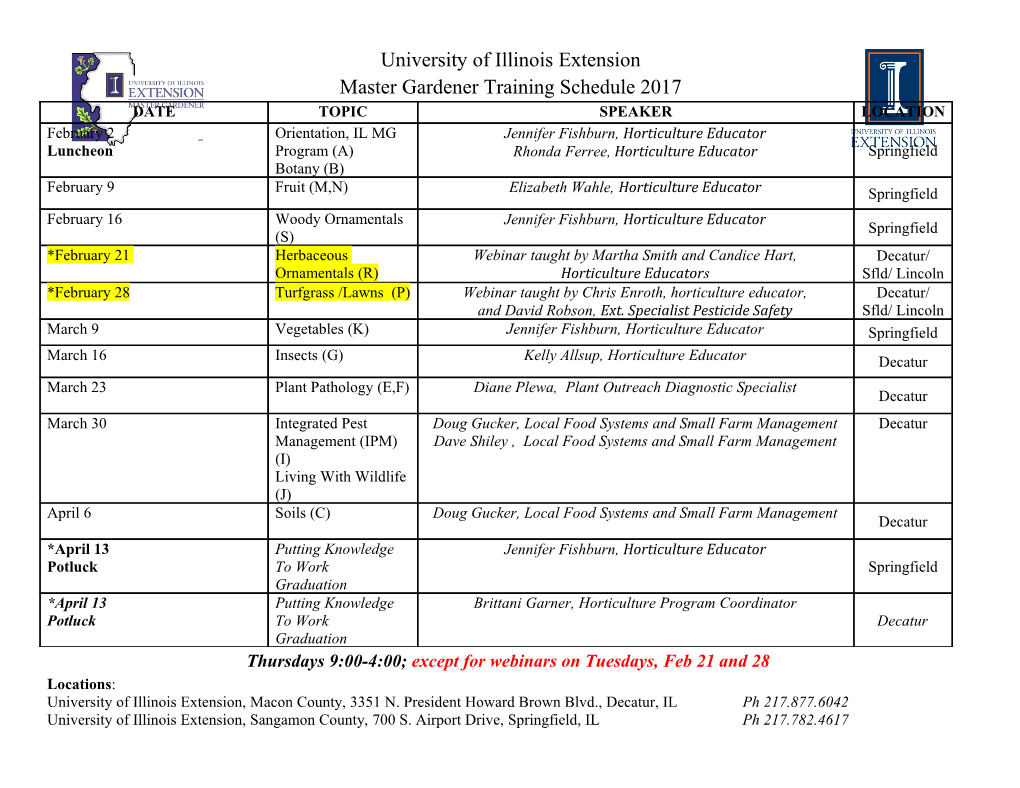
<p> 1</p><p>Rangeland Inventory, Monitoring, and Evaluation</p><p>Susan Muir (New Mexico State University) Mitchel P. McClaran (University of Arizona)</p><p>16 September 1997 (Draft for Review)</p><p>This is not an index to every page in this section, but most of the higher-level ones. It is designed to give an overview of the subject matter the chapter covers and access to most parts.</p><p>Introduction</p><p>This is the top-level page of the chapter, with an overview of rangeland inventory and monitoring and links to the 5 main sections below.</p><p>General Principles of Rangeland Inventory and Monitoring</p><p> Site Selection o Range Sites o Key Areas o Comparison Areas o Critical Areas Selection of Attributes o Key Species o Species Groups Time of Sampling How Often to Sample </p><p>Sampling Concepts</p><p> Sampling Terminology and Theory Sample Units o Sample Unit Size o Sample Unit Shape o Sample Size Allocation of Sample Units - Sampling Designs o Random Sampling o Systematic Sampling o Selected Sampling o Stratified Sampling </p><p>Vegetation Attributes 2</p><p> Biomass o Biomass Property o Dry Matter Content o Sampling Date o Separation of Dead and Live Material o Methods to Determine Biomass . Direct Methods to Determine Biomass . Harvesting to Determine Biomass . Estimation Approaches to Determine Biomass . Sample Units for Direct Methods to Determine Biomass . Indirect Methods to Determine Biomass . Climatic Records . Plant Dimensions . Reference Unit Method o Statistical Analysis of Biomass Data Cover o Methods to Determine Cover . Point Sampling to Determine Cover . Line Sampling to Determine Cover . Sampling in Quadrats to Determine Cover . Plotless Sampling to Determine Cover o Statistical Analysis of Cover Data Density o Boundary Decisions o Identification of Individuals o Influence of Spatial Patterns o Rooted or Canopy Dimensions o Species Groups o Methods to Determine Density . Counting Individual Plants . Sample Units for Counting Individual Plants to Determine Density . Data Recording and Calculations when Counting Individual Plants to Determine Density . Distance Methods o Statistical Analysis of Density Data Frequency o Nested Quadrats o Rooted or Canopy Dimensions o Species Groups o Specific Guidelines on Sample Unit Size to Determine Frequency o Sources of Sampling Error o Methods to Determine Frequency . Data Recording and Calculations to Determine Frequency o Statistical Analysis of Frequency Data Species Composition 3</p><p> o Species Groups o Dry Weight Rank Method o Species Data from Other Attributes </p><p>Rangeland Evaluation</p><p> Range Sites Range Condition Range Trend Recent Proposals for Evaluating the Status of Rangeland Resources o Desired Plant Community o Rangeland Health o Resource Value Rating o Site Conservation Rating </p><p>Management Applications</p><p> Carrying Capacity o Methods to Determine Carrying Capacity . Matching Forage Supply and Animal Demand . Range Sites Utilization o Methods to Determine Utilization . Grazed and Ungrazed Comparisons . Grazed Class Method . Grazed Plant Method . Height-Weight Method . Utilization Zones </p><p>Introduction | General Principles | Sampling Concepts | Vegetation Attributes Rangeland Evaluation | Management Applications | Chapter Outline</p><p>Introduction</p><p>Rangeland inventory and rangeland monitoring are the processes of describing and evaluating the resources at a rangeland site. a. Rangeland inventory is information collected to document and describe the existing resource status within a management unit. Features included depend on the purpose of the inventory, but in rangeland situations are likely to entail vegetation types, range sites, range condition, carrying capacity, soil types, utilization patterns, topography, streams, habitat assessments for wildlife, and improvements such as roads, watering points, and fences. 4 b. Rangeland monitoring is conducted to record changes in resource status, usually to assess the response to a management program at a site. Such changes can only be detected by a series of measurements spanning time. Data collected from a range inventory provides a valuable baseline against which to compare responses, but monitoring can rarely be conducted at the same level of detail as the information provided by an inventory. Instead, monitoring is usually based on observations of key areas and key vegetation attributes carefully selected to meet the objectives of the program. For example, species composition could be measured in a riparian area to determine the impact of a certain grazing system, changes in mesquite (Prosopis spp.) density could be used to assess the effectiveness of herbicide control, or ground cover could be chosen to monitor the impact of tourism at a popular site in a National Park.</p><p>Inventory and monitoring have always been central themes of rangeland management, as tools to assist in making wise decisions according to the productive potential of the land resource, such as, carrying capacity, utilization levels, grazing systems, and range improvements. Today under the philosophy of multiple use and increased legislative demands, however, inventory and monitoring programs serve a greater variety of interests and groups. For example, Artz (1984, p. 600) enumerated the following objectives of inventory and monitoring programs.</p><p>1. To develop high quality land use plans. 2. To allocate resources to uses and users. 3. To assess current conditions and to monitor conditions in the future for measurement of progress towards goals. 4. To assess impacts of proposed land use actions. 5. To assess capability or potential of resource production under various levels of management. 6. To establish a common basis of measurement between various land types and ownerships. 7. To assist in defending decisions in hearings and court actions. 8. To satisfy legal requirements. </p><p>These objectives must be implemented at different time scales and levels of detail, depending on the purpose and end-user of the resource information. For example, local land managers (e.g., ranchers, stewards of local natural public reserves), need detailed site information to plan and evaluate specific projects, such as erosion control, reseeding programs, the management of invasive plants, or the regulation of wildlife populations. Conversely, regional and national administrators are increasingly obliged by legal mandates to use inventory and monitoring information for a variety of assignments, from district land use plans to large-scale national inventories reporting on current resource status, which rely on data collected at more general or summarized levels.</p><p>When planning an inventory or monitoring program, the objectives must be balanced against the availability of resources, including time, money, and skilled personnel. In combination, these criteria will influence inventory and monitoring strategies, sampling 5 designs, which vegetation attributes are collected, and evaluation of the final data from ecological and management perspectives.</p><p>In this chapter, you will find the following sections:</p><p> General Principles of Inventory and Monitoring Sampling Concepts Vegetation Attributes Rangeland Evaluation Management Applications </p><p>References and Further Reading</p><p>Artz, J.L. 1984. The environment of rangeland inventory 1980. In: National Research Council/National Academy of Sciences. Developing strategies for rangeland management. Westview Press. pp 593-605.</p><p>Bonham, C.D. 1989. Measurements for terrestrial vegetation. John Wiley & Sons, New York, NY. p 265.</p><p>Holechek, J.L., Pieper, R.D., and C.H. Herbel. 1995. Range management principles and practices. Prentice Hall, Englewood Cliffs, NJ. 2nd ed. pp 159-176.</p><p>National Research Council. 1994. Rangeland health: New methods to classify, inventory and monitor rangelands. National Academy Press. pp 134-157.</p><p>Pearson, H.A., and J.W. Thomas. 1984. Adequacy of inventory data for management interpretations. In: National Research Council/National Academy of Sciences. Developing strategies for rangeland management. Westview Press. pp 745-763.</p><p>Smith, E.L. 1984. Use of inventory and monitoring data for range management purposes. In: National Research Council/National Academy of Sciences. Developing strategies for rangeland management. Westview Press. pp 809-842.</p><p>West, N.E., McDaniel, K., Smith, E.L., and S. Leonard. 1994. Monitoring and interpreting ecological integrity on arid and semi-arid lands of the western United States. Range Improvement Task Force, Las Cruces, NM. pp 1-15.</p><p>Rangeland Inventory, Monitoring, and Evaluation: General Principles </p><p>Introduction | General Principles | Sampling Concepts | Vegetation Attributes Rangeland Evaluation | Management Applications | Chapter Outline</p><p>Reliable inventory and monitoring data are difficult to obtain in rangeland situations, which are characterized by extensive areas and considerable spatial and temporal in 6 attributes. Although inventory and monitoring embrace many similar principles, different vegetation attributes and sampling methods are often required to meet the specific objectives of each process.</p><p>For example, inventories usually aim to provide a detailed overview of resources for the entire management unit. In this situation, sampling strategies should be chosen to give an accurate depiction of important attributes for the whole site, best obtained from observations at many locations. In contrast, monitoring is conducted over a duration of years, and usually limited to detecting range trends in selected areas and for key species. Furthermore, different personnel are often involved in collecting the repeated measurements during monitoring, meaning sampling strategies should be easy to follow and promote precise results that are free from observer variability.</p><p>Therefore, no single recipe can be prescribed as the design that will most efficiently measure rangeland attributes. Instead, sampling strategies must be tailored according to specific inventory or monitoring objectives, that govern the purposes of the data once it is collected. However, objectives must be devised to suit the type of vegetation being sampled and to accommodate the limits of time or labor skills. In many cases, further compromises must be reached between optimizing the accuracy and precision of sampling strategies with budgetary constraints.</p><p> Site Selection Selection of Attributes Time of Sampling How Often to Sample </p><p>References and Further Reading</p><p>Bureau of Land Management. 1996. Sampling vegetation attributes. Interagency Technical Reference, BLM/RS/ST-96/002+1730. pp 1-29.</p><p>McClaran, M.P., and D.N. Cole. 1993. Packstock in wilderness: Use, impacts, monitoring, and management. General Technical Report INT-301. Intermountain Research Station, United States Department of Agriculture, Forest Service. Ogden, UT. pp 10-16.</p><p>Pieper, R.D. 1984. A critique of "Methods for Inventory and Monitoring of Vegetation, Litter, and Soil Surface Condition". In: National Research Council/National Academy of Sciences. Developing strategies for rangeland management. Westview Press. pp 691- 701.</p><p>Risser, P.G. 1984. Methods for inventory and monitoring of vegetation, litter, and soil surface condition. In: National Research Council/National Academy of Sciences. Developing strategies for rangeland management. Westview Press. pp 647-690. 7</p><p>Nevada Range Studies Task Group. 1984. Nevada rangeland monitoring handbook. Soil Conservation Service, Forest Service, Bureau of Land Management, University of Nevada Reno, Agricultural Research Service and Range Consultants. September 1984. pp 1-49.</p><p>Smith, E.L., and G.B. Ruyle. 1991. Considerations when monitoring rangeland vegetation. G.B. Ruyle. (ed). Some Methods for Monitoring Rangelands and Other Natural Area Vegetation. University of Arizona, College of Agriculture, Extension Report 9043. pp 1-5.</p><p>Site Selection</p><p>Selection of sites for sampling is a critical consideration when designing rangeland inventory or monitoring schedules. Documentation of site locations, either on maps or with clear written directions, is also an important component of the sampling protocol.</p><p>Site selection typically assumes a procedure of selected sampling for either inventory or monitoring programs. After general locations have been identified, further decisions must be made concerning the layout of sample units within the selected sampling area. This next step entails the adoption of a combination of random sampling and systematic sampling approaches.</p><p>When undertaking an inventory of an area, it is preferable to establish sampling locations throughout the management unit to ensure a thorough coverage of the site. Sampling efficiency may be obtained by adopting a stratified sampling scheme, often using range sites as the basis of stratification.</p><p>In monitoring, it is usually only practical to sample a few areas. Each site should be restricted to relatively uniform areas, such as range sites, to facilitate data collection and guide the degree to which interpretations can be extrapolated beyond the immediate sampling area. Permanently located sites reduce sampling error associated with spatial variation of the attributes so that data can provide a clearer depiction of range trend over time.</p><p>The location of sites and sample size depend upon the selection of attributes and their variability within the management unit, the type of management and expected responses, the availability of sampling resources, and the purpose of the inventory or monitoring program. Effective site selection will minimize sampling requirements, enhance statistical accuracy and precision, and ensure that the collected data can be interpreted in a manner relevant to the objectives of the program. Therefore, several different approaches are commonly used to select and classify sampling sites, reflecting the various purposes of inventory or monitoring programs.