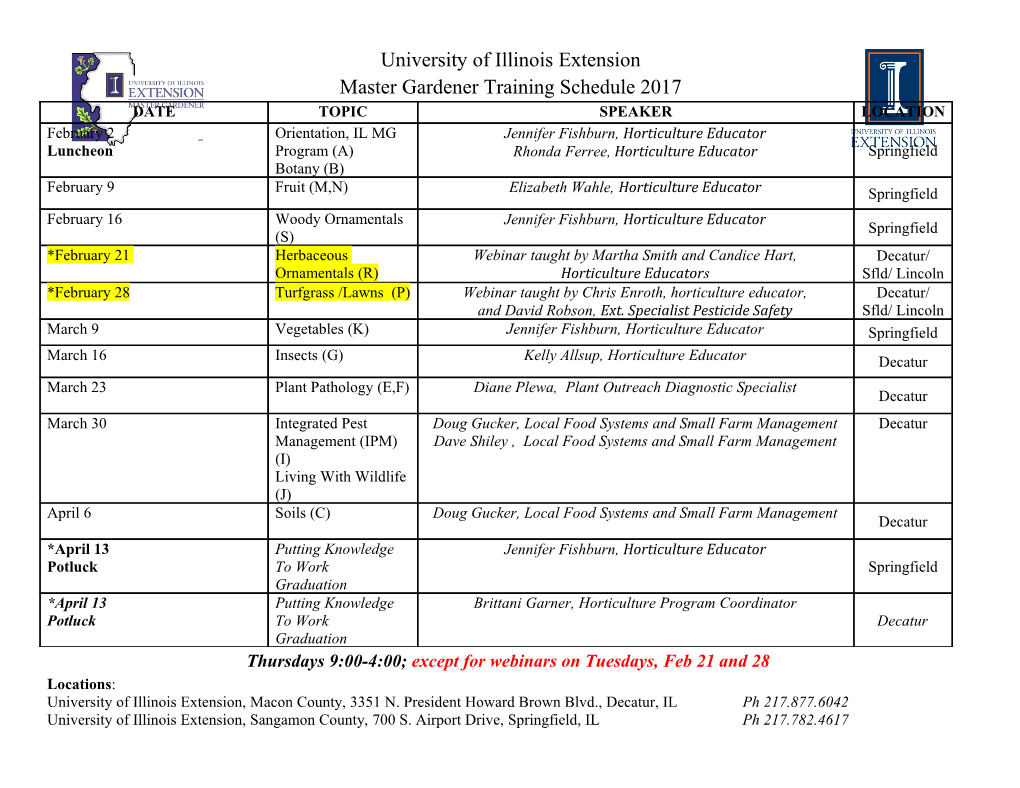
<p> 1Individual variability in the movement behaviour of juvenile Atlantic salmon</p><p>2Mathieu L. Roy1, André G. Roy1, James W. A. Grant2, Normand Bergeron3</p><p>31Département de géographie, Université de Montréal, 520 Côte Ste-Catherine, Montréal, </p><p>4QC, H2B 2V8, Canada.</p><p>52Department of Biology, Concordia University, 7141 Sherbrooke St. West, Montréal, QC,</p><p>6H4B 1R6, Canada.</p><p>73 INRS-Eau, Terre et Environnement, 490 rue de la Couronne, Québec, QC, G1K 9A9, </p><p>8Canada</p><p>9</p><p>10</p><p>11</p><p>12</p><p>13</p><p>14</p><p>15</p><p>16</p><p>17</p><p>18</p><p>19</p><p>20</p><p>21</p><p>22</p><p>23</p><p>1 1 2 24Abstract</p><p>25Stream-dwelling salmonid populations are generally thought to be composed of both </p><p>26relatively mobile and sedentary individuals, but this conclusion is primarily based on </p><p>27results obtained from recapture methods with low temporal resolution. In this study, the </p><p>28mobility of 50 juvenile Atlantic salmon was monitored using a large array of passive </p><p>29integrated transponder antennas buried in the bed of a natural stream. Fish locations were </p><p>30recorded at a high frequency for a period of three months in a 65 m reach. Four types of </p><p>31daily behaviour were identified: stationary (detected primarily at one location), sedentary </p><p>32(limited movement between a few locations), floater (frequent movements in a restricted </p><p>33home range) and wanderer (movements across the reach). Most individuals exhibited low</p><p>34mobility on most days, but also showed occasional bouts of high mobility. Between-</p><p>35individual variability accounted for only 12-17% of the variability in the mobility data. </p><p>36High mobility was more frequent at low flow, but no difference was observed between </p><p>37the summer (12-18oC) and the autumn (4-12oC). Individual variation on a daily basis </p><p>38suggested that movement behaviour is a response to changing environmental conditions </p><p>39rather than an individual behavioural trait. </p><p>40</p><p>41Keywords: Juvenile salmon, Mobility, Individual variability, Behaviour, Habitat. </p><p>42</p><p>43</p><p>44</p><p>45</p><p>46</p><p>3 2 4 47Introduction</p><p>48 Early studies depicted juvenile salmonids as sedentary, territorial animals </p><p>49exhibiting high site fidelity (Kalleberg 1958; Keenleyside 1962). The development of </p><p>50better tagging technology, which allowed for the tracking of individuals at a higher </p><p>51temporal resolution, revealed that the territorial mosaic of salmon parr was more flexible </p><p>52and dynamic than previously thought (Armstrong et al. 1999; Okland et al. 2004). In </p><p>53particular, Atlantic salmon parr have large, overlapping home ranges, with some </p><p>54individuals moving out of their home ranges to relocate either upstream or downstream </p><p>55(Okland et al. 2004; Ovidio et al. 2007), suggesting little fidelity to a particular </p><p>56microhabitat, sometimes undertaking habitat switches of several kilometres to lakes </p><p>57(Hutchings, 1986; Erkinaro et al. 1998) or estuaries (Cunjak et al. 1986).</p><p>58 It now seems broadly accepted that both sedentary and mobile individuals occur </p><p>59within a given population (Gowan et al. 1994; Rodriguez 2002; Morrissey and Ferguson </p><p>602011). While the size of the two fractions varies considerably between sites, species, and </p><p>61life stages, the sedentary fraction tends to be larger than the mobile fraction (e.g. </p><p>62Hesthagen 1988; Steingrimsson and Grant 2003). Some individuals have been </p><p>63characterized as “movers” (i.e. cruise foragers) whereas others as “stayers” (sit-and-wait </p><p>64foragers), based on the proportion of time spent moving (Grant and Noakes 1987; </p><p>65McLaughlin et al. 1994). Although spatial behaviour might be a heritable trait (Ferguson </p><p>66and Noakes 1983), Gowan et al. (1994) suggested that individuals may switch tactics in </p><p>67response to changing environmental conditions. Although some juvenile salmonids can </p><p>68defend the same territory for extended periods (Martel 1996), a fraction can switch </p><p>69between sedentary and mobile behaviour between two subsequent years (Harcup et al. </p><p>5 3 6 701984). However, it remains unclear how common this behaviour is, and at what temporal</p><p>71frequency the switching occurs. </p><p>72 Fish movements have been linked to changes in biotic and abiotic conditions </p><p>73(Gowan et al. 1994), likely due to variation in flow stage, temperature and daily light </p><p>74cycles. However, the effects of these variables on the behaviour of fish seem to be </p><p>75complex, as several studies have provided contrasting results. For instance, salmonids </p><p>76have been reported to decrease their mobility and territory size at high flows (Kemp et al. </p><p>772006), whereas others report the opposite trend (Scruton et al. 2003; Riley et al. 2009), or</p><p>78no trend at all (Berland et al. 2004; Heggenes et al. 2007). Similarly, while water </p><p>79temperature affects fish metabolism (Jonsson et al. 2001), its effect on fish activity is less </p><p>80certain (Fraser et al. 1993; Breau et al. 2007). As temperature drops in the autumn, </p><p>81Atlantic salmon parr suppress their daytime activity, presumably as a result of a tradeoff </p><p>82between growth and predation risk (Fraser et al. 1995; Johnston et al. 2004). </p><p>83Nevertheless, other proximate factors must also influence fish activity on a seasonal basis</p><p>84(Bremset 2000), as other studies report either no decrease or an increase in fish mobility </p><p>85(Nykanen et al. 2004; Riley et al. 2006).</p><p>86 The spatial arrangement of microhabitats might also influence mobility because </p><p>87habitat heterogeneity decreases territory size (Venter et al. 2008) and mobility (Heggenes </p><p>88et al. 2007). In less heterogeneous habitats, individuals might have to move farther to </p><p>89encounter complementary microhabitats that provide foraging opportunities and shelter </p><p>90(Venter et al. 2008). However, information on the relationship between habitat structure </p><p>91and fish mobility remains fragmentary. Furthermore, juvenile salmonids are often </p><p>92captured using methods that might be better suited for catching sedentary than mobile </p><p>7 4 8 93fish (Gowan and Fausch 1996). Therefore, if mobility affects habitat use, the estimation </p><p>94of habitat preference might be biased towards sedentary fish.