sense because the tRNA is going to come in and be decoded and will stick across and hand its new amino acid to the large piece which has the transfer center where the new amino acid is going to be joined to the old polypeptide chain, and then the amino acid is going to exit the ribosome by this tunnel. XIII. Electron Micrograph of Polysomes [S13] a. Shows a polyribosome. This is a ribosome that’s called a polyribosome because there’s many ribosomes on a single message. All of these large spheres are the ribosomes, and they are traveling down along the message. As they go, they’re adding amino acids to them. Ultimately, can see the growing polypeptide chain which is now protruding from the ribosome. This is a nice arrangement where one message can be translated to synthesize many proteins - many peptides - just from one message, by using a train of ribosomes passing down that railway of the message.</p><p>END 47 MIN.</p>
Details
-
File Typepdf
-
Upload Time-
-
Content LanguagesEnglish
-
Upload UserAnonymous/Not logged-in
-
File Pages5 Page
-
File Size-