</p><p> Range Sites Key Areas Comparison Areas 8</p><p> Critical Areas </p><p>References and Further Reading</p><p>(Note: pdf files require Adobe Acrobat (free) to view)</p><p>Bureau of Land Management. 1996. Sampling vegetation attributes. Interagency Technical Reference, BLM/RS/ST-96/002+1730. pp 3-13.</p><p>Larson, L.L., and P.A. Larson. 1987. Use of microsite sampling to reduce inventory sample size. Journal of Range Management 40:378-379. (pdf)</p><p>McClaran, M.P., and D.N. Cole. 1993. Packstock in wilderness: Use, impacts, monitoring, and management. General Technical Report INT-301. Intermountain Research Station, United States Department of Agriculture, Forest Service. Ogden, UT. pp 13-14.</p><p>Reppert, J.N., Reed, M.J., and P. Zusman. 1962. An allocation plan for range unit sampling. Journal of Range Management 15:190-193. (pdf)</p><p>Smith, E.L., and G.B. Ruyle. 1991. Considerations when monitoring rangeland vegetation. G.B. Ruyle. (ed). Some methods for monitoring rangelands and other natural area vegetation. University of Arizona, College of Agriculture, Extension Report 9043. pp 2-4.</p><p>Range Sites</p><p>Range sites are the principal units of rangeland classification that are based on categorizing vegetation according to site potential. Site potential is defined as the capacity of an area to support a distinct species composition and/or total biomass. Range sites act as the primary organizational element to obtain inventory and monitoring information during sampling. Therefore, the vegetation represented by a range site must be sufficiently uniform for the valid interpretation and extrapolation of data for management applications, yet incorporate the inherent variability expected in rangeland landscapes.</p><p>The range site concept was developed by the Soil Conservation Service (SCS, now Natural Resources Conservation Service) by adopting ideas previously introduced into forestry science. Other government agencies with land management responsibilities (ie., Bureau of Land Management, and Forest Service) use similar, but not always equivalent, concepts and terms to classify rangelands, such as ecological site, ecological type, or habitat type.</p><p>Most important, range site classification is centered on identifying differences in potential productivity, rather than simply categorizing the existing vegetation. A classification based on site potential is more meaningful to planning and management because it 9 encourages the consideration of a wider range of options, rather than being restricted to existing conditions. However, deciding the nature of the potential plant community is also subject to the biases of personal judgement, particularly when the vegetation has been modified under a long tradition of land use.</p><p>These differences among range sites reflect the combined influence of environmental factors, including climate (total precipitation, seasonality of precipitation, temperature, frost free days, etc.), soils (soil surface texture, water holding capacity, etc.), topography, and fire. Therefore, common principles of ecological succession are incorporated into the range site concept, by assuming that only one distinctive plant community (the climax community), can evolve under a certain set of environmental conditions.</p><p>The importance of soil characteristics and topography on plant growth is well recognized, and these factors are usually included when naming range sites (e.g. Limestone Hills, Sandy Loam Upland, Clayey Bottom, etc.). Soil maps are often adopted as the foundation of range site maps, but extensive field reconnaissance, coupled with sampling of vegetation attributes such as biomass and species composition, must also be followed during the process of delineating range sites within an area. The same range site can occur at different locations across an extensive region, wherever the vegetation is considered to exhibit a similar potential for productivity and species composition.</p><p>Comprehensive descriptions have been developed for each range site, based on research, field surveys and professional observation. The description includes climate, topography, soils, potential vegetation, and management interpretations such as carrying capacity and resource value ratings. A major disparity between the various government agencies is that descriptions of the potential plant community are restricted to native species under Natural Resource Conservation Service (NRCS) procedures, whereas other agencies include naturalized introduced plants. This difference has considerable consequences, particularly when using the descriptions as benchmarks of site potential for range condition assessments.</p><p> USDA/NRCS Ecological Site Descriptions </p><p>References and Further Reading</p><p>(Note: pdf files require Adobe Acrobat (free) to view)</p><p>Anderson, E.W. 1983. Ecological site / range site / habitat type: A viewpoint. Rangelands 5:187-188. de Oliveira, J.G.B. 1979. Characterization of range sites. Ph.D. Dissertation, University of Arizona. pp. 1-105.</p><p>Driscoll, R.S. 1984. Classification for stratification, mapping, and data display for range inventories. In: National Research Council/National Academy of Sciences. Developing strategies for rangeland management. Westview Press. pp. 615-626. 10</p><p>Frost, W.E., and E.L. Smith. 1991. Biomass productivity and range condition on range sites in southern Arizona. Journal of Range Management 44:64-66. (pdf)</p><p>Leonard, S.G., Staidl, G.J., Gebhardt, K.A., and D.E. Prichard. 1992. Viewpoint: Range site/ecological site information requirements for classification of riverine riparian ecosystems. Journal of Range Management 45:431-435. (pdf)</p><p>Mueggler, W.F. 1984. Classification for stratification, mapping, and data display for range inventory: Comments and discussion. In: National Research Council/National Academy of Sciences. Developing strategies for rangeland management. Westview Press. pp. 627-630.</p><p>National Research Council. 1994. Rangeland health: New methods to classify, inventory and monitor rangelands. National Academy Press. pp. 66-75, 82-85.</p><p>Shiftlet, T.N. 1973. Range sites and soils in the United States. In: D.N. Hyder. (ed.) Arid shrublands. Proceedings of the Third Workshop of the United States/Australia Rangelands Panel. Society for Range Management, Denver, CO. pp. 26-33.</p><p>Society for Range Management. 1983. Guidelines and terminology for range inventories and monitoring. Report of the Range Inventory Standardization Committee. Society for Range Management, Denver, CO.</p><p>Soil Conservation Service. 1976. National range handbook. United States Department of Agriculture.</p><p>Task Group on Unity in Concepts and Terminology. 1995. New concepts for assessment of range condition. Journal of Range Management 48:271-282.</p><p>Weixelman, D., Zamudio, D., Zamudio, K., and R. Tausch. 1997. Classifying ecological types and evaluating site degradation. Journal of Range Management 50:315-321.</p><p>Key Areas</p><p>Key areas are sampling sites deliberately selected, based mainly on professional judgement, in rangeland inventory or monitoring programs to be representative of the entire management unit. Data from key areas are interpreted as an indicative guide of average responses to land use throughout the management unit.</p><p>As a type of selected sampling, the capacity of a key area to represent the entire area depends on the experience and skill of observers selecting the location. In general, key areas should represent common range sites of the management unit, and address the objectives of the monitoring program. For example, when monitoring utilization, key areas should provide important sources of forage, but not be too close nor too distant from water points since neither of those locations will be a typical indication of grazing patterns over the entire area. 11</p><p>References and Further Reading</p><p>Bureau of Land Management. 1996. Sampling vegetation attributes. Interagency Technical Reference, BLM/RS/ST-96/002+1730. pp 2-3.</p><p>Holechek, J.L., Pieper, R.D., and C.H. Herbel. 1995. Range management principles and practices. Prentice Hall, Englewood Cliffs, NJ. 2nd ed. pp 204-205.</p><p>Nevada Range Studies Task Group. 1984. Nevada rangeland monitoring handbook. Soil Conservation Service, Forest Service, Bureau of Land Management, University of Nevada Reno, Agricultural Research Service and Range Consultants. September 1984. pp 2-3, 9-10.</p><p>Smith, E.L., and G.B. Ruyle. 1991. Considerations when monitoring rangeland vegetation. G.B. Ruyle. (ed). Some methods for monitoring rangelands and other natural area vegetation. University of Arizona, College of Agriculture, Extension Report 9043. pp 2-3.</p><p>Comparison Areas</p><p>Comparison areas, also known as reference areas or benchmark areas, are additional sampling locations established in rangeland inventory or monitoring programs to isolate the effects of land use from other natural events (especially weather factors) influencing vegetation responses. Therefore, comparison areas are usually chosen at localities with minimal management impacts, such as livestock exclosures or sites distant from water points. In other situations, fenceline contrasts reveal useful cues to separate the effects of land use from weather conditions.</p><p>Credible interpretations of land use impacts depend upon the selection of comparison areas that are representative of corresponding key areas or critical areas, partially realized by restricting sampling locations to the same range site. Problems of variability within a range site could be overcome by including multiple comparison areas, although this is seldom practical within the scope of time and budgetary constraints associated with most land evaluation programs.</p><p>References and Further Reading</p><p>Bureau of Land Management. 1996. Sampling vegetation attributes. Interagency Technical Reference, BLM/RS/ST-96/002+1730. p 6.</p><p>Smith, E.L., and G.B. Ruyle. 1991. Considerations when monitoring rangeland vegetation. G.B. Ruyle. (ed). Some methods for monitoring rangelands and other natural area vegetation. University of Arizona, College of Agriculture, Extension Report 9043. pp 2-3. 12</p><p>Wilson, A.D. 1984. Points of reference in the assessment of change in vegetation and land condition. Australian Rangeland Journal 6:69-74.</p><p>Critical Areas</p><p>Critical areas are sampling locations deliberately chosen in rangeland inventory or monitoring programs because of unique values or special concerns such as riparian zones, restricted habitats for threatened or endangered species, or range sites highly susceptible to erosion.</p><p>Unlike key areas, vegetation responses observed in critical areas are not interpreted to reflect management impacts over the entire management unit. Instead, critical areas are evaluated separately to ensure the fulfillment of specific goals that are associated with resource conservation.</p><p>References and Further Reading</p><p>Bureau of Land Management. 1996. Sampling vegetation attributes. Interagency Technical Reference, BLM/RS/ST-96/002+1730. pp 2-3.</p><p>Smith, E.L., and G.B. Ruyle. 1991. Considerations When Monitoring Rangeland Vegetation. G.B. Ruyle. (ed). Some methods for monitoring rangelands and other natural area vegetation. University of Arizona, College of Agriculture, Extension Report 9043. pp 2-3.</p><p>Selection of Attributes</p><p>Rangeland inventory and monitoring programs have usually focused on describing vegetation attributes, although soil characteristics are assuming greater importance in recent proposals for evaluating the status of rangeland resources. Vegetation attributes are generally regarded as easier to quantify compared to soil properties, which often rely on subjective assessments. Information on weather conditions, stocking rates, utilization patterns, wildlife, wildfires, and multiple use activities are useful to supplement measured vegetation responses, and to aid in interpreting the results. Photographs also provide a valuable visual record of the overall status of rangeland resources.</p><p>No single vegetation attribute is best suited to describe the existing status or to detect trends in rangeland vegetation. Instead, attributes should be selected in light of the objectives of each inventory or monitoring program, the type of vegetation, and the availability of skilled observers. Ideally, attributes should involve rapid and simple sampling methods, to ensure that accurate and precise data can be collected within the budgetary and time constraints associated with many rangeland evaluation plans.