</p><p>95 The results from movement studies depend on how frequently fish have been </p><p>96located and for what duration (Lucas and Baras 2000). For instance, fish mobility </p><p>97estimates from radio-telemetry studies are generally greater than those obtained from </p><p>98mark-recapture studies. However, radio-telemetry suffers from the inability to sample </p><p>99small fish and from relatively large sampling units of habitat, making difficult the </p><p>100quantification of small-scale movements. Recent developments in flat-bed passive </p><p>101integrated transponder (PIT) antenna grid provide fish tracking data at both high temporal</p><p>102and spatial resolutions over extended periods of time (Greenberg and Giller 2000; Riley </p><p>103et al. 2003). In this study, we used a large PIT antenna grid to monitor daily movement of</p><p>104a group of individually marked Atlantic salmon parr 1+ in a natural stream. Positions of </p><p>105tagged fish were recorded continually during three months of the summer and autumn. </p><p>106While previous studies have reported a high between-individual variation in parr mobility</p><p>107(Okland et al. 2004; Ovidio et al. 2007), within-individual mobility variation has received</p><p>108little attention. Hence, our primary objective was to document the magnitude of </p><p>109individual variation of parr daily mobility to test the competing predictions that </p><p>110individuals: (1) adopt consistent mobile or sedentary tactics over long periods of time; or </p><p>111(2) modify their mobility based on changing biotic and abiotic conditions. Second, if the </p><p>112data support the second prediction, we tested whether changes in behaviour could be </p><p>113predicted by environmental fluctuations. In particular, we tested the predictions that parr </p><p>114will be more sedentary: (3) when flow stage increases, as both the availability of drifting </p><p>115prey and swimming energy costs increase; (4) in the autumn than in the summer; and, (5) </p><p>9 5 10 116in heterogeneous habitats, which likely provide complementary feeding and sheltering </p><p>117habitats in closer proximity.</p><p>118</p><p>119Material and methods</p><p>120Study site</p><p>121This study was carried out on the Xavier Brook, a tributary of the Ste-Marguerite River in</p><p>122Saguenay, Québec, Canada (48°2591799 N; 69°5394899 W). The study reach, located </p><p>123425 m from the main river confluence, was approximately 65 x 10 m (length x width), </p><p>124composed of two pools separated by a steep riffle, providing high physical habitat </p><p>125diversity. In the thalweg at low stage (0.4 m3·s-1), depth ranged from approximately 0.1 m</p><p>126in the riffle to 1.65 m in the upstream pool. Median substrate size (B-axis, i.e. particle </p><p>127width) varied from gravel-cobble in the riffle to gravel-cobble in the deep portion of the </p><p>128pools and gravel-sand in the pool recirculation zones (substrate classification according to</p><p>129Wolman 1954).</p><p>130</p><p>131Fish tracking system</p><p>132To monitor fish movements, we used a large flatbed antenna grid covering the entire </p><p>133study reach. The system was used to monitor tagged fish locations in the reach during 97 </p><p>134days (24 July to 1 November 2008). The tracking system consisted of an array of 149 </p><p>135circular antennas (50 cm in diameter), which were buried within the river bed and </p><p>136designed to detect the presence of 23mm PIT tags (Texas Instruments (TIRIS) model RI-</p><p>137TRP-RRHP, 134 2 kHz) and other tags complying with the ISO 11784/11785 </p><p>138international standards. Each antenna was interrogated for fish presence every 34 s (i.e. </p><p>11 6 12 1390.03 Hz). Antennas were distributed along cross-channel transects each composed of five </p><p>140antennas. Overall, the detection field of the antenna grid covered 19% of the wetted area </p><p>141of the site at a discharge of 0.4 m3· s-1.</p><p>142 Each group of five antennas was linked to a tuning capacitor, which was wired to </p><p>143a CYTEK multiplexer (JX/256 series, mercury wetted 256 single poles relay, www.cytec-</p><p>144ate.com). The multiplexer was connected to an Aquartis controller (custom made by </p><p>145Technologie Aquartis; www.aquartis.ca) composed of a TIRIS S-2000 reader, a </p><p>146datalogger and a custom-made controller unit. The system was powered by three solar </p><p>147panels connected to four 6V batteries plugged in series and two 12V batteries plugged in </p><p>148parallel. Each antenna was activated successively for the detection of PIT tag presence. </p><p>149When a PIT tagged fish was detected, the date (dd/mm/yy), time (hh/mm/ss), antenna ID </p><p>150(multiplexer card and port number) and fish ID (tag number) were recorded. Detection </p><p>151range varied from 300-400 mm above the bed surface and 600-800 mm in diameter. </p><p>152During the study period, all antennas detected at least one fish. For more technical details </p><p>153on the antenna grid, see Johnston et al. (2009).</p><p>154</p><p>155Fish capture and tagging</p><p>156 We captured 69 Atlantic salmon parr (1+) in the study reach on two occasions </p><p>157using a backpack electrofishing device: 44 fish were caught on 24 July 2008, and 25 on </p><p>15828 August 2008. During the second electrofishing session, fish were captured </p><p>159immediately upstream of the reach to avoid re-capturing tagged fish. Only parr of body </p><p>160length >80 mm were kept for the experiment to avoid the potential negative effects of PIT</p><p>161tagging on parr survival and growth (Sigourney et al. 2005); smaller juveniles as well as </p><p>13 7 14 162juvenile brook trout (Salvelinus fontinalis) and longnose dace (Rhinichthys cataractae) </p><p>163were released at individual capture locations. Fish were then anesthetised in a clove oil </p><p>164solution (3 ml/10 L) and implanted with 23-mm PIT tags (Texas Instruments) in the </p><p>165abdominal cavity secured with surgical tissue adhesive (Vetbond©). Tagged fish were </p><p>166allowed a recovery period of approximately 2 hours in a fish tank before being released </p><p>167on the study site. A total of three fish died during tagging, two during the first tagging </p><p>168session and one during the second. Average fork length (L ± SD) and average mass (M± </p><p>169SD) of tagged fish were: LA: 98 ± 7.4 mm; MA= 9.7 ± 1.7 g; LB: 109 ± 8.3 mm; MB: 10.7 </p><p>170± 2.3 g. The fish captured in August were larger than those captured one month earlier </p><p>171(fork length t = -5.73, df =64, p < 0.001 (mass t = -1.92, df = 64, p=0.06).</p><p>172</p><p>173Habitat characterization</p><p>174 Flow stage and water temperature fluctuations were recorded every 15 min using </p><p>175a pressure transducer (Level logger) installed at the bottom of the upstream pool. Water </p><p>176stage was estimated by correcting the recorded pressure values for changes in </p><p>177atmospheric data obtained from the closest meteorological station, and then subtracting </p><p>178the minimum value observed during the study period. Therefore, stage was defined as the</p><p>179water level above the minimum summer low flow level. The study period was </p><p>180characterized by substantial discharge variability (Fig. 1). A high magnitude flow event </p><p>181occurred at the beginning of August, followed by a stage decrease in the following </p><p>182month. Then, a prolonged low flow lasted until the end of October when it was </p><p>183interrupted by several precipitation events. Base flow between these events was </p><p>184approximately 10 cm over the minimum flow, which corresponded roughly to the median</p><p>15 8 16 185flow recorded during the study period. Flow stage values were categorized as low (0-10 </p><p>186cm, 35% of days), medium (10-15 cm, 35% of days), high (15-25 cm, 13% of days) and </p><p>187very high (25 cm and higher, 17% of days). Using a field based digital elevation model of</p><p>188the reach, bankful flow was estimated to occur at a stage of 60 cm. </p><p>189 During the same period, water temperature decreased from 19.0oC to 2.8oC (Fig. </p><p>1901). From 24 July to 1 September, daily average water temperature fluctuated around </p><p>19115oC. After 1 September, water temperature decreased linearly. Water temperature </p><p>192reached 12oC on 9 September, which corresponds to the upper boundary of the </p><p>193temperature range at which parr suppress their daytime activity (Valdimarsson et al. </p><p>1941997). The study period was therefore divided into two periods: summer (12-18 oC) and </p><p>195autumn (4-12 oC). </p><p>196 Depth and bed roughness were also characterized in detail throughout the reach. </p><p>197Topography was surveyed using a robotic total station (Trimble 5600DR) by combining a</p><p>198systematic transect sampling approximately 1 m apart with the characterization of </p><p>199individual roughness elements that protruded approximately 10 cm above the local mean </p><p>200bed elevation. This strategy was adopted to optimize sampling effort, as sampling point </p><p>201density increased proportionally with bed complexity. From the total of 6250 sampled </p><p>202points, a digital elevation model was created using a triangular irregular network </p><p>203interpolation with pixel size of 10 cm. Topography was detrended for mean thalweg slope</p><p>204and water surface at median flow was subtracted to obtain flow depth. Therefore, </p><p>205variability of flow depth mainly reflected height variation induced by the riffle-pool </p><p>206channel morphology. Depth was not temporally adjusted to flow stage to reflect the use </p><p>207of specific habitats rather than specific depth values. This way, across flow stages, high </p><p>17 9 18 208depth use could be interpreted as the use of habitat located in a pool rather than be </p><p>209confused with habitats located in the riffle at a higher flow stage.</p><p>210 Bed roughness, expressed as the spatial standard deviation of bed elevation values</p><p>211of the DEM pixels included in a moving window of 65 cm2, was characterized by </p><p>212computing an index based on the estimate of local bed elevation variability. The size of </p><p>213the window was determined in order to characterize the roughness of most of the largest </p><p>214particles present on the reach. We focused on protuberance from the bed that might be </p><p>215more important in creating flow refuges and cover than average particle size. For </p><p>216instance, it is common to observe large particles buried in the bed that do not protrude </p><p>217higher above the average bed height than smaller particles (Nikora et al. 1998). The </p><p>218downstream pool exhibited the largest coherent region of high bed roughness, whereas </p><p>219the remainder of the reach showed an apparently random spatial pattern of bed roughness.</p><p>220For every antenna, mean depth at median flow and bed roughness were estimated by </p><p>221averaging all pixel values located in a circle matching the antenna detection range. Fish </p><p>222daily habitat use was then estimated by averaging the mean values associated with all </p><p>223visited locations weighted by the number of detections per antenna.</p><p>224</p><p>225Data analysis</p><p>226 Fish behaviour was characterized on a daily basis using four variables. The </p><p>227number of movements and the distance travelled provided estimates of fish mobility, </p><p>228whereas the number of sites visited and the extent of the reach used by fish gave estimates</p><p>229of home range size. We defined a fish movement as a change of fish location (antenna): </p><p>230i.e. every time a fish was detected at two different locations successively, a movement </p><p>19 10 20 231was recorded. The number of movements was an indicator of activity that did not take </p><p>232into account the distance between locations. In contrast, the daily distance travelled was </p><p>233defined as the sum of the distance (m) between each antenna successively visited. The </p><p>234number of sites represented the number of different antenna locations where a fish was </p><p>235detected in a day. However, despite a high spatial coverage and a high temporal sampling</p><p>236frequency of the tracking system, fish could sometimes travel between two distant </p><p>237locations without being detected by antennas located in between. Hence, the variable </p><p>238extent fills this gap by representing a home range length, or the distance between the two </p><p>239most distant locations visited by a fish in a day.</p><p>240 We used a principal component analysis (PCA) on the daily mobility variables to </p><p>241describe the variability of every fish. Prior to the PCA, each variable was normalized </p><p>242(log10+1) and standardized. Then, based on the mobility variables, fish behaviour was </p><p>243classified using a k-means clustering algorithm. The correct number of behavioural types </p><p>244(clusters) was determined by comparing silhouette values between three and five </p><p>245behavioural types (Kaufman and Rousseeuw, 1990) </p><p>246 The frequency of occurrence of behavioural types per individual that spent six </p><p>247days or more in the study reach was examined. Then, the components of variance of the </p><p>248four mobility variables were estimated using an additive-variance component model, </p><p>249using individuals as a random factor, in which yij (mobility of fish I on day j) = µ+αi+εij where µ is </p><p>250the mean of the population, i, deviation from the mean of the ith fish (i=1 to 24) εij, is the</p><p>251residuals containing the intra-individual variation. To meet the model assumptions, </p><p>252transformed variables were used (log10+1). However, the descriptive statistics shown in </p><p>253the figures and tables are based on non transformed data. </p><p>21 11 22 254To examine the temporal variability of fish behaviour, the proportion of behavioural </p><p>255types adopted by each individual was plotted on a time series. Then, the frequency of </p><p>256occurrence of behavioural types was examined in relation to flow-stage categories and </p><p>257season. A generalized estimation equation (GEE) approach was used to describe the </p><p>258observed and expected occurrence of a behavioural type as a function of flow stage and </p><p>259season. GEEs are an extension of generalized linear models that accommodate repeated </p><p>260measurement of the same individuals and a categorical response variable (Diggle et al. </p><p>2612002). Therefore, the variable days was used as a repeated measure, fish as subjects, flow </p><p>262stage and temperature as fixed factors and behavioural types as a dependent categorical </p><p>263variable. GEEs were performed by SPSS 17 © (SPSS Inc. Chicago, Illinois) using a </p><p>264Poisson distribution with a log link and repeated measurement covariance structure set to </p><p>265first order autoregressive to account for temporal dependence between successive days. </p><p>266Similarly, to examine differences in habitat use in terms of depth and bed roughness in </p><p>267relation to behavioural types, two distinct mixed-effects models were performed using </p><p>268fish as subjects, days as repeated measures, behavioural types as a fixed factor and depth </p><p>269and roughness as a dependant variable. Again, first order autoregressive covariance of the</p><p>270repeated measurements was chosen. Best suited models were selected based on lowest </p><p>271Akaike’s information criterion (AIC) and quasi AIC (QIC) values (Burnham et al. 2011). </p><p>272For both types of statistical models, the effect of fish mass and length was tested. No </p><p>273significant effect was observed (p > 0.05), perhaps because of a low statistical power </p><p>274resulting from the need to treat the fish from the two tagging periods separately and of the</p><p>275overall low variability of values observed among fish. Therefore, mass and length were </p><p>276not incorporated in the models.