</p><p>Vegetation attributes commonly measured in rangeland inventory and monitoring programs include: 13</p><p> Biomass - is the primary objective of inventories conducted to determine carrying capacity, utilization patterns, or range condition. Despite its importance in management applications, few techniques are available to rapidly obtain accurate and precise biomass data for large areas, so that other attributes such as cover are usually recommended for large scale monitoring programs. It is also difficult to isolate fluctuations in biomass created by weather conditions and immediate grazing history from changes induced by active management. Cover - is widely adopted for both inventory and monitoring purposes because it is an attribute that provides rapid and repeatable data that is relevant to many practical interpretations regarding land use (eg., forage availability, wildlife habitat) and resource status (eg., erosion potential). Basal cover is generally considered a more stable measure than canopy cover to identify herbaceous vegetation change, since it is less influenced by weather fluctuations, time of year, and immediate grazing history. Density - may provide useful inventory information for large perennial species, particularly trees and shrubs. However, density is rarely measured in monitoring situations, because it can be very time consuming, and there are errors associated with the identification of individual plants. Frequency - is regularly used in monitoring programs, because it is a rapid technique that provides precise results. Although a sensitive index of changes in a species over time, additional data must be collected to reveal which other attribute caused the change. Frequency has less relevance in inventory applications, because it does not provide an absolute measure of species abundance. Species Composition - is commonly assessed to describe the character of vegetation during detailed inventory programs. However, accurate determination of species composition can be tedious, because all species must be sampled. Therefore, in long-term or broadscale monitoring situations, it can be more efficient to concentrate on a few key species or species groups, chosen to meet the objectives of the program. </p><p>In addition to deciding which vegetation attributes to measure, the planning of inventory or monitoring programs must also select which plant species to include in the sampling protocol.</p><p> Key Species Species Groups </p><p>References and Further Reading</p><p>Bonham, C.D. 1989. Measurements for terrestrial vegetation. John Wiley & Sons, New York, NY. pp 268-283.</p><p>Bureau of Land Management. 1996. Sampling vegetation attributes. Interagency Technical Reference, BLM/RS/ST-96/002+1730. pp 23-29. 14</p><p>Holechek, J.L., Pieper, R.D., and C.H. Herbel. 1995. Range management principles and practices. Prentice Hall, Englewood Cliffs, NJ. 2nd ed. pp 162-165.</p><p>McClaran, M.P., and D.N. Cole. 1993. Packstock in wilderness: Use, impacts, monitoring, and management. General Technical Report INT-301. Intermountain Research Station, United States Department of Agriculture, Forest Service. Ogden, UT. pp 11-13.</p><p>Pieper, R.D. 1984. A critique of "Methods for Inventory and Monitoring of Vegetation, Litter, and Soil Surface Condition". In: National Research Council/National Academy of Sciences. Developing strategies for rangeland management. Westview Press. pp 691- 701.</p><p>Risser, P.G. 1984. Methods for inventory and monitoring of vegetation, litter, and soil surface condition. In: National Research Council/National Academy of Sciences. Developing strategies for rangeland management. Westview Press. pp 647-690.</p><p>Smith, E.L., and G.B. Ruyle. 1991. Considerations when monitoring rangeland vegetation. G.B. Ruyle. (ed). Some methods for monitoring rangelands and other natural area vegetation. University of Arizona, College of Agriculture, Extension Report 9043. pp 1-2.</p><p>Key Species</p><p>Rangeland inventory or monitoring programs usually concentrate on sampling only a few important species or species groups that serve as indicators of status and/or trend for the entire vegetation. Key species should be selected to satisfy program objectives, such as rare species or important forage plants. This approach permits more efficient sampling and interpretation, because measuring all species is time consuming and often provides greater detail than required.</p><p>Because most inventory and monitoring programs have typically evaluated the impact of livestock grazing on rangelands, important forage plants have traditionally been selected as key species. Consequently, when the key species concept is commonly applied to determine utilization levels, selection of plants could vary according to dietary preferences associated with the type of animal using the rangeland and time of sampling. In this situation, the utilization of key species is extrapolated to judge the proper use of other species at the site. Therefore, key species should generally be abundant, productive and palatable components of the vegetation, and moderately sensitive to grazing.</p><p>However, key species can be selected to meet any management objective. For example, key species may be chosen in relation to their role in watershed protection, or as threatened or endangered species requiring special attention. Furthermore, if the management goal is to improve low range condition, key species may not be abundant in the existing vegetation, but should still possess a seed source and be known as an indicator of improving range condition. 15</p><p>References and Further Reading</p><p>Bureau of Land Management. 1996. Sampling vegetation attributes. Interagency Technical Reference, BLM/RS/ST-96/002+1730. pp 4-5.</p><p>Holechek, J.L., Pieper, R.D., and C.H. Herbel. 1995. Range management principles and practices. Prentice Hall, Englewood Cliffs, NJ. 2nd ed. pp 204-205.</p><p>Nevada Range Studies Task Group. 1984. Nevada rangeland monitoring handbook. Soil Conservation Service, Forest Service, Bureau of Land Management, University of Nevada Reno, Agricultural Research Service and Range Consultants. September 1984. pp 2-3, 10.</p><p>Roberts, B.R., and D.P.J. Opperman. 1974. Veld management recommendations, a reassessment of key species and proper use factors. Proceedings of the Grassland Society of Southern Africa 9:149-155.</p><p>Smith, A.D. 1965. Determining common use grazing capacities by application of the key species concept. Journal of Range Management 18:196-201.</p><p>Species Groups</p><p>Considering each individual species at the site, even to determine species composition, is often a time consuming and inefficient approach to collect data, especially for attributes determined by counting, such as frequency or density. Accuracy can be compromised from species misidentification (particularly for seedlings, grazed, or rare plants), and such detailed information may be unnecessary to satisfy many inventory or monitoring objectives. Many statistical inference procedures are also ineffective when a species is rarely recorded.</p><p>Instead, many studies focus on close inspection of a few key species that are nominated to meet the goals of the sampling program. Remaining species of interest can be allocated to broad groups that are based on either morphological characteristics, eg., Aristida sp., or functional features, eg., perennial forage grasses or succulents. Clear ground rules are needed to ensure that species are consistently designated to their correct group.</p><p>References and Further Reading</p><p>(Note: pdf files require Adobe Acrobat (free) to view)</p><p>Bartos, D.L., Brown, J.L., and G.D. Booth. 1994. Twelve years of biomass response in aspen communities following fire. Journal of Range Management 47:79-83. (pdf)</p><p>Mueller-Dombois, D., and H. Ellenburg. 1974. Aims and methods of vegetation ecology. John Wiley & Sons, New York. pp. 140-145. 16</p><p>Haferkamp, M.R., Volesky, J.D., Borman, M.M., and Heitschmidt, R.K., and P.O. Currie. 1993. Effects of mechanical treatments and climatic factors on the productivity of Northern Great Plains rangelands. Journal of Range Management 46:346-350. (pdf)</p><p>Hartnett, D.C., Hickman, K.R., and L.E. Walter. 1996. Effects of bison grazing, fire, and topography on floristic diversity in tallgrass prairie. Journal of Range Management 49:413-420.</p><p>Jameson, D.A. 1991. Effects of a single season and rotation harvesting on cool- and warm-season grasses of a mountain grassland. Journal of Range Management 44:327- 329. (pdf)</p><p>Time of Sampling</p><p>Season to season fluctuations in vegetation attributes represent the key consideration when deciding what time of year to conduct rangeland inventory or monitoring programs. To prevent seasonal differences from confounding interpretations, comparisons between inventory data from various sites or monitoring data from a single site over a number of years, should be confined to the same season so that key species are at a similar level of maturity. Also, it is possible to select vegetation attributes that are relatively insensitive to seasonal fluctuations, such as basal cover, to minimize the effects of sampling during different seasons.</p><p>The best time of the year to sample depends upon the objectives of the program and the selection of attributes to be measured. For example, regular monitoring to evaluate stocking rates by determining utilization levels are best conducted at the end of the grazing season. If species composition is identified as a monitored attribute, more accurate data collection is possible by coinciding field work with the period when plants are flowering and identifiable.</p><p>Other secondary factors may also control the most practical time of sampling. For example, sampling opportunities may be restricted by boggy soils limiting the accessibility to high elevation sites during spring, the availability of skilled labor, or conflicting workplace commitments.</p><p>References and Further Reading</p><p>Bureau of Land Management. 1996. Sampling vegetation attributes. Interagency Technical Reference, BLM/RS/ST-96/002+1730. pp 1-29.</p><p>McClaran, M.P., and D.N. Cole. 1993. Packstock in wilderness: Use, impacts, monitoring, and management. General Technical Report INT-301. Intermountain Research Station, United States Department of Agriculture, Forest Service. Ogden, UT. p 13. 17</p><p>Smith, E.L., and G.B. Ruyle. 1991. Considerations when monitoring rangeland vegetation. G.B. Ruyle. (ed). Some methods for monitoring rangelands and other natural area vegetation. University of Arizona, College of Agriculture, Extension Report 9043. p 3.</p><p>How Often to Sample</p><p>Data need only be collected on a single occasion for an inventory program, to document rangeland resources and to evaluate their current status. In contrast, monitoring must be conducted on a recurrent basis to detect changes in vegetation attributes. Although it may appear simpler to identify and interpret the causes of vegetation change under an annual sampling regime, such a protocol is rarely undertaken except when monitoring utilization. For example, vegetation changes large enough to assume practical significance often take more than one year to become evident, particularly after allowing for variability arising from weather, and sampling error factors. Consequently, the optimum interval between sampling depends on the longevity of key species. For example, long-lived perennial shrubs such as mesquite (Prosopis spp.) need not be monitored as frequently as populations of shorter-lived annuals or biennials such as Rothrock grama (Bouteloua rothrockii). In addition, frequent sampling is usually limited by time and budgetary constraints.</p><p>Therefore, with the exception of biomass data to evaluate utilization patterns or residual biomass levels, it is not unusual to return to sample sites only once every 3 to 5 years in a long-term monitoring program. More frequent monitoring may be desirable for newly developed schedules, to confirm that the selection of attributes, time of sampling, sampling methods and site selection are suited to the objectives of the program. In other situations, it may be plausible to a) measure a different subset of sites each year so that all sites are sampled at the end of 3 to 5 years, most years, b) measure all sites when changes are noted at a subset of sites, c) when altering stocking rates, or d) during extraordinary seasonal conditions. Photographs may also be considered as a quick and easy alternative to provide additional information for sites in years when sampling of vegetation attributes is not undertaken.</p><p>References and Further Reading</p><p>McClaran, M.P., and D.N. Cole. 1993. Packstock in wilderness: Use, impacts, monitoring, and management. General Technical Report INT-301. Intermountain Research Station, United States Department of Agriculture, Forest Service. Ogden, UT. p 14.</p><p>Smith, E.L., and G.B. Ruyle. 1991. Considerations when monitoring rangeland vegetation. G.B. Ruyle. (ed). Some methods for monitoring rangelands and other natural area vegetation. University of Arizona, College of Agriculture, Extension Report 9043. p 3. 18</p><p>Rangeland Inventory, Monitoring, and Evaluation: Sampling Concepts</p><p>Introduction | General Principles | Sampling Concepts | Vegetation Attributes Rangeland Evaluation | Management Applications | Chapter Outline</p><p>Extensive areas are often covered in range inventory or monitoring programs, making it impractical to count every plant or to traverse every meter of land. Therefore, a sample is collected to obtain a subset of data that is assumed to represent the entire site, or population.</p><p>If rangeland vegetation was homogeneous, designing a sampling regime would be fairly straightforward. For example, only a few samples would be needed to obtain an accurate description of a well-manicured lawn. However, rangelands are typically characterized by a diverse assemblage of plant species, some of which are abundant while others may be very rare. Plants are also distributed in a variety of spatial patterns, that may be expressed at several different scales in the landscape. In fact, any factor that affects germination, establishment or mortality will influence the spatial arrangement of plants, including soil type, aspect, water redistribution patterns, grazing distribution (eg., location of fences and water), and wildlife impacts (eg., kangaroo rat mounds). Rangeland plant communities also exhibit considerable temporal variation, so that the presence and abundance of a species may fluctuate markedly between seasons and years.