</p><p>23 12 24 277</p><p>278Results</p><p>279Fish tracking</p><p>280 Of the 66 fish that were PIT-tagged and released in the reach, 4 individuals (6%) </p><p>281were never detected by the tracking system and 12 individuals (18%) were detected for </p><p>282either less than 24 hours following release or less than three hours in a single day. These </p><p>283individuals were not included in further analyses. Of the remaining fish, 10 individuals </p><p>284(15%) were detected in the reach during a single day, 16 individuals (24%) were detected</p><p>285for 1 to 5 days and 24 individuals (37%) remained between 6 and 70 days in the reach.</p><p>286</p><p>287Behavioural types</p><p>288 The daily distance travelled, the number of movements, the number of sites visited</p><p>289and the extent of the reach used by fish were positively correlated, which allowed for data</p><p>290reduction. Indeed, 90% of the variability was explained by the two first axes of a PCA </p><p>291(Table 1). The primary ordination axis (PCA1), which accounted for 70% of the </p><p>292variability, was positively correlated with all mobility variables, but was least strongly </p><p>293correlated with extent (Fig. 2). Therefore, low values of PCA1 represented lower mobility</p><p>294in smaller home ranges, whereas higher values represented higher mobility in larger </p><p>295home ranges. In contrast, the secondary axis explained 20% of the variability and was </p><p>296negatively correlated to the number of movements and positively correlated to the extent </p><p>297of fish movement. Data ordination illustrated the high variability of overall mobility </p><p>298exhibited by fish during the entire study period (Fig.2.).</p><p>25 13 26 299 Based on a cluster analysis, fish spatial behaviour was categorized into four types:</p><p>300Stationary, Sedentary, Floater and Wanderer (Fig. 2). Stationary behaviour was </p><p>301characterized by low mobility, being detected most often by a single antenna (Table 2). </p><p>302Fish 15 on Day 26 (Aug 18) adopted typical stationary behaviour (Fig. 3). On some </p><p>303occasions, stationary behaviour also included the use of more than one location during </p><p>304the day. However, these locations were adjacent to each other and no back and forth </p><p>305movements were observed.</p><p>306 When fish were detected at a few locations in a day, their behaviour was </p><p>307characterized as sedentary (Table 2). Fish exhibiting sedentary behaviour travelled on </p><p>308average 10m daily and moved three to four times between locations for an average extent </p><p>309of 5.7 m. For example, on Day 61 (22 Sep), Fish 50 exhibited typical sedentary behaviour</p><p>310by using four locations located throughout half the channel length and moved only once </p><p>311between each location (Fig. 3). </p><p>312 Cluster analysis also discriminated two types of higher mobility behaviour (high </p><p>313PCA1 scores) along the extent-number of movement gradient (PCA2 axis) (Fig. 2). When </p><p>314individuals used a relatively restricted home range (average extent: 5.7m), but made </p><p>315many movements between locations (mean =36), their behaviour was defined as floater </p><p>316(Table 2). For instance, Fish 37 on Day 32 (24 Aug) was detected at only five nearby </p><p>317locations in the downstream pool, but switched 34 times between these locations (Fig. 3). </p><p>318During the study period, the most extreme floater made 525 movements, resulting in a </p><p>319daily travelled distance of 2449 m on an extent of 5.3 m.</p><p>320 In contrast, when a fish exhibited a high distance travelled (avg: 115 m), but over </p><p>321a larger extent (avg: 25.3 m), their behaviour was defined as wanderer (Table 2). Typical </p><p>27 14 28 322wanderer behaviour involved travelling across the entire reach, from one pool to the </p><p>323other. Wanderer behaviour was characterized by a similar number of sites visited as the </p><p>324floater. However, the number of movements between locations was generally lower and </p><p>325the locations visited were farther away. The number of sites visited by fish adopting </p><p>326wandering behaviour was not higher than for floaters, likely because individuals moving </p><p>327long distances travelled rapidly and were thus difficult to detect. For example, on Day 61 </p><p>328(22 Sep), Fish 66 travelled from the downstream pool almost to the upstream pool, then </p><p>329back again, but was detected at only 11 sites for a total of 16 movements (Fig. 3).</p><p>330</p><p>331Individual variability in behaviour</p><p>332 Individuals exhibited a variety of types of mobility behaviour rather than </p><p>333‘specializing’ on one type over the study period. Among the 26 individuals that were </p><p>334detected on the site for less than six days, their behaviour was sedentary, stationary and </p><p>335wanderer on average for 36%, 30% and 30% of their time, respectively (Fig. 4a). Floater </p><p>336behaviour was only observed in five fish, which represented on average 8% of their time </p><p>337(Fig. 4a).</p><p>338 Among the individuals that stayed more than six days on the study site, high intra-</p><p>339individual variability of behaviour was observed (Fig. 4b). Out of 24 individuals, 15 </p><p>340showed all four types of behaviour during the study period. Low-mobility behaviour was </p><p>341most frequently observed, as individuals were sedentary and stationary for 33% and 28% </p><p>342of the days, respectively, during which they were detected in the reach. Floater and </p><p>343wanderer behaviour were less frequent, with an average of 19% and 20% of the days, </p><p>344respectively. However, six fish were more mobile than the others, exhibiting floater or </p><p>29 15 30 345wanderer behaviour for more than 50% of the days. For fish that stayed more than six </p><p>346days, there was no significant trend between the duration in the reach and the proportion </p><p>347of days each behaviour type was adopted by an individual (p > 0.1).</p><p>348 All fish were sedentary most of the time, but many individuals exhibited </p><p>349occasional bouts of high mobility. Indeed, all fish that stayed more than 6 days in the </p><p>350reach showed a low median distance travelled, but most moved more than 90 m. The </p><p>351daily number of movements displayed a similar pattern, with a relatively low median </p><p>352number of movements and numerous extreme values. Although the number of sites and </p><p>353the extent did not show as many extreme values, there was high intra-individual </p><p>354variability. Decomposing the components of variation of the four mobility variables </p><p>355indicated that intra-individual variation accounted for 83 to 88% of the total variation, </p><p>356compared to 12 to 17% for the inter-individual variation (Table 3). Similarly, average </p><p>357ordination scores for individuals that stayed more than six days suggested that a </p><p>358considerable number of individuals had relatively similar average mobility (Fig. 2). For </p><p>359instance, 17 individuals (70%) had their average ordination scores categorized as </p><p>360sedentary while the remaining fish was categorized as floater or wanderer. </p><p>361</p><p>362Temporal variability</p><p>363 The frequency of behaviour exhibited over the course of the season suggested that on </p><p>364most days, a mixture of behavioural types was observed (Fig. 5). Following the tagging </p><p>365of 42 parr on 24 July, 14 individuals were present on the reach. The number of </p><p>366individuals dropped drastically on 4 Aug. following a major flood event, then fluctuated </p><p>367between 4 and 8 before the second tagging session on 28 Aug., after which the number </p><p>31 16 32 368peaked at 22 and then constantly decreased until the end of the observation period. </p><p>369Despite the variability of behaviour observed on a daily basis, some periods were </p><p>370dominated by specific behaviour types. For instance, between 14 Aug. and 28 Aug., </p><p>371wandering behaviour was observed only four times, whereas most fish exhibited </p><p>372wandering behaviour from Day 16 Oct. to 20 Oct. Similarly, from day 22 Aug. to 29 </p><p>373Aug., floater behaviour was most frequent.