</p><p>Designing effective sampling regimes to accommodate this inherent variability is the real challenge of rangeland inventory or monitoring programs. It is the step that determines the efficiency of sampling, the value of the data, and which statistical models may be applied during data analysis.</p><p> Sampling Terminology Sample Units Allocation of Sample Units - Sampling Designs </p><p>References and Further Reading</p><p>Bonham, C.D. 1989. Measurements for terrestrial vegetation. John Wiley & Son, New York, NY. pp 1-18.</p><p>Cochran, W.G. 1977. Sampling techniques. John Wiley & Sons, New York, NY.</p><p>Cook, C.W., and J. Stubbendieck. (eds). 1986. Range research: Basic problems and techniques. Society for Range Management, Denver, CO. pp 216-250. 19</p><p>Sampling Terminology and Theory</p><p>Much of the terminology and concepts related to sampling is derived from the theory of statistical inference. People often become uncomfortable with statistical theory, but only an elementary understanding is required to understand the general concepts of sampling and data analysis covered here.</p><p> Attribute Population Sample Parameter Statistic Sampling Distributions Sample Mean Sample Variance Standard Error Confidence Interval Accuracy and Bias Precision and Error </p><p>References and Further Reading</p><p>Bonham, C.D. 1989. Measurements for terrestrial vegetation. John Wiley & Son, New York, NY. pp 49-88.</p><p>Cook, C.W., and J. Stubbendieck. (eds). 1986. Range research: Basic problems and techniques. Society for Range Management, Denver, CO. pp 215-216.</p><p>Attributes</p><p>Attributes are the characteristics of the population we wish to measure during sampling, such as species composition, biomass, cover, density, or frequency, at a site. Other attributes of a vegetation community that we may need to describe include plant vigor, habitat quality, soil condition status, erosion potential, etc. Obviously some attributes are easy to measure directly, while others depend on an indirect or subjective assessment of the characteristic.</p><p>References and Further Reading</p><p>Cochran, W.G. 1977. Sampling techniques. John Wiley & Sons, New York, NY.</p><p>20</p><p>Population</p><p>The population is the set of all individuals possessing the particular attribute we wish to describe. Therefore, the limits to the population are decided by the objectives of the sampling program.</p><p>For example, if we wished to conduct a national inventory to determine the volume of commercially valuable timber in a National Forest, the population would comprise all trees of a harvestable size within that National Forest. In another situation, a Bureau of Land Management field officer may want to know the biomass of forage within a particular pasture, to determine its stocking rate over the next 12 months. This time, the population is confined to the forage species within that pasture, excluding the unpalatable or inaccessible material that will not be consumed.</p><p>Because it is usually impractical or impossible to measure all members of the population, a sample is selected and measured to obtain information on the population.</p><p>References and Further Reading</p><p>Cochran, W.G. 1977. Sampling techniques. John Wiley & Sons, New York, NY.</p><p>Dowdy, S. and S. Weardon. 1991. Statistics for research. John Wiley & Sons, New York, NY. 2nd ed. pp 21-22.</p><p>Sample</p><p>A sample is that portion of the population which is actually measured. Sampling is a more practical way to obtain inventory or monitoring information because not all members of the population need to be measured. It permits data to be obtained at less effort and expense, and may enhance its integrity by reducing mistakes associated with the tedium of prolonged repetitive measurements.</p><p>For example, it would be an unreasonable undertaking to measure every tree constituting the population to obtain information for an inventory on the volume of commercially valuable timber in a National Forest! Instead, data on this attribute may be collected using several smaller areas (perhaps 5 or 10 ha) as samples, and then extrapolated to represent the entire commercial timber resource for the National Forest. Likewise, a Bureau of Land Management field officer is not likely to want to cut and weigh all forage plants within a particular pasture to determine the biomass of forage so that the stocking rate for the next 12 months can be estimated. Instead, they are likely to weigh the forage cut from a sample of smaller quadrats (perhaps each only 1m2), and use this information to make some useful conclusions about forage biomass over the entire pasture.</p><p>The key assumption of the sampling process is that sample members make up a representative selection of the entire population. That is, we assume that the information on a particular attribute which we obtained from the sample reliably reflects that attribute 21 in the population. Safeguards incorporated into the sampling process to support this assumption are discussed under Accuracy and Bias and Precision and Error.</p><p>References and Further Reading</p><p>Bonham, C.D. 1989. Measurements for terrestrial vegetation. John Wiley & Son, New York, NY. pp 10-11.</p><p>Cook, C.W., and J. Stubbendieck. (eds.) 1986. Range research: Basic problems and techniques. Society for Range Management, Denver, CO. pp 215-219.</p><p>Parameter</p><p>Values that summarize properties of the population are known as parameters. Commonly expressed parameters are the population mean ( ) and the population variance ( 2).</p><p>Each population has a unique set of parameters, but their actual values are rarely known because of the impracticality of taking a census that includes every member in the population. Instead, data obtained from sampling is used to infer information regarding the parameters of the population.</p><p>We need a simple understanding of population parameters in rangeland inventory or monitoring for two reasons:</p><p>1. The objective of a sampling program is to estimate the parameters without measuring every member of the population. 2. Statistical techniques to analyze differences between treatments, sites, years, etc., are based on comparing parameters from different samples to determine the probability that all data from these different locations and sampling dates are actually derived from the same population. </p><p>References and Further Reading</p><p>Bonham, C.D. 1989. Measurements for terrestrial vegetation. John Wiley & Son, New York, NY. pp 50-58.</p><p>Cochran, W.G. 1977. Sampling techniques. John Wiley & Sons, New York, NY.</p><p>Statistics</p><p>Values which summarize properties of the sample are known as statistics. Commonly expressed statistics are the sample mean ( ) and the sample variance (s2). In sampling, we use statistics derived from the sample data to infer something about the population parameters, since we assume that the sample is representative of the population. 22</p><p>The inferential relationship between population parameters and sample statistics is covered in the discussions of sample mean and sample variance.</p><p>References and Further Reading</p><p>Bonham, C.D. 1989. Measurements for terrestrial vegetation. John Wiley & Son, New York, NY. pp 50-58.</p><p>Sampling Distributions</p><p>Sampling distributions describe general patterns in the spread and frequency of the values in the sample data. The type of sampling distribution generated during sampling depends on the vegetation attribute, spatial patterns of the vegetation, the sampling method, and characteristics of sample unit.</p><p>Sampling distributions have important ramifications on statistical approaches to analyze the data collected in rangeland inventory or monitoring programs. Therefore, specific considerations concerning each vegetation attribute are discussed in statistical analysis of biomass data, statistical analysis of cover data, statistical analysis of density data, and statistical analysis of frequency data.</p><p>There are three common sampling distributions encountered during sampling:</p><p> Binomial Distribution Normal Distribution Poisson Distribution </p><p>References and Further Reading</p><p>Bonham, C.D. 1989. Measurements for terrestrial vegetation. John Wiley & Sons, New York, NY. pp 58-61, 71-81.</p><p>Dowdy, S. and S. Weardon. 1991. Statistics for research. John Wiley & Sons, New York, NY. 2nd ed. pp 161-191.</p><p>Sample Mean</p><p>The sample mean ( ) is the average of a set of values that are sampled from a population. Because vegetation attributes usually exhibit a normal distribution, a greater proportion of the data will lie close to the mean. The sample mean is computed by adding all values in a sample data set and dividing by the number of values in the data set. 23</p><p>The population mean ( ) is calculated in the same manner. However, because we usually aren't able to collect data on all members of the population, we can use the sample mean to estimate the population mean, based on the assumption that they are equal.</p><p>This relationship between the sample mean and the population mean will be valid whenever the attribute being sampled exhibits a normal distribution, and the sampling procedure is free of bias. Safeguards incorporated into the sampling process to protect against bias are discussed under Accuracy and Bias.</p><p>References and Further Reading</p><p>Bonham, C.D. 1989. Measurements for terrestrial vegetation. John Wiley & Son, New York, NY. pp 52-54.</p><p>Cook, C.W., and J. Stubbendieck. (eds). 1986. Range research: Basic problems and techniques. Society for Range Management, Denver, CO. pp 217-219.</p><p>Sample Variance</p><p>The sample variance (s2) is a measure of dispersal that reflects the spread of data around the sample mean. The sample variance is calculated as</p><p>This equation indicates that the variance gives us a measure of dispersal by considering the average absolute difference between each value in the data set and the sample mean.</p><p>We rarely have the need to directly calculate the population variance ( 2), because it is unusual to measure all members of the population. However, we can estimate the population variance from our sample data, from the assumption that</p><p>2 = s2</p><p>References and Further Reading</p><p>Bonham, C.D. 1989. Measurements for terrestrial vegetation. John Wiley & Son, New York, NY. pp 54-58.</p><p>Cook, C.W., and J. Stubbendieck. (eds). 1986. Range research: Basic problems and techniques. Society for Range Management, Denver, CO. pp 217-219. 24</p><p>Standard Error</p><p>The standard error ( ) is a measure of the variability among the sample means ( ) of repeated samples taken from the same population. The standard error is a measure of precision, and reflects how certain we are in the results from our sampling. A small standard error indicates that we expect to get the same result from repeated samples, whereas a large standard error suggests that repeated samples produce highly divergent results.</p><p>The standard error is calculated as</p><p>If we sample a highly variable population (i.e., the value of s in the formula is large) we would expect a large standard error, which demonstrates a low level of repeatability among samples and indicates we should be cautious about how well our sample actually represents the population. By including more members of the population in each sample (i.e., increasing the value of n in the formula), we should reduce the standard error and improve the repeatability among samples</p><p>References and Further Reading</p><p>Bonham, C.D. 1989. Measurements for terrestrial vegetation. John Wiley & Son, New York, NY. p 8.</p><p>Cook, C.W., and J. Stubbendieck. (eds). 1986. Range research: Basic problems and techniques. Society for Range Management, Denver, CO. pp 217-219</p><p>Confidence Interval</p><p>The confidence interval gives a range centered around the sample mean ( ) and it indicates how closely we believe our sample mean is representing the population mean ( ). A large confidence interval suggests that the sample does not provide a precise representation of the population mean, whereas a narrow confidence interval demonstrates a greater degree of precision.</p><p>The formula used to calculate the confidence interval of a sample is</p><p>All confidence intervals are expressed according to a particular probability (also referred to as level of confidence) that the interval correctly includes the population mean. This caveat is necessary because the population parameters remain unknown, but are estimated by theoretical inferences from the sample statistics. Therefore, confidence intervals become wider as we want to be more certain that the population mean is included. The 25 probability level is incorporated into the equation by t or the t-value. Probability levels conventionally assigned to confidence intervals included 80%, 90% or 95%, and can be obtained from t-tables presented in most statistics textbooks. A t-value of 1.96 is used for a 95% confidence interval for normally distributed samples with a sample size greater than 30.</p><p>The other factor influencing confidence interval width is the standard error ( ), which indicates variability of the population and the degree to which it is mediated by sample size.</p><p>Confidence intervals are used in statistical analysis to describe the probability that two sample means are from the same population, provided that the data sets exhibit a normal distribution. If there is overlap between the confidence intervals for two means being compared, then it is concluded that these values are not significantly different with a probability equivalent to the probability used to establish the t-value.</p><p>References and Further Reading</p><p>Bonham, C.D. 1989. Measurements for terrestrial vegetation. John Wiley & Son, New York, NY. pp 64-65.</p><p>Cook, C.W., and J. Stubbendieck. (eds). 1986. Range research: Basic problems and techniques. Society for Range Management, Denver, CO. pp 216-219.</p><p>Accuracy and Bias</p><p>The accuracy of a sample reflects how closely the sample mean ( ) represents the true population mean ( ). Sample accuracy is jeopardized by systematic factors associated with the placement or measurement of sample units, that lead to cumulative errors or biases in the sample mean. These may be caused by inherent flaws in our sampling procedures, or by personal prejudices during data collection.</p><p>Sample accuracy cannot be assessed or corrected after the data is collected, because the true population mean remains unknown (if it were known, we wouldn't be sampling!). The best way to ensure accurate data is to incorporate safeguards into the sampling process that protect against bias. These safeguards include:</p><p> Random Sampling Ground Rules Use of Equipment </p><p>References and Further Reading</p><p>Bonham, C.D. 1989. Measurements for terrestrial vegetation. John Wiley & Son, New York, NY. pp 10-11. 26</p><p>Cook, C.W., and J. Stubbendieck. (eds). 1986. Range research: Basic problems and techniques. Society for Range Management, Denver, CO. pp 215-220.</p><p>Precision and Error</p><p>Precision of a sample can be described as the variation among all the samples used to estimate the population parameters. In this respect it is analogous to the sample variance, and is reflected in the width of the confidence interval of a sample. Precision is affected by various compensating 'errors' associated with inherent variability of the population, as well as random measurement errors, such as possible inconsistent assessments when making estimates, for example, the designation of classes in the Daubenmire cover class method to determine cover, or the designation of ranks in the comparative yield method to determine biomass.</p><p>Precision is an important factor to consider when designing the inventory or monitoring program because the sample variance is the foundation of subsequent statistical analysis. Therefore, although sampling precision can be quantified from the collected data, it is used as an expression of the confidence we have in our collected data. Highly variable samples have a low precision, meaning we are unsure how well our particular sample represents the population.</p><p>The best way to ensure precise data is to incorporate safeguards into the sampling process that protect against errors. These safeguards include:</p><p> Ground Rules Use of Equipment </p><p>References and Further Reading</p><p>Bonham, C.D. 1989. Measurements for terrestrial vegetation. John Wiley & Son, New York, NY. pp 10-11.</p><p>Cook, C.W., and J. Stubbendieck. (eds). 1986. Range research: Basic problems and techniques. Society for Range Management, Denver, CO. pp 215-220.</p><p>Greig-Smith, P. 1983. Quantitative plant ecology. Blackwell Scientific Publications, Oxford.3rd ed. pp 25-26.</p><p>Ground Rules</p><p>Ground rules are guidelines developed to minimize the subjectivity of sampling. Ground rules should be developed before field work commences, and should be clearly included in the sampling protocol. Ground rules are especially important to maintain sampling accuracy and precision when a number of different people are involved in data collection, or when data is collected over a number of years. 27</p><p>References and Further Reading</p><p>Greig-Smith, P. 1983. Quantitative plant ecology. Blackwell Scientific Publications, Oxford.3rd ed. pp 25-26.</p><p>Sample Units</p><p>Sample units are the members of the population from which measurements are taken during sampling. Sample units are distinct and non-overlapping entities, such as quadrats or transects, individual plants, branches within a plant, etc.</p><p>Characteristics of the sample unit have a significant effect on sample accuracy and sample precision (and therefore sampling costs). Sample unit dimensions may also be manipulated to promote a normal distribution in the collected data that will permit the application of conventional analysis of variance models for statistical analysis.</p><p>Types of Sample Units</p><p>The form of the sample unit must be decided during the planning stages for the inventory or monitoring program because the goals of these efforts will require a particular sample unit. The general goal of inventory or monitoring programs is to describe attributes of the vegetation at a site. Therefore, sample units are usually one of the following types of delineated small-scale area within the general area of interest.</p><p> Quadrat Nested Quadrats Transect Plotless </p><p>Other Considerations</p><p>The following aspects of sample units are also important to consider:</p><p> Sample Unit Size Sample Unit Shape Sample Size </p><p>References and Further Reading</p><p>Bonham, C.D. 1989. Measurements for terrestrial vegetation. John Wiley & Son, New York, NY. pp 1-40.</p><p>Kent, M., and P. Croker. 1992. Vegetation description and analysis. Belhaven Press, London. pp 40-56. 28</p><p>Quadrats</p><p>Quadrats are two-dimensional sample units of any size or shape. In some cases, a tape may be laid on the ground at each sampling location to define the quadrat, but more often the quadrat is a frame created from narrow steel or plastic piping and carried from one sampling location to the next. Quadrats are used to measure most vegetation attributes in most vegetation types.</p><p>Permanent quadrats are a useful technique when the site is repeatedly sampled to monitor changes in a vegetation attribute, such as frequency or density. Quadrat location may be marked by pegs or by following a grid system. Although labor-intensive to establish, the permanent siting of quadrats offers the considerable advantage of removing some of the error associated with different placement of the sample unit at each sampling event. Therefore, these techniques are most suitable for small scale studies, but are generally impractical for large scale inventory or monitoring programs.</p><p>References and Further Reading</p><p>Austin, M.P. 1981. Permanent quadrats: An interface for theory and practice. Vegetatio 42:11-21.</p><p>Bonham, C.D. 1989. Measurements for terrestrial vegetation. John Wiley & Son, New York, NY. pp 19-40.</p><p>Goldberg, D.E., and R.M. Turner. 1986. Vegetation change and plant demography in permanent plots in the Sonoran Desert. Ecology 67:695-712.</p><p>Kent, M., and P. Choker. 1992. Vegetation description and analysis. Belhaven Press, London. pp 40-44.</p><p>Roshier, D., Lee, S., and F. Boreland. 1997. A digital technique for recording plant population data in permanent plots. Journal of Range Management 50:106-109.</p><p>Nested Quadrats</p><p>Nested quadrats are a special sample unit that includes a series of different sized quadrats all placed at the same location. They are usually positioned so that all have a common corner point, causing the area sampled by smaller quadrats to be included in the progressively larger ones.</p><p>Nested quadrats are a useful approach when no single sample unit size suits all species that need to be sampled for frequency and density. The nesting allows for the more abundant species to be efficiently assessed in the smaller quadrats while increasing the likelihood of encountering the less common species in the larger quadrats. 29</p><p>Nested quadrats may also be used when planning the sampling protocol to determine the optimum sample unit size for the specific area.</p><p>References and Further Reading</p><p>Bonham, C.D. 1989. Measurements for terrestrial vegetation. John Wiley & Son, New York, NY. pp 19-40.</p><p>Mueller-Dombois and Ellenburg. 1974. Aims and methods of vegetation ecology. John Wiley & Sons, New York, NY. pp 48-50.</p><p>Transects</p><p>Transects are specially shaped sample units having a length dimension that greatly exceeds the width, so that it extends in a continuous manner across the vegetation. Transects are the sample unit of choice when sampling involves larger plants, such as shrubs or trees, because it allows a larger area to be systematically sampled by proceeding along its elongated axis.</p><p>Line transects feature only a length dimension, usually defined by the edge of a tape stretched across the area to be sampled.</p><p>Belt transects have a width dimension to create a sampling area; the length is usually defined by the edge of a stretched tape, while another tape or rule is used to define its width.</p><p>Pace-transects are established when the observer strides along an imaginary line across the site, using their foot placement to determine specific sampling points.</p><p>References and Further Reading</p><p>Bonham, C.D. 1989. Measurements for terrestrial vegetation. John Wiley & Son, New York, NY. pp 19-40.</p><p>Kent, M., and P. Choker. 1992. Vegetation description and analysis. Belhaven Press, London. pp 40-56.</p><p>Plotless Sampling to Determine Cover</p><p>Cover estimated by plotless methods has the advantage of integrating large areas within a single sampling point. Early range assessments relied on the ocular reconnaissance method to estimate cover, where observers traversed the site before subjectively assigning a cover value. This technique generally provided consistent results when practiced by experienced observers, and large areas were surveyed in one day; but extensive training is required and personal bias is difficult to quantify. 30</p><p>A more objective plotless approach is the Variable Plot Method, where cover is assessed using a geometric gauge. This technique has been successfully used in USA rangelands, and is discussed in greater detail as a plotless method to determine cover.</p><p>References and Further Reading</p><p>Bonham, C.D. 1989. Measurements for terrestrial vegetation. John Wiley & Sons, New York, NY. pp 27-31.</p><p>Daubenmire, R. 1968. Plant communities: A textbook on plant synecology. Harper & Row, New York, NY. pp 86-87.</p><p>Mueller-Dombois, D., and H. Ellenburg. 1974. Aims and methods of vegetation ecology. John Wiley & Sons, New York, NY. pp 101-106.</p><p>Sample Unit Size</p><p>The optimum sample unit size for rangeland sampling depends on the attribute being described, the size of plants present, and the scale of spatial patterns within the vegetation. Sometimes the size selected in a rangeland inventory or monitoring program is determined by convention or past practices. In fact, it is important to continue using sample units of the same size for repeated measurements. Therefore, sample unit size must be carefully considered in the planning stages because of its critical role in determining sample accuracy and sample precision.</p><p>Sample unit size influences sample accuracy by controlling the likelihood of boundary decisions. Larger sample units have a lower perimeter:area ratio (Table 1), which reduces possible bias from incorrect boundary decisions.</p><p>Table 1. Effect of size on perimeter:area ratios of sample units </p><p>Dimensions Perimeter:Area Ratio</p><p>1 x 1 4.0</p><p>2 x 2 2.0</p><p>3 x 3 1.3</p><p>4 x 4 1.0</p><p>5 x 5 0.8</p><p>6 x 6 0.7 31</p><p>Sampling precision is influenced by manipulating sample unit size in a manner that considers vegetation patterns, so that more variability is encompassed within sample units rather than among sample units. Larger sample units reduce sample variance and usually generate data that more closely follow a normal distribution. However, these advantages are offset by several other factors. First, the size of the sample units must more than double to reduce sample size by 50%, causing an increase in the total area sampled. Second, the smaller sample size suggested by a lower sample variance would decrease the precision of the sample, due to its effect on the t-value when calculating confidence intervals. Third, sample units with a large area are difficult to methodically count or estimate, which increases the possibility of bias. Finally, the logistical constraints of time and resources generally permit fewer samples to be taken using large sample units, presenting problems of achieving an adequate dispersal of sample units across the site. In practice, the trade-off between fewer, large sample units and many smaller sample units depends on the time taken to measure attributes at each sample unit and the time needed to travel to and locate the additional sample units.</p><p>In summary, sample units should be large enough so that few are completely empty, and so that most include more than one plant. Conversely, inefficient expenditure of time relative to the additional information acquired is apparent in sample units that are larger than necessary. Larger sample units are also needed to accommodate larger life forms, such as bigger shrubs and trees. In contrast, smaller sample units are more convenient in dense vegetation. A system of nested quadrats can overcome problems faced when sampling species of different life form or abundance.</p><p> Specific Guidelines on Sample Unit Size for Biomass Specific Guidelines on Sample Unit Size for Density Specific Guidelines on Sample Unit Size for Frequency </p><p>References and Further Reading</p><p>Bonham, C.D. 1989. Measurements for terrestrial vegetation. John Wiley & Son, New York, NY. pp 33-35.</p><p>Cook, C.W., and J. Stubbendieck. (eds). 1986. Range research: Basic problems and techniques. Society for Range Management, Denver, CO. pp 220-221.</p><p>Daubenmire, R. 1968. Plant communities: A textbook on plant synecology. Harper & Row, New York, NY. pp 85-89.</p><p>Specific Guidelines on Sample Unit Size for Biomass</p><p>The size of sample units is a critical decision in estimating biomass in order to obtain precise data without spending too much time sampling. If too small, the many empty quadrats will give the collected data a skewed sampling distribution, and infrequent species may be missed. Conversely, if quadrats are too large, sampling becomes time 32 consuming and there is a greater likelihood of sampling errors originating from careless evaluation or boundary decisions.