</p><p>374 Examining the frequencies of occurrence of behavioural types in relation to flow </p><p>375stage and season using GEE showed a general decrease in mobility with increasing flow </p><p>376stage (Wald χ2=7.974, df=3, p=0.047). Pooled frequencies of occurrence illustrated an </p><p>377increase in the proportions of sedentary behaviour from 30% to 45% with an increase in </p><p>378flow stage (Fig. 6). Conversely, wandering behaviour decreased from 22% of occurrence </p><p>379to 7% from low flow to a very high flow. Over the season, parr used slightly different </p><p>380habitats in terms of depth when adopting different behavioural types (F = 5.46, df = </p><p>3813,514, p = 0.001). Daily average depth used was 0.2 m higher for the fish exhibiting </p><p>382floater behaviour than the other behavioural types (Fig. 7a). However, this trend was </p><p>383observed for only five individuals (Fig. 7b). In fact, a mixed effect model accounting for </p><p>384individual and temporal dependence indicated that wanderer behaviour was associated </p><p>385with lower depths used than the three other behavioural types (confidence interval on </p><p>386depth difference, df = 222-249, p < 0.03 for all comparisons, Bonferroni adjustments). In</p><p>387contrast, no difference in bed roughness used was observed for the different behaviour </p><p>388types (F = 1.235, df = 3, 549, p = 0.296).</p><p>389</p><p>390Discussion</p><p>33 17 34 391 In this study, most individuals exhibited low mobility (stationary and sedentary </p><p>392behaviour) on most days, but most individuals also showed occasional bouts of high </p><p>393mobility, either by carrying out frequent movements in a relatively restricted area </p><p>394(floater) or by travelling across the reach, from one pool to the other (wanderer). Our </p><p>395results suggest that most fish in the reach switched behaviour on a daily basis.</p><p>396 Daily behaviour switching contrasts with the common view that fish population </p><p>397are composed of fractions of sedentary and mobile individuals (Rodriguez 2002, </p><p>398Morrissey and Ferguson 2011). However, comparisons of behavioural categories across </p><p>399study designs and spatial scale is complicated. Telemetry and recapture techniques can </p><p>400provide a very large spatial scale, which is useful to characterize habitat switches </p><p>401sometimes involving migrations of several kilometres to lacustrine (Hutchings 1986, </p><p>402Ryan 1986) or estuarine environments (Cunjak et al. 1989). Our relatively restricted </p><p>403study area (65 m reach) most likely underestimated the mobility of fish exhibiting </p><p>404wandering behaviour. Nevertheless, reach extent likely had a minor effect on the majority</p><p>405of fish, which adopted sedentary behaviour most of the time and therefore should not </p><p>406affect our conclusion about intra-individual variability in behaviour. Hence, our proposed</p><p>407classification of mobility behaviour is dependent on the spatial scale of the study design, </p><p>408which focused on the fine scale movements of parr in a single reach. </p><p>409 Most studies confirming the presence of mobile and sedentary fractions of a </p><p>410population have used recapture techniques with relatively low temporal sampling </p><p>411frequency (e.g. Heggenes et al. 1991; Roghair 2005). Such techniques require sampling </p><p>412over a long duration to obtain individual variability without underestimating fish </p><p>413mobility. For instance, if an individual moves 40 meters upstream over a short period of </p><p>35 18 36 414time and then back to its original location, the following recapture could lead to the </p><p>415biased conclusion of sedentary behaviour Rather than being strictly sedentary or mobile, </p><p>416individual brown trout switched behaviour over the course of a two-year study (Harcup et</p><p>417al. 1984). Our data support a similar flexibility of the mobile or sedentary fractions, but </p><p>418over shorter time scales.</p><p>419 Generally, there was less variation in behaviour when environmental conditions </p><p>420were more homogeneous. Despite a baseline amount of variation, some types of </p><p>421behaviour tended to dominate during particular periods of environmental stasis (i.e. more </p><p>422sedentary at high flow, more floating at low flow). Furthermore, the most drastic </p><p>423environmental fluctuations might have triggered changes in behaviour. For instance, the </p><p>424steady declines in water temperature from 16-20 October were accompanied by </p><p>425wandering behaviour by most fish. Perhaps, this was the trigger that winter or spawning </p><p>426is imminent, leading to a search for overwintering habitat by females and spawning </p><p>427opportunities for males. Similarly, during the major floods the few fish that were detected</p><p>428were sedentary rather than mobile, and the most sedentary fish were probably even not </p><p>429detected.</p><p>430 Salmon parr in our study exhibited a decrease in mobility with an increase in flow</p><p>431stage. Similar results have been observed in previous studies (Kemp et al. 2006), whereas</p><p>432others found no effect of flow stage on mobility (Robertson et al. 2004; Heggenes et al. </p><p>4332007). Because salmon parr are well adapted to using flow refuges to maintain station on </p><p>434the bed, most of the increased swimming costs that accompany higher flows (Hill and </p><p>435Grossman 1993) are likely to be associated with foraging movements or longer range </p><p>436movements from one foraging location to another (Liao 2007). Moreover, when </p><p>37 19 38 437velocities are higher, parr tend to reduce their foraging territory size in response to the </p><p>438increased swimming costs and prey density (Hughes and Dill 1990; Piccolo et al. 2008).</p><p>439 We found no difference in movement behaviour between the summer and autumn,</p><p>440despite the decrease in temperature. A decrease in mobility was expected due to a </p><p>441decrease in metabolic rate (Jonsson et al. 2001) and the expected decrease of diurnal </p><p>442activity (Fraser et al. 1993). However, parr can remain active even when water </p><p>443temperature is close to zero (Bremset 2000). Indeed, a radio-telemetry study showed that </p><p>444parr home ranges were as large during the autumn as during the summer (Okland et al. </p><p>4452004). Furthermore, a recent study undertaken under similar temperature ranges reported </p><p>446a higher mobility of parr in the winter (6.6-10.8 oC) than during the autumn (10.7-14.3oC)</p><p>447(Riley et al. 2006). The authors suggested that this behaviour might be unique to </p><p>448groundwater fed systems. Taken together with previous studies, our results suggest that </p><p>449mobility can remain relatively high even when water temperatures are low. In this study, </p><p>450the effect of lower metabolism on movement might have been offset by several factors </p><p>451including a change from sit and-wait drift foraging to benthic cruise foraging due to a </p><p>452decrease in drift abundance (Nislow et al. 1998). Interestingly, all individuals adopted </p><p>453wandering behaviour on two days in mid-October close to spawning season when </p><p>454temperature was between 4 and 6oC. The presence of spawning adults passing through the</p><p>455site may have increased the mobility of tagged fish, particularly the precocious parr.</p><p>456 We hypothesized that individuals would be more sedentary in shallow and </p><p>457heterogeneous habitats because high habitat heterogeneity is more likely to provide </p><p>458complementary feeding and sheltering habitats close together (Johnston et al. 2010) and </p><p>459because territory size tends to decrease with habitat heterogeneity (Kemp et al. 2005; </p><p>39 20 40 460Dolinsek et al. 2007; Venter et al. 2008). Our results did not support this prediction. </p><p>461However, for five individuals that remained over forty days in the reach, deeper habitats </p><p>462were associated with floater behaviour. Finding mobile fish in pools is in agreement with </p><p>463the assumption that foraging fish occupy a larger territory in lower velocity areas </p><p>464(Hughes and Dill 1990). Furthermore, although the term floater tends to refer to </p><p>465individuals deprived of a territory, such behaviour could be associated with multiple </p><p>466central place foraging, where fish frequently switch from one foraging territory to an </p><p>467adjacent one (Steingrimsson and Grant 2008). Nevertheless, when fish adopted wanderer </p><p>468behaviour, they used slightly shallower habitats than when adopting other behavioural </p><p>469types. These results contrast with our hypothesis and with previous observations on adult </p><p>470grayling (Thymallus thymallus (Nykanen et al. 2004). The presence of mobile fish in </p><p>471deeper areas might have implications for the accuracy of abundance surveys. As the </p><p>472catchability of juvenile salmonids decrease with mobility (Crozier and Kennedy, 1994), </p><p>473electrofishing might underestimate abundance in pools, which could be mistakenly </p><p>474considered as low quality or unused habitats (Linnansaari et al. 2010).</p><p>475 One possible mechanism explaining our observation of mostly sedentary </p><p>476behaviour interrupted by frequent bouts of high mobility might be the fish’s need to </p><p>477‘sample’ their spatially and temporally variable environment to evaluate drift abundance. </p><p>478Being aware of the quality of alternative habitats, an assumption of the ideal free </p><p>479distribution, is critical to determine which habitats or strategy will generate the greatest </p><p>480energy intake and growth (Power 1984), within the constraints of dominance hierarchies </p><p>481and predation risk.</p><p>41 21 42 482 In summary, the present study showed that Atlantic salmon parr are sedentary on </p><p>483most days, but also exhibit infrequent bouts of higher mobility. Movement behaviour </p><p>484appears to be plastic, allowing individuals to adapt to changing environmental conditions </p><p>485(Gowan et al. 1994). Even though movement behaviour was linked to variation in flow </p><p>486stage, a high between- and among intra-individual variation was observed, suggesting </p><p>487individuals undertake movements in reaction to other proximate factors operating at </p><p>488shorter time scales. Several studies on fish behaviour, movement and habitat use have </p><p>489reported a high inter-individual variation (Okland et al. 2004; Ovidio et al. 2007). </p><p>490However, studies are often conducted for a shorter duration and at a lower temporal </p><p>491frequency than our study. Furthermore, intra-individual variability is often overlooked by </p><p>492averaging values to estimate home ranges over the entire study period. Therefore, </p><p>493differences in individual behaviour may decrease as study duration increases. Because </p><p>494Atlantic salmon parr exhibit relatively high mobility, maintaining connectivity between </p><p>495different habitats (i.e. pools and riffles) should be considered a priority in salmon </p><p>496conservation practices.</p><p>497</p><p>498Acknowledgements</p><p>499 We would like to thank Francis Bérubé and Marc-André Pouliot for their help in </p><p>500the development of the tracking system and for essential logistical and technical </p><p>501assistance, and Jordan Rosenfeld for interesting discussions. We are also grateful to </p><p>502Patricia Johnston, André Boivin, Claude Gibeault, Marie-Êve Roy and René Roy for their</p><p>503help in the field and Geoide, NSERC and the Canada Research Chair Program for the </p><p>43 22 44 504funding of this research. Three anonymous reviewers provided useful comments that </p><p>505substantially improved the paper.</p><p>506</p><p>507References</p><p>508Armstrong, J.D., Huntingford, F.A., and Herbert, N.A. 1999. Individual space use </p><p>509 strategies of wild juvenile Atlantic salmon. J. Fish Biol. 55: 1201-1212.</p><p>510Berland, G., Nickelsen, T., Heggenes, J., Okland, F., Thorstad, E.B., and Halleraker, J. </p><p>511 2004. Movements of wild Atlantic salmon parr in relation to peaking flows below </p><p>512 a hydropower station. River Res. Appl. 20: 957-966.</p><p>513Bovee, K.D. 1982. A guide to stream habitat analysis using the instream flow incremental</p><p>514 methodology. Fish Wildl. Serv. FWS/OBS-82/26. </p><p>515Breau, C., Weir, L.K., and Grant, J.W.A. 2007. Individual variability in activity patterns </p><p>516 of juvenile Atlantic salmon (Salmo salar) in Catamaran Brook, New Brunswick. </p><p>517 Can. J. Fish.Aquat. Sci. 64: 486-494.</p><p>518Bremset, G. 2000. Seasonal and diel changes in behaviour, microhabitat use and </p><p>519 preferences by young pool-dwelling Atlantic salmon, Salmo salar, and brown </p><p>520 trout, Salmo trutta. Environ. Biol. Fish. 59: 163-179.</p><p>521Burnham K.P., Anderson, D.R., and Huyvaert, K.P. 2011. AICc model selection in the </p><p>522 ecological and behavioral sciences: some background, observations and </p><p>523 comparisons. Behav Ecol Sociobiol. 65: 23-35.</p><p>524Crozier, W.W. and Kennedy, G.J.A. 1994. Application of semi-quantitative electrofishing</p><p>525 to juvenile salmonid stock surveys. J. Fish Biol., 45: 159–164.</p><p>45 23 46 526Cunjak, R.A. , Chadwick , E.M.P. , & Shears , M. 1989. Downstream Movements and </p><p>527 Estuarine Residence by Atlantic Salmon Parr (Salmo Salar). Can. J. of </p><p>528 Fish.Aquat. Sci. 46: 1466 – 1471.</p><p>529Erkinaro, J., Niemelä, E., Saari, A., Shustov, Yu., and Jørgensen, L. 1998. Timing of </p><p>530 habitat shift by Atlantic salmon parr from fluvial to lacustrine habitat: analysis of </p><p>531 age distribution, growth and scale characteristics. Can. J. of Fish.Aquat. Sci. 55: </p><p>532 2266-2273.</p><p>533Diggle, P.J., Heagerty, P., Liang, K.-Y., and Zeger, S.L. 2002. Longitudinal data analysis.</p><p>534 Oxford University Press, U.K.</p><p>535Dolinsek, I.J., Grant, J.W.A., and Biron, P.M. 2007. The effect of habitat heterogeneity </p><p>536 on the population density of juvenile Atlantic salmon salmo salar l. J. Fish Biol. </p><p>537 70: 206-214.</p><p>538Ferguson, M.M., and Noakes, D.L.G. 1983. Movers and stayers - genetic-analysis of </p><p>539 mobility and positioning in hybrids of lake charr, Salvelinus namaycush, and </p><p>540 brook charr, S. fontinalis (Pisces, Salmonidae). Behav. Genet. 13: 213-222.