</p><p>Also refer to Sample Unit Size in the Sampling Concepts section.</p><p>Sample Unit Size for Biomass Sampling</p><p> increasing quadrat size will reduce the number of emply quadrats, so that collected data assumes a normal distribution. if more than 5% of quadrats are 'empty', increase quadrat size. when sampling involves the use of equipment such as small field scales, ensure that average quadrat biomass is >10 g, to overcome the potential error from insensitive measurement intervals. have quadrats no larger than necessary, to avoid problems associated with careless sampling, boundary decisions, and excessive time requirements. </p><p>References and Further Reading</p><p>(Note: pdf files require Adobe Acrobat (free) to view)</p><p>Bonham, C.D. 1989. Measurements for terrestrial vegetation. John Wiley & Sons, New York, NY. pp 217-226.</p><p>Brummer, J.E., Nichols, J.T., Engel, R.K., and K.M. Eskridge. 1994. Efficiency of different quadrat sizes and shapes for sampling standing crop. Journal of Range Management 47:84-89. (pdf)</p><p>Papanastasis, V.P. 1977. Optimum size and shape of quadrat for sampling herbage weight in grasslands of northern Greece. Journal of Range Management 30:446-449. (pdf)</p><p>Sukhatme, P.V. 1947. The problem of plot size in large-scale yield surveys. Journal of the American Statistical Association 42:297-310.</p><p>Van Dyne, G.M., Vogel, W.G., and H.G. Fisser. 1963. Influence of small plot size and shape on range herbage production estimates. Ecology 44:746-759.</p><p>Weigert, R.G. 1962. The selection of an optimum quadrat size for sampling the standing crop of grasses and forbs. Ecology 43:125-129.</p><p>Wight, J.R. 1967. The sampling unit and its effect on saltbush yield estimates. Journal of Range Management 20:323-325. (pdf)</p><p>Specific Guidelines on Sample Unit Size for Density</p><p>The size of sample units is a critical decision in determining density, so that we can get precise data without spending too much time counting. If too small, the many empty 33 quadrats (or transects) will give the collected data a skewed or Poisson distribution, and conventional statistical inferential techniques cannot be applied. Conversely, if quadrats are too large, counting all individuals becomes time consuming and there is a greater likelihood of sampling errors originating from careless counting.</p><p>Also refer to Sample Unit Size in the Sampling Concepts section.</p><p>Sample Unit Size for Density Sampling</p><p> increasing sample unit size will reduce the number of 'empty' quadrats so that collected data assumes a normal distribution. most efficient sampling is achieved by using size that results in 20% 'empty' quadrats (or transects). if only one size is used, increase sample unit size is there are more 'empty' quadrats than quadrats with one individual. if only one sample unit size is used, aim for an average density of 4 - 10 plants/quadrat. use nested quadrats to measure important specieis exhibiting different abundance or size characteristics. have sample units no larger than necessary, to avoid problems associated with careless counting and excessive time requirements. use the same size sample unit throughout the inventory or monitoring program and avoid comparing data collected from different size quadrats. </p><p>References and Further Reading</p><p>(Note: pdf files require Adobe Acrobat (free) to view)</p><p>Bartlett, M.S. 1948. Determination of plant densities. Nature 162:621.</p><p>Bonham, C.D. 1989. Measurements for terrestrial vegetation. John Wiley & Sons, New York. pp 142-148.</p><p>Barman, F.H.A. 1953. The statistical efficiency of sample plot size and shape in forest ecology. Ecology 34:474-487.</p><p>Eddleman, L.E., Remmenga, E.E., and R.T. Ward. 1964. An evaluation of plot methods for alpine vegetation. Bulletin of the Torrey Botanical Club. 91:439-450.</p><p>Greig-Smith, P. 1983. Quantitative plant ecology. Blackwell Scientific Publications, Oxford.3rd ed. pp. 28-31.</p><p>Heady, H.F. 1958. Vegetational changes in the California annual type. Ecology 39:402- 406. 34</p><p>Lyon, L.J. 1968. An evaluation of density sampling methods in a shrub community. Journal of Range Management 21:16-20. (pdf)</p><p>Mueller-Dombois, D., and H. Ellenburg. 1974. Aims and methods of vegetation ecology. John Wiley & Sons, New York. pp. 69-70.</p><p>Specific Guidelines on Sample Unit Size for Frequency</p><p>Size of sample units is a key decision made in frequency sampling because it has an overwhelming influence on frequency values, as well as sampling precision. For example, large-sized quadrats may result in several common species having 100% frequency, even though their actual abundance differs, and repeated sampling of the same area would have little ability to detect change in frequency values. By contrast, small quadrats may repeatedly miss the less common species, even when present in the area being sampled.</p><p>The problem of obtaining frequency measures for species of differing abundance may be resolved by several approaches. A series of nested quadrats is often adopted when one species dominates the biomass, cover or density of the vegetation. It may also be possible to use species groups for less abundant or insignificant species.</p><p>Also refer to Sample Unit Size in the Sampling Concepts section.</p><p>Sample Unit Size for Frequency Sampling</p><p> frequency values are dependent on quadrat size - legitimate comparisons cannot be made for frequency data obtained from different sized quadrats increasing quadrat size will increase frequency; smaller quadrats will reduce frequency if only one quadrat size is to be used, the most frequent species should occur in 60-85% of the quadrats use nested quadrats to measure a variety of species that exhibit different abundance or size characteristics if the data follows a binomial distribution, ensure that all important species have 20%-80% frequency. Frequency values 5% or 95% cannot be easily distinguished from 0% or 100%, respectively, by the statistical analysis of frequency data using binomial procedures. </p><p>References and Further Reading</p><p>Aberdeen, J.E.C. 1958. The effect of quadrat size, plant size, and plant distribution on frequency estimates in plant ecology. Australian Journal of Botany 6:47-58.</p><p>Despain, D.W., Ogden, P.R., and E.L. Smith. 1991. Plant frequency sampling for monitoring rangelands. In: G.B. Ruyle. (ed). Some methods for monitoring rangelands 35 and other natural area vegetation. University of Arizona, College of Agriculture, Extension Report 9043. pp. 8-12.</p><p>Hironaka, M. 1985. Frequency approaches to monitor rangeland vegetation. In: W.C. Krueger. (chairman). Proceeding 38th Annual Meeting, Society for Range Management, Salt Lake City, UT, February 1985. p. 85.</p><p>Hyder, D.N., Conrad, C.E., Tueller, P.T., Calvin, L.D., Poulton, C.E., and F.A. Sneva. 1963. Frequency sampling of sagebrush-bunchgrass vegetation. Ecology 44:740-746.</p><p>Hyder, D.N., Bement, R.E., Remmenga, E.E. and C. Terwilliger. 1966. Frequency sampling of blue grama range. Journal of Range Management 18:94-98.</p><p>Mueller-Dombois, D., and H. Ellenburg. 1974. Aims and methods of vegetation ecology. John Wiley & Sons, New York, NY. pp. 73-76.</p><p>Sample Unit Shape</p><p>Sample units used to sample vegetation attributes are generally circular, square or rectangular in shape. In a monitoring program, it is essential to use sample units of the same shape for all subsequent or compared measurements. Sometimes the shape that is selected for a study is determined by convention or past practices, although it is a factor that can significantly affect sample accuracy and sample precision.</p><p>The shape of sample units influences sample accuracy by controlling the likelihood of bias associated with boundary decisions. Sampling precision is influenced by manipulating sample unit shape in a manner that considers vegetation patterns, so that more variability is encompassed within rather than among sample units.</p><p>A general principle is that circular sample units enhance sample accuracy, while elongated types of sample units promote sample precision; leading to the rule of thumb that long, narrow sample units are most suitable in sparse or clumped vegetation, but circular sample units are more convenient in dense vegetation with a more uniform spatial distribution.</p><p>This rule of thumb is guided by the following considerations:</p><p> a. Circular sample units have a lower boundary length: area ratio. Fewer boundary decisions are encountered in circular sample units compared to square or rectangular sample units of the same size, improving the accuracy and precision of the sample (Table 1). </p><p>Table 1. Effect of sample unit shape on boundary length </p><p>Sample Unit Shape Area Dimensions Perimeter Area:Perimeter Ratio 36</p><p>(m2) (m) (m)</p><p>Circle 10 3.6 (diameter) 11.2 1 : 1.1</p><p>Square 10 3.2 X 3.2 12.6 1 : 1.3</p><p>Rectangle 10 5.0 X 2.0 14.0 1 : 1.4</p><p>Rectangle 10 50.0 X 0.2 100.4 1 : 10.0 b. Long narrow sample units reduce sample variance. In many vegetation communities, plants feature a patchy spatial pattern. Sampling using a circular sample unit promotes an 'all-or-nothing' situation, where some encompass most of a vegetation patch while others fall in the interspaces between vegetation patches, leading to data with a large sample variance. Under these conditions, it is more desirable to have long narrow sample units which include both the patch and the interspace within their boundaries. c. Long narrow sample units are subject to greater measurement error. Long narrow sample units are difficult to examine in their entirety. As well as the errors arising from boundary decisions, small plants are more likely to be overlooked in long, narrow sample units. Additionally, techniques requiring a subjective estimation or ranking, such as the comparative yield method to estimate biomass, the dry-weight-rank method to determine species composition, or the Daubenmire cover class method to estimate cover, are more difficult to execute in elongated quadrats. </p><p> Specific Guidelines on Sample Unit Shape for Biomass Specific Guidelines on Sample Unit Shape for Density Specific Guidelines on Sample Unit Shape for Frequency </p><p>References and Further Reading</p><p>Bonham, C.D. 1989. Measurements for terrestrial vegetation. John Wiley & Son, New York, NY. pp 33-35.</p><p>Clapham, A.R. 1932. The form of the observational unit in quantitative ecology. Journal of Ecology 20:192-197.</p><p>Cook, C.W., and J. Stubbendieck. (eds). 1986. Range research: Basic problems and techniques. Society for Range Management, Denver, CO. pp 220-221.</p><p>Daubenmire, R. 1968. Plant communities: A textbook on plant synecology. Harper & Row, New York, NY. pp 87-88. 37</p><p>Specific Guidelines on Sample Unit Shape for Biomass</p><p>The shape of sample units is a critical decision in determining biomass in order to obtain accurate and precise estimates of biomass. The smaller sample units used to estimate biomass for herbaceous species are conventionally square or slightly rectangular shaped quadrats. In sparse and patchy vegetation, elongated quadrats may reduce the sample size required to obtain an adequate representation of biomass by decreasing sample variance and improving precision. However, such gains may be counterbalanced by increased errors arising from boundary decisions, and larger confidence intervals during the statistical analysis of biomass data.</p><p>Also refer to Sample Unit Shape in the Sampling Concepts section.</p><p>References and Further Reading</p><p>(Note: pdf files require Adobe Acrobat (free) to view)</p><p>Bonham, C.D. 1989. Measurements for terrestrial vegetation. John Wiley & Sons, New York, NY. pp 217-226.</p><p>Brummer, J.E., Nichols, J.T., Engel, R.K., and K.M. Eskridge. 1994. Efficiency of different quadrat sizes and shapes for sampling standing crop. Journal of Range Management 47:84-89. (pdf)</p><p>Papanastasis, V.P. 1977. Optimum size and shape of quadrat for sampling herbage weight in grasslands of northern Greece. Journal of Range Management 30:446-449. (pdf)</p><p>Pechanec, J.F., and G. Stewart. 1940. Sagebrush-grass range sampling studies: Size and structure of sampling units. Journal of the American Society of Agronomy 32:669-682.</p><p>Van Dyne, G.M., Vogel, W.G., and H.G. Fisser. 1963. Influence of small plot size and shape on range herbage production estimates. Ecology 44:746-759.</p><p>Wight, J.R. 1967. The sampling unit and its effect on saltbush yield estimates. Journal of Range Management 20:323-325. (pdf)</p><p>Specific Guidelines on Sample Unit Shape for Density</p><p>The smaller sample units used to determine the density of herbaceous species are conventionally square or slightly rectangular shaped quadrats. In sparse and patchy vegetation, elongated quadrats may reduce sample size requirements, by decreasing the sample variance and improving precision.</p><p>When bigger sample units are required to obtain density estimates for larger species, particularly trees and shrubs, an extended belt transect is often selected. A belt transect is favored because it is easy to progress along its length to account for the entire area, while 38 methodically ensuring that plants are neither missed nor counted twice. By contrast, belt transects are rarely used as sample units when measuring other attributes, such as biomass or cover, where most techniques require that the entire quadrat can be assessed from a single viewing.</p><p>Also refer to Sample Unit Shape in the Sampling Concepts section.