</p><p>541Fraser, N.H.C., Heggenes, J., Metcalfe, N.B., and Thorpe, J.E. 1995. Low summer </p><p>542 temperatures cause juvenile Atlantic salmon to become nocturnal. Can. J. Zoolog. </p><p>543 73: 446-451.</p><p>544Fraser, N.H.C., Metcalfe, N.B., and Thorpe, J.E. 1993. Temperature-dependent switch </p><p>545 between diurnal and nocturnal foraging in salmon. P. Roy. Soc. B-Biol. Sci.252: </p><p>546 135-139.</p><p>47 24 48 547Gowan, C., and K. D. Fausch. 1996. Mobile brook trout in two high-elevation Colorado </p><p>548 streams: re-evaluating the concept of restricted movement. Can. J. Fish. Aquat. </p><p>549 Sci. 53:1370-1381.</p><p>550Gowan, C., Young, M.K., Fausch, K.D., and Riley, S.C. 1994. Restricted movement in </p><p>551 resident stream salmonids - a paradigm lost. Can. J. Fish. Aquat. Sci. 51: 2626-</p><p>552 2637.</p><p>553Grant, J.W.A., and Noakes, D.L.G. 1987. Movers and stayers: foraging tactics of young-</p><p>554 of-the-year brook charr, Salvelinus fontinalis. J. Anim. Ecol. 56: 1001-1013.</p><p>555Greenberg, L.A., and Giller, P.S. 2000. The potential of flat-bed passive integrated </p><p>556 transponder antennae for studying habitat use by stream fishes. Ecol. Freshw. Fish</p><p>557 9: 74-80.</p><p>558Harcup, M.F., Williams, R., and Ellis, D.M. 1984. Movements of brown trout, Salmo </p><p>559 trutta L., in the River Gwyddon, South Wales. J. Fish Biol. 24: 415-426.</p><p>560Heggenes, J., Northcote, T.G., and Peter, A. 1991. Spatial stability of cutthroat trout </p><p>561 (Oncorhynchus clarki) in a small, coastal stream. Can. J. Fish. Aquat. Sci. 48: </p><p>562 757-762.</p><p>563Heggenes, J., Omholt, P.K., Kristiansen, J.R., Sageie, J., Okland, F., Dokk, J.G., and </p><p>564 Beere, M.C. 2007. Movements by wild brown trout in a boreal river: Response to </p><p>565 habitat and flow contrasts. Fisheries Manag. Ecol. 14: 333-342.</p><p>566Hesthagen, T. 1988. Movements of brown trout, Salmo trutta, and juvenile Atlantic </p><p>567 salmon, Salmo salar, in a coastal stream in northern Norway. J. Fish Biol. 32: </p><p>568 639-653.</p><p>49 25 50 569Hill, J., and Grossman, G.D. 1993. An energetic model of microhabitat use for rainbow-</p><p>570 trout and rosyside dace. Ecology. 74: 685-698.</p><p>571Hughes, N.F., and Dill, L.M. 1990. Position choice by drift-feeding salmonids: Model </p><p>572 and test for Arctic grayling (Thymallus arcticus) in subarctic mountain streams, </p><p>573 interior Alaska. Can. J. Fish. Aquat. Sci. 47: 2039-2048.</p><p>574Hutchings, J.A. 1986. Lakeward migrations by juvenile Atlantic salmon, Salmo salar, </p><p>575 Can. J. Fish. Aquat. Sci. 43: 732-741.</p><p>576Johnston P., and Bergeron N. 2010. Variation of juvenile Atlantic salmon (Salmo salar) </p><p>577 body composition along sedimentary links. Ecol. Freshw. Fish. 19: 187-196.</p><p>578Johnston, P., Bergeron, N.E., and Dodson, J.J. 2004. Diel activity patterns of juvenile </p><p>579 Atlantic salmon in rivers with summer water temperature near the temperature-</p><p>580 dependent suppression of diurnal activity. J. Fish Biol. 65: 1305-1318.</p><p>581Johnston, P., Bérubé, F., and Bergeron, N.E. 2009. Development of a flatbed passive </p><p>582 integrated transponder antenna grid for continuous monitoring of fishes in natural </p><p>583 stream. J. Fish Biol. 74: 1651–1661.</p><p>584Jonsson, B., Forseth, T., Jensen, A.J., and Naesje, T.F. 2001. Thermal performance of </p><p>585 juvenile Atlantic salmon, Salmo salar L. Funct. Ecol. 15: 701-711.</p><p>586Kalleberg, H. 1958. Observations in a stream tank of territoriality and competition in </p><p>587 juvenile salmon and trout (Salmo salar L. and S. trutta L. ). Inst. Freshw. Res. </p><p>588 Drottningholm Rep. 39: 55-98. </p><p>589Kaufman L., and Rousseeuw, P.J. 1990. Finding Groups in Data: An Introduction to </p><p>590 Cluster Analysis. John Wiley & Sons, Inc. Hoboken, NJ. USA.Keenleyside, </p><p>591 M.H.A. 1962. Skin diving observations of Atlantic salmon and brook trout</p><p>51 26 52 592 in the Miramichi River, New Brunswick. J. Fish. Res. Board Can. 19: 625-634.</p><p>593Kemp, P.S., Armstrong, J.D., and Gilvear, D.J. 2005. Behavioural responses of juvenile </p><p>594 Atlantic salmon (Salmo salar) to presence of boulders. River Res. Appl. 21: 1053-</p><p>595 1060.</p><p>596Kemp, P.S., Gilvear, D.J., and Armstrong, J.D. 2006. Variation in performance reveals </p><p>597 discharge-related energy costs for foraging Atlantic salmon (Salmo salar) parr. </p><p>598 Ecol. Freshw. Fish 15: 565-571.</p><p>599Liao, J.C. 2007. A review of fish swimming mechanics and behaviour in altered flows. </p><p>600 Philos. T. Roy. Soc. B. 362: 1973-1993.</p><p>601Linnansaari, T., Keskinen, A., Romakkaniemi, A., Erkinaro, J., and Orell, P. 2010. Deep </p><p>602 habitats are important for juvenile Atlantic salmon Ssalmo salar L. In large rivers.</p><p>603 Ecol. of Freshw. Fish 19: 618-626.</p><p>604Lucas, M.C., and Baras, E. 2000. Methods for studying spatial behaviour of freshwater </p><p>605 fishes in the natural environment. Fish Fish. 1: 283-316.</p><p>606Martel, G. 1996. Growth rate and influence of predation territoriality in juvenile coho </p><p>607 salmon (Oncorhynchus kisutch). Can. J. Fish. Aquat. Sci. 53: 660-669.</p><p>608McLaughlin, R.L., Grant, J.W.A., and Kramer, D.L. 1994. Foraging movements in </p><p>609 relation to morphology, water-column use, and diet for recently emerged brook </p><p>610 trout (Salvelinus fontinalis) in still-water pools. Can. J. Fish. Aquat. Sci 51: 268-</p><p>611 279.</p><p>612Morrissey, M.B., and Ferguson, M.M. 2011. Individual variation in movement </p><p>613 throughout the life cycle of a stream-dwelling salmonid fish. Mol. Ecol. 20: 235-</p><p>614 248.</p><p>53 27 54 615Nikora, V.I., Goring, D.G., and Biggs, B.J.F. 1998. On gravel-bed roughness </p><p>616 characterization. Water Resour. Res. 34: 517-527.</p><p>617Nislow, K.H., Folt, C., and Seandel, M. 1998. Food and foraging behavior in relation to </p><p>618 microhabitat use and survival of age-0 Atlantic salmon. Can. J. Fish. Aquat. Sci. </p><p>619 55: 116-127.</p><p>620Nykanen, M., Huusko, A., and Lahti, M. 2004. Changes in movement, range and habitat </p><p>621 preferences of adult grayling from late summer to early winter. J. Fish Biol. 64: </p><p>622 1386-1398.</p><p>623Okland, F., Thorstad, E.B., and Naesje, T.F. 2004. Is Atlantic salmon production limited </p><p>624 by number of territories? J. Fish Biol. 65: 1047-1055.</p><p>625Ovidio, M., Enders, E.C., Hallot, E.J., Roy, M.L., Philippart, J.C., Petit, F., and Roy, </p><p>626 A.G. 2007. Mobility and home-range use of Atlantic salmon parr over short time </p><p>627 scales. Aquat. Living Resour. 20: 95-101.</p><p>628Piccolo, J.J., Hughes, N.F., and Bryant, M.D. 2008. Water velocity influences prey </p><p>629 detection and capture by drift-feeding juvenile coho salmon (Oncorhynchus </p><p>630 kisutch) and steelhead (Oncorhynchus mykiss irideus). Can. J. Fish. Aquat. Sci. </p><p>631 65: 266-275.</p><p>632Power, M.E. 1984. Habitat quality and the distribution of algae-grazing catfish in a </p><p>633 Panamanian stream. J. Anim. Ecol. 53: 357-374. </p><p>634Riley, W.D., Eagle, M.O., Ives, M.J., Rycroft, P., and Wilkinson, A. 2003. A portable </p><p>635 passive integrated transponder multi-point decoder system for monitoring habitat </p><p>636 use and behaviour of freshwater fish in small streams. Fisheries Manag. Ecol. 10: </p><p>637 265-268.</p><p>55 28 56 638Riley, W.D., Ives, M.J., Pawson, M.G., and Maxwell, D.L. 2006. Seasonal variation in </p><p>639 habitat use by salmon, Salmo salar, trout, Salmo trutta and grayling, Thymallus </p><p>640 thymallus, in a chalk stream. Fisheries Manag. Ecol. 13: 221-236.