</p><p>References and Further Reading</p><p>(Note: pdf files require Adobe Acrobat (free) to view)</p><p>Bonham, C.D. 1989. Measurements for terrestrial vegetation. John Wiley & Sons, New York. pp. 142-148.</p><p>Eddleman, L.E., Remmenga, E.E., and R.T. Ward. 1964. An evaluation of plot methods for alpine vegetation. Bulletin of the Torrey Botanical Club. 91:439-450.</p><p>Lyon, L.J. 1968. An evaluation of density sampling methods in a shrub community. Journal of Range Management 21:16-20. (pdf)</p><p>Mueller-Dombois, D., and H. Ellenburg. 1974. Aims and methods of vegetation ecology. John Wiley & Sons, New York. pp. 68-70.</p><p>Specific Guidelines on Sample Unit Shape for Frequency</p><p>The effect of the shape of sample units on precision and boundary decisions is highly pertinent to frequency sampling, but quadrats of almost any shape have been used. Much of the monitoring work throughout western USA rangelands have used square quadrats, usually with dimensions of 40 cm x 40 cm. Extended rectangular quadrats would probably not be practical, because the time taken to carefully search for various species would negate the speed advantages generally offered by frequency sampling.</p><p>Also refer to Sample Unit Shape in the Sampling Concepts section.</p><p>References and Further Reading</p><p>Despain, D.W., Ogden, P.R., and E.L. Smith. 1991. Plant frequency sampling for monitoring rangelands. In: G.B. Ruyle. (ed). Some methods for monitoring rangelands and other natural area vegetation. University of Arizona, College of Agriculture, Extension Report 9043. p. 12.</p><p>, M.1985. Frequency approaches to monitor rangeland vegetation. In: W.C. Krueger. (chairman). Proceeding 38th Annual Meeting, Society for Range Management, Salt Lake City, UT, February 1985. p. 85. 39</p><p>Hyder, D.N., Conrad, C.E., Tueller, P.T., Calvin, L.D., Poulton, C.E., and F.A. Sneva. 1963. Frequency sampling of sagebrush-bunchgrass vegetation. Ecology 44:740-746.</p><p>Hyder, D.N., Bement, R.E., Remmenga, E.E. and C. Terwilliger. 1966. Frequency sampling of blue grama range. Journal of Range Management 18:94-98.</p><p>Sample Size</p><p>Sample size, or sampling intensity, refers to the number of sample units that will be measured in the inventory or monitoring program.</p><p>Sample size is an important consideration during the planning stages of a monitoring program because it is a key way to influence the precision of the collected data. Larger sample sizes improve precision because a greater proportion of the population is being measured. For example, consider a bag of 100 marbles, featuring 8 colors in differing quantities. If we only pull 5 marbles from the bag we don't have a very good idea of colors or quantities, because we haven't even had the opportunity to sample each color (ie., 8 colors cannot be fully represented by 5 marbles). If we pull 40 marbles from the bag we get a better idea of colors and quantities, and our confidence in the representativeness of our data will continue to increase as more marbles from the population in the bag are studied.</p><p>Larger sample sizes are required if the population has a large variance or if a species of interest occurs in low abundance. In both cases, a greater proportion of the population must be sampled to obtain an accurate and precise measure of the vegetation attribute.</p><p>There are two possible approaches to determine an adequate sample size when designing an inventory or monitoring program.</p><p> Statistical Techniques Graphing Techniques </p><p>Sample size is a critical decision in designing an inventory or monitoring program because it is an important way to influence the precision of the sample. Special consideration regarding sample size depends on the vegetation attribute being sampled, and the sampling method selected to collect the data.</p><p> Specific Guidelines on Sample Size for Biomass Specific Guidelines on Sample Size for Density Specific Guidelines on Sample Size for Frequency </p><p>References and Further Reading</p><p>(Note: pdf files require Adobe Acrobat (free) to view) 40</p><p>Bonham, C.D. 1989. Measurements for terrestrial vegetation. John Wiley & Son, New York, NY. pp 65-71.</p><p>Larson, L.L., and P.A. Larson. 1987. Use of microsite sampling to reduce inventory sample size. Journal of Range Management 40:378-379. (pdf)</p><p>Pechanec, J.F, and G. Stewart. 1941. Sagebrush-grass range sampling studies: variability of native vegetation and sampling error. Journal of American Society of Agronomy 33:1057-1071.</p><p>Statistical Techniques</p><p>Sample size (n) can be determined using a statistical approach that considers the variability of the population and our requirements for precision. The formula to determine the number of samples needed to meet these statistical criteria is</p><p>The variability in the population is accounted for by s2, or the sample variance. A preliminary sample may be collected, or data from earlier studies in the same vegetation may be used to provide an indication of the expected variability in the population to be sampled. The formula shows the need for more sample units in a highly variable population (when s2 is large). Stratified sampling can help to reduce variability and decrease sample size requirements within a site. Variability may also be reduced by manipulating sample unit size and sample unit shape.</p><p>The t-value serves to weight the equation according to a probability level that reflects the probability that our conclusions are erroneous. If we are willing to accept a greater probability of incorrect assessments, the t-value will be smaller, meaning that fewer samples will be needed. The t-value can be obtained from the t-tables found in most statistics textbooks.</p><p>The k-value of the denominator is the difference between the sample mean and the population mean ( ). Therefore, this component of the formula indicates how closely we want our sample mean to reflect the population mean. The formula shows that demanding a very small margin of error, insisting that the sample mean is very close to the population mean, will increase in the number of sample units that must be measured.</p><p>References and Further Reading</p><p>Bonham, C.D. 1989. Measurements for terrestrial vegetation. John Wiley & Son, New York, NY. pp 65-67. 41</p><p>Graphing Techniques</p><p>Sampling precision is improved by increasing sample size because data describing the vegetation attribute is collected for a greater proportion of the population. At some point, however, we begin to collect information that is repetitious and additional sampling becomes inefficient.</p><p>This point can be identified from a graph that illustrates changes to the sample mean with each additional sample unit. When there are few records comprising a sample, the sample mean will fluctuate widely between points because of the low likelihood that a small sample is representative of the population. However, these fluctuations will dampen as the sample size increases. The actual cut-off point for an adequate sample size is a subjective decision made by the sampler and is based on their perceived trade-off between costs and returns involved with the data collection.</p><p>Data for the graph can be obtained from a preliminary sample, or by examining data as it is collected in the field. In either case, the data should be randomized before graphing in order to scramble possible trends in the data that reflect underlying landscape patterns. A series of running means is calculated by initially calculating the average of two records, then three records, then four records, etc. The running means for each sample size are graphed on the vertical axis and the horizontal axis represents the sample size for each of the running means.</p><p>References and Further Reading</p><p>Daubenmire, R. 1968. Plant communities: A textbook on plant synecology. Harper & Row, New York, NY. pp 89-91.</p><p>Greig-Smith, P. 1983. Quantitative plant ecology. Blackwell Scientific Publications, Oxford.3rd ed. p 31.</p><p>Kershaw, K.A. 1964. Quantitative and dynamic ecology. Edward Arnold Publishing Company, London. pp 68-72.</p><p>Mueller-Dombois, D., and H. Ellenburg. 1974. Aims and methods of vegetation ecology. John Wiley & Sons, New York. pp 77-80.</p><p>Specific Guidelines on Sample Size for Biomass</p><p>Sample size is a critical decision in determining biomass because it is a key way to influence the precision of the collected data. Larger sample sizes are required if the collected data has a large sample variance or if the key species occurs in low abundance. In both cases, a greater proportion of the population must be sampled to obtain an accurate and precise measure of biomass.</p><p>Also refer to Sample Size in the Sampling Concepts section. 42</p><p>Sample Size for Biomass Sampling</p><p> estimate sample size using either statistical techniques or graphing techniques. in most situations, a large sample size (e.g., 50-2000 quadrats) is needed to provide an accurate estimate and to reduce sample variance. </p><p>References and Further Reading</p><p>(Note: pdf files require Adobe Acrobat (free) to view)</p><p>Bonham, C.D. 1989. Measurements for terrestrial vegetation. John Wiley & Sons, New York, NY. pp 65-69, 218.</p><p>Pieper, R.D. 1988. Rangeland vegetation productivity and biomass. In: P.T. Tueller (ed). Vegetation science applications for rangeland analysis and management. Handbook of Vegetation Science, Volume 14. Kluwer Academic Publishers, Dordrecht. pp 453-454.</p><p>Wight, J.R. 1967. The sampling unit and its effect on saltbush yield estimates. Journal of Range Management 20:323-325. (pdf)</p><p>Specific Guidelines on Sample Size for Density</p><p>Sample size is a critical decision for determining density, because it is a key way to influence the precision of the collected data. Larger sample sizes are required if the collected data has a large sample variance or if the key species occurs in low abundance. In both cases, a greater proportion of the population must be sampled to obtain an accurate and precise measure of density.</p><p>Also refer to Sample Size in the Sampling Concepts section.</p><p>Sample Size for Density Sampling</p><p> if the collected data follows a normal distribution, esitmate sample size using either statistical techniques or graphing techniques if the collected data follows a Poisson distribution, sample precision is determined by the total number of individuals counted, rather than the number of sample units in the sample sampling intensity may be set as an arbitrary percentage of the entire area. (For example, it could be decided to sample 1% or 5% of the site area), and sample number depends on the sample unit size selected. </p><p>References and Further Reading</p><p>Bonham, C.D. 1989. Measurements for terrestrial vegetation. John Wiley & Sons, New York, NY. p. 192. 43</p><p>Eddleman, L.E., Remmenga, E.E., and R.T. Ward. 1964. An evaluation of plot methods for alpine vegetation. Bulletin of the Torrey Botanical Club. 91:439-450.</p><p>Greig-Smith, P. 1983. Quantitative plant ecology. Blackwell Scientific Publications, Oxford.3rd ed. pp. 26-32.</p><p>Specific Guidelines on Sample Size for Frequency</p><p>Sample size is a critical decision in determining frequency, because it is a key way to influence the precision of the collected data. Larger sample sizes are required if the collected data has a large sample variance or if the key species occurs in low abundance. In both cases, a greater proportion of the population must be sampled to obtain an accurate and precise measure of frequency.</p><p>From a practical sense, remember that frequency is determined as a percentage. Therefore, if 50 quadrats are recorded, frequency increases in 2% intervals for each quadrat the species is found, so that the outcome can be no more precise than ±1%. For this reason, 100 quadrats are often accepted as the minimum sample size for frequency data, and 200 quadrats is recommended as a sampling intensity that provides a reasonable compromise between statistical and logistical considerations. Many more samples (e.g., 500-1000 quadrats) would be needed if the sampling program aims to detect small changes in frequency.</p><p>Also refer to Sample Size in the Sampling Concepts section.</p><p>Sample Size for Frequency Sampling</p><p> 100 quadrats is the minimum sample size. frequency data from randomly located quadrats usually follows a binomial distribution, so that the conventional statistical technique cannot be used to estimate sample size. a sample size of 100 or 200 quadrats allows the statistical analysis of frequency data based on readily available Binomial Statistics Tables. much larger sample sizes are required to confidently detect small changes between sampling times or sites. </p><p>References and Further Reading</p><p>(Note: pdf files require Adobe Acrobat (free) to view)</p><p>Despain, D.W., Ogden, P.R., and E.L. Smith. 1991. Plant frequency sampling for monitoring rangelands. In: G.B. Ruyle. (ed). Some methods for monitoring rangelands and other natural area vegetation. University of Arizona, College of Agriculture, Extension Report 9043. p. 13. 44</p><p>Hironaka, M. 1985. Frequency approaches to monitor rangeland vegetation. In: W.C. Krueger. (chairman). Proceeding 38th Annual Meeting, Society for Range Management, Salt Lake City, UT, February 1985. p. 85.</p><p>Mueller-Dombois, D., and H. Ellenburg. 1974. Aims and methods of vegetation ecology. John Wiley & Sons, New York, NY. pp. 74-76.</p><p>Whysong, G.L., and W.W. Brady. 1987. Frequency sampling and type II errors. Journal of Range Management 40:472-474. (pdf) </p><p>Allocation of Sample Units - Sampling Designs</p><p>Decisions relating to the arrangement of sample units within the site have a large influence on sample precision, sample efficiency, and statistical models used to analyze the data. These decisions are an important component of designing the inventory or monitoring program before the data is actually collected.