</p><p>641Riley, W.D., Maxwell, D.L., Pawson, M.G., and Ives, M.J. 2009. The effects of low </p><p>642 summer flow on wild salmon (Salmo salar), trout (Salmo trutta) and grayling </p><p>643 (Thymallus thymallus) in a small stream. Freshwater Biol. 54: 2581-2599.</p><p>644Robertson, M.J., Pennell, C.J., Scruton, D.A., Robertson, G.J., and Brown, J.A. 2004. </p><p>645 Effect of increased flow on the behaviour of Atlantic salmon parr in winter. J. </p><p>646 Fish Biol. 65: 1070-1079.</p><p>647Rodriguez, M.A. 2002. Restricted movement in stream fish: The paradigm is incomplete, </p><p>648 not lost. Ecology 83: 1-13.</p><p>649Roghair, C.N. 2005. Brook trout movement during and after recolonization of a naturally </p><p>650 defaunated stream reach. N. Am. J. Fish. Manage. 25: 777-784.</p><p>651Ryan, P.M. 1986. Lake Use by Wild Anadromous Atlantic Salmon, Salmo salar, as an </p><p>652 Index of Subsequent Adult Abundance. Can. J. Aquat. Sci. 43: 2-11.</p><p>653Scruton, D.A., Ollerhead, L.M.N., Clarke, K.D., Pennell, C., Alfredsen, K., Harby, A., </p><p>654 and Kelly, D. 2003. The behavioural response of juvenile Atlantic salmon (Salmo </p><p>655 salar) and brook trout (Salvelinus fontinalis) to experimental hydropeaking on a </p><p>656 Newfoundland (Canada) river. River Res. Appl. 19: 577-587.</p><p>657Sigourney, D.B., Horton, G.E., Dubreuil, T.L., Varaday, A.M., and Letcher, B.H. 2005. </p><p>658 Electroshocking and PIT Tagging of Juvenile Atlantic Salmon: Are There </p><p>659 Interactive Effects on Growth and Survival? N. Am. J. Fish. Manage. 25: 1016-</p><p>660 1021.</p><p>57 29 58 661Steingrimsson, S.O., and Grant, J.W.A. 2003. Patterns and correlates of movement and </p><p>662 site fidelity in individually tagged young-of-the-year Atlantic salmon (Salmo </p><p>663 salar). Can. J. Fish. Aquat. Sci 60: 193-202.</p><p>664Steingrimsson, S.O., and Grant, J.W.A. 2008. Multiple central-place territories in wild </p><p>665 young-of-the-year Atlantic salmon Salmo salar. J. Anim. Ecol. 77: 448-457.</p><p>666Valdimarsson, S.K., Metcalfe, N.B., Thorpe, J.E., and Huntingford, F.A. 1997. Seasonal </p><p>667 changes in sheltering: effect of light and temperature on diel activity in juvenile </p><p>668 salmon. Anim. Behav. 54: 1405-1412.</p><p>669Venter, O., Grant, J.W.A., Noel, M.V., and Kim, J.-W. 2008. Mechanisms underlying the</p><p>670 increase in young-of-the-year Atlantic salmon (Salmo salar) density with habitat </p><p>671 complexity. Can. J. Fish. Aquat. Sci 65: 1956-1964.</p><p>672Wolman, M.G. 1954. A method of sampling coarse river-bed material. Trans. Am. </p><p>673 Geophys. Union. 35: 951-956.</p><p>674</p><p>675</p><p>59 30 60 676 677</p><p>678Table 1. Pearson correlation coefficients of mobility variables versus axis scores from an </p><p>679ordination of daily fish spatial behaviour in the study reach and proportion of total </p><p>680variance expressed by the two first ordination axes (n=681).</p><p>PCA1 PCA2 Distance travelled 0.56 - 0.52 Number of movements 0.51 - 0.14 Number of sites 0.52 0.04 Extent 0.38 0.83 Proportion of variance 0.70 0.20 681</p><p>682</p><p>683</p><p>684</p><p>685</p><p>686</p><p>687</p><p>688</p><p>689</p><p>690</p><p>691</p><p>692</p><p>693</p><p>694Table 2. Frequency of occurrence (n) and mean (range) of the four mobility variables for </p><p>695each behavioural type pooled for all individuals. </p><p>Stationary Sedentary Floater Wanderer N 161 213 134 111 Distance traveled (m) 2.2(0-12) 10.6(0.7-41) 70(7.6-2454) 115(15-2249) Number of movements 1.0(0-4) 3.4(1-11) 36(4-558) 10.9(3-154)</p><p>61 31 62 Number of sites 1.8(1-4) 3.3(2-6) 5.7(2-12) 5.8(3-17) Extent (m) 2.5(0-6) 7.23(1.2-35) 5.7(1.3-15) 28.2(16-43) 696</p><p>697</p><p>698</p><p>699</p><p>700</p><p>701</p><p>702</p><p>703</p><p>704</p><p>705</p><p>706</p><p>707</p><p>708</p><p>709</p><p>710Table 3. Geometric mean, total sum of squares, and within- and between-individual </p><p>711variation in four mobility variables and principle component 1 for 24 juvenile Atlantic </p><p>712salmon parr monitored for 6-97 days (619 observations). </p><p>713</p><p>714</p><p>Mean Total Intra Inter</p><p>(%) (%) PCA1 -0.1 1655.7 87 13 Distance travelled 0.93 167.7 88 12 Number of movements 0.68 92.5 87 13 Number of sites 0.69 18.9 85 15</p><p>63 32 64 Extent (m) 0.76 57.6 83 17 715</p><p>716</p><p>717</p><p>718</p><p>719</p><p>720</p><p>721</p><p>722</p><p>723</p><p>724</p><p>725</p><p>726</p><p>727</p><p>728Figure captions</p><p>729Fig. 1. Water temperature (upper curve) and stage (lower curve) recorded from 24 July to </p><p>73030 October 2008. The vertical dashed line divides the study into summer and autumn </p><p>731periods based on a threshold of 12oC. The horizontal dashed line shows the flow stage </p><p>732matching bankfull discharge. *indicates the two fish tagging sessions. </p><p>733</p><p>734Fig. 2. Principal component analysis (PCA) on 50 Atlantic salmon parr daily mobility </p><p>735variables (N=681) during 97 days. Each dot represents the mobility of an individual on a </p><p>736particular day. Open circles show individual average values for the 24 fish that remained </p><p>737in the reach for six days or more. Polygons delineate behavioural types (stationary, </p><p>738sedentary, floater and wanderer) discriminated by a cluster analysis (K-means) on the </p><p>65 33 66 739daily mobility data: Number of sites, Distance travelled (m), Number of movements and </p><p>740Extent (m).</p><p>741</p><p>742Fig.3. Typical daily mobility corresponding to four behavioural types. Examples were </p><p>743selected based on the closest average PCA1 and PCA2 scores for each type: 1- (star) </p><p>744Stationary: 0 movement (18 Aug., Fish 15); 2- (black) Sedentary: 3 movements, 4 sites </p><p>745(22 Sept., Fish 50), 3- (white) Floater: 37 movements, 5 sites (24 Aug., Fish 37), 4- </p><p>746(dashed) Wanderer 14 movements, 11 sites (22 Sept, Fish 66). Contour shows depth at an</p><p>747estimated discharge of 0.4 m3/s (flow stage: 15 cm).</p><p>748</p><p>749</p><p>750</p><p>751Fig. 4. a) Number of days Atlantic salmon parr stayed in the reach subdivided by </p><p>752behavioural type. Dashed line indicates the fish that stayed more than 6 days. b) </p><p>753Proportion of days fish showed each of the behavioural types. Most individuals exhibited </p><p>754all types of behaviour during the study period.</p><p>755</p><p>756Fig. 5. Time series of the number of individuals tracked on the study site decomposed by </p><p>757behavioural types.42 individuals were PIT tagged on 24 July and 24 more were added on </p><p>75828 Aug. 2008. </p><p>759</p><p>760Fig.6. Proportion of fish behaviour exhibited on a daily basis by all individuals in relation</p><p>761to flow stage: low (0-10 cm, n= 34days), median (10-15, n=34 days), high (15-20 cm, </p><p>762n=13 days), very high (>20 cm, n=16 days).</p><p>67 34 68 763</p><p>764Fig.7. a) Daily averaged flow depth used per behaviour types pooled for all fish (unequal </p><p>765number of days per fish). b) Daily averaged flow depth used, each line representing </p><p>766averages per behaviour types per individual. Five individuals (bold lines) exhibited a </p><p>767relatively higher depth used while adopting floater behaviour. </p><p>768</p><p>769 770 771 772 773 774 775 776 777 778 779 780Figures 1 to 7</p><p>781</p><p>69 35 70 782</p><p>783</p><p>784</p><p>71 36 72 785</p><p>786</p><p>73 37 74 787 788</p><p>75 38 76</p>
Details
-
File Typepdf
-
Upload Time-
-
Content LanguagesEnglish
-
Upload UserAnonymous/Not logged-in
-
File Pages38 Page
-
File Size-