</p><p>In vegetation where distinct boundaries of spatial patterns can be identified, sampling precision will be improved by stratified sampling. Under this approach, it is important to ensure that the sample unit is confined within the boundaries of the subunit. For example, when collecting data to determine range condition of a sandy loam upland range site, care must be taken that the transect does not extend into the adjoining loamy upland range site.</p><p>Vegetation often exhibits an identifiable gradient according to slope, soil depth, or distance from water, where the effect of orientation of sample units on sample precision becomes increasingly pertinent. In these situations where the gradient is too subtle to create distinct sampling areas, the sampling precision will be enhanced by orienting the quadrat or transect so it intersects across the gradient, with the aim of encompassing the range of variability within each sample unit.</p><p>Types of Sampling</p><p>The following are the standard sampling techniques used for rangelands:</p><p> Random Sampling Systematic Sampling Selected Sampling Stratified Sampling </p><p>References and Further Reading</p><p>(Note: pdf files require Adobe Acrobat (free) to view)</p><p>Bormann, F.H. 1953. The statistical efficiency of sample plot size and shape in forest ecology. Ecology 34:474-487. 45</p><p>Cook, C.W., and J. Stubbendieck. (eds). 1986. Range Research: Basic Problems and Techniques. Society for Range Management, Denver, CO. pp 221-250.</p><p>Daubenmire, R. 1968. Plant Communities: A Textbook on Plant Synecology. Harper & Row, New York, NY. pp 82-86.</p><p>Greig-Smith, P. 1983. Quantitative Plant Ecology. Blackwell Scientific Publications, Oxford.3rd ed. pp 20-25.</p><p>Hurlbert, S.H. 1984. Pseudoreplication and the design of ecological field experiments. Ecological Monographs 54:187-211.</p><p>Kenkel, N.G., Juhasz-Nagy, P., and J. Podani. 1989. On sampling procedures in population and community ecology. Vegetatio 83:195-207.</p><p>Kent, M., and P. Choker. 1992. Vegetation Description and Analysis. Belhaven Press, London. pp 40-54.</p><p>Wester, D.B. 1992. Viewpoint: replication, randomization, and statistics in range research. Journal of Range Management 45:285-290. (pdf, 990K).</p><p>Random Sampling</p><p>In random sampling, any member of the population has an equal chance of being selected to contribute to the sample. In practice, this means that the set of potential sample units are identified and then the individuals that are actually sampled are selected using a randomization technique, such as throwing a dice, flipping a coin, or using a random number table. For example, 100 contiguous 1m2 quadrats could be identified along a 100m tape, and then 20 of these quadrats are selected from a random number table and measured. Similarly, 10 potential transects could be systematically identified at 10m intervals along a 100m baseline, and 3 of these transects selected for sampling using a deck of cards.</p><p>Random selection of sample units is an underlying assumption of most statistical inference techniques, because it ensures that the sample unit selection is free from personal bias and not confounded by possible spatial patterns within the vegetation. Therefore, a major advantage of adopting random sampling is that data sets can be compared using conventional statistical inference techniques that estimate the sample mean and its precision.</p><p>However, random sampling is often impractical to apply in its purest sense. For example, randomly located quadrats are often difficult to accurately locate, particularly when an extensive area is sampled, and a large proportion of sampling time is devoted to locating the quadrats. In addition, a larger sample size is needed to obtain adequate precision 46 under random sampling, because the technique ensures that all the variability of the population is represented in the sample. Therefore, simple random sampling designs usually feature low sampling efficiency. Finally, random selection processes may lead to an uneven distribution of sample units across the site, so that some areas may be poorly represented during sampling.</p><p>Several designs have been developed to address these disadvantages while maintaining the principles of randomization, including restricted random techniques (arrangement of sample units within blocks or clusters) and stratified sampling.</p><p>References and Further Reading</p><p>(Note: pdf files require Adobe Acrobat (free) to view)</p><p>Cook, C.W., and J. Stubbendieck. (eds). 1986. Range research: Basic problems and techniques. Society for Range Management, Denver, CO. pp 221-223.</p><p>Daubenmire, R. 1968. Plant communities: A textbook on plant synecology. Harper & Row, New York, NY. pp 81-86.</p><p>Dowdy, S. and S. Weardon. 1991. Statistics for research. John Wiley & Sons, New York, NY. 2nd ed. pp 23-25.</p><p>Osborne, J.G. 1942. Sampling errors of systematic and random surveys of cover-type areas. Journal of American Statistics Association 37:257-264.</p><p>Salmon, S.C. 1953. Random versus systematic arrangement of field plots. Agronomy Journal 45:459-462.</p><p>Wester, D.B. 1992. Viewpoint: replication, randomization, and statistics in range research . Journal of Range Management 45:285-290. (pdf)</p><p>Systematic Sampling</p><p>In a systematic sampling design, sample units are selected according to a predetermined methodical pattern, which ensures that each unit of the sample represents an equal portion of the whole population. For example, running transects off a baseline at regular 10m intervals represents a systematic sampling design. Systematic sampling is also commonly used in research situations, when quadrats are located on a grid arrangement throughout the small study area.</p><p>Methodical selection within the site permits sample units to be located more rapidly and more evenly distributed than is the case with random sampling. Sample units are also selected without personal bias, as long as the systematic arrangement is determined without intent to include or exclude certain portions of the population. 47</p><p>The main drawback of systematic sampling is that typical methods of statistical inference cannot be applied, because the assumption of random selection is not met. Even though the observer may be certain that the sampling units were selected free from personal bias, it is possible that the accuracy of the data is compromised by a regular spatial pattern within the vegetation community. For example, sampling according to a 30m grid in a pecan orchard where trees were planted on a 30m spacing could result in estimated cover values of either 0% or 100%, depending on whether the sampling grid coincided with the trees or with the interspaces!</p><p>Various interpretations and modifications have been suggested so that data collected under systematic sampling designs represent an unbiased selection of the population, to ensure valid applications of typical statistical analysis methods. For example, a degree of randomization may be incorporated into the sampling design by tossing a coin to decide whether each regularly spaced transect should be run in a left or right perpendicular direction away from the baseline. In other situations, the scale of spatial patterns within the vegetation community may have been previously investigated (particularly for key species), so a contrasting scale could be adopted when planning the systematic sampling design.</p><p>However, the purist's approach to rangeland inventory and monitoring will insist that only true randomization ensures valid statistical analysis using typical methods. In practice, it remains the responsibility of individual to objectively guarantee the accuracy of the data.</p><p>References and Further Reading</p><p>Cook, C.W., and J. Stubbendieck. (eds). 1986. Range research: Basic problems and techniques. Society for Range Management, Denver, CO. pp 249-250.</p><p>Daubenmire, R. 1968. Plant communities: A textbook on plant synecology. Harper & Row, New York, NY. pp 81-86.</p><p>Osborne, J.G. 1942. Sampling errors of systematic and random surveys of cover-type areas. Journal of American Statistics Association 37:257-264.</p><p>Salmon, S.C. 1953. Random versus systematic arrangement of field plots. Agronomy Journal 45:459-462.</p><p>Selected Sampling</p><p>Selected sampling involves active selection of members of the population that are considered to be most representative of the objectives outlined in the inventory or monitoring program. For example, key areas are often selected for closer attention in utilization studies, because it is not possible to monitor utilization across the entire site. Likewise, in a riparian monitoring program, transects may be subjectively located at several locations considered typical of the riparian zone. 48</p><p>The potential of selected sites to accurately represent the larger area depends upon personal judgement. With experience and skill, people are often able to successfully select representative sites. However, in other cases, personal bias or unrecognized natural factors affecting the area may confound the accuracy of the data from the selected sites. Therefore, extra care must be taken in interpreting the data, in addition to the initial site selection.</p><p>Fewer sites are usually chosen in a selected sampling design, because they are assumed to provide a good representation of the population, and no measure of sample variance is required. As a consequence, the overall sampling effort is greatly reduced, and selected sampling is often adopted in monitoring programs where the primary goal is to investigate change over time for extensive areas. However, because the data provides no measure of variability for the area being sampled, sample precision cannot be estimated and statistical inference analysis cannot be applied to describe spatial patterns fro the general area. However, it is possible to use statistical inference methods to compare values for each site among the various times that the sample units were measured.</p><p>References and Further Reading</p><p>Greig-Smith, P. 1983. Quantitative plant ecology. Blackwell Scientific Publications, Oxford.3rd ed. p 20.</p><p>Kent, M., and P. Choker. 1992. Vegetation description and analysis. Belhaven Press, London. pp 40-44.</p><p>Smith, E.L., and G.B. Ruyle. 1991. Considerations when Monitoring Rangeland Vegetation. In: G.B. Ruyle. (ed). Some methods for monitoring rangelands and other natural area vegetation. University of Arizona, College of Agriculture, Extension Report 9043. pp 2-3.</p><p>Stratified Sampling</p><p>Stratified sampling involves dividing the site into sections that are more homogenous than the entire area. Boundaries of the sections should be based on factors that are readily identified and mapped, such as different vegetation types, soil types, topography, range sites, range condition classes or utilization levels.</p><p>Stratification of the area makes sampling more efficient, because fewer samples are required for a precise estimate of the sample mean and sample variance of a uniform area. Data from each section can be analyzed and interpreted separately, or can be combined to describe the entire management unit. This sampling scheme also overcomes the problem of poor distribution of sample units associated with random sampling.</p><p>In stratified sampling, sample size is usually determined for the entire site, and then sample units are divided among the stratified sections. The number of sample units may be allocated on the basis of the area of each section ('proportional allocation'), or by 49 considering variability within each section so that the attribute is estimated with the same precision for all strata ('optimum allocation').</p><p>References and Further Reading</p><p>Cook, C.W., and J. Stubbendieck. (eds). 1986. Range research: Basic problems and techniques. Society for Range Management, Denver, CO. pp 232-241.</p><p>Rangeland Inventory, Monitoring, and Evaluation: Vegetation Attributes </p><p>Introduction | General Principles | Sampling Concepts | Vegetation Attributes Rangeland Evaluation | Management Applications | Chapter Outline</p><p>Rangeland inventory and monitoring programs have traditionally focused on describing attributes of the vegetation to describe current rangeland status or detect changes over time. The selection of attributes is an important consideration when planning a sampling program.</p><p>The following sections describe the vegetation attributes that are regularly used in rangeland inventory or monitoring programs and discuss the strengths and weaknesses for the many field sampling techniques that are available to measure these attributes.</p><p> Biomass Cover Density Frequency Species Composition </p><p>Biomass</p><p>Biomass is a commonly measured vegetation attribute that refers to the weight of plant material within a given area. Other general terms, such as 'yield' or 'production', are sometimes used interchangeably with biomass. Units to express biomass should be selected so that actual plant weight is easy to visualize, such as lb/acre, kg/ha or g/m2 according to vegetation abundance and objectives of the inventory or monitoring program. 50</p><p>Biomass is one of the most commonly measured attributes in range inventory or monitoring programs. Biomass data may be collected on an individual species basis, as species groups, or as a total weight for the vegetation. Species composition may also be calculated as the contribution (percent by weight) that each species makes to the total biomass.</p><p>Biomass is an attribute that is time consuming and laborious to collect, but easy to interpret. Biomass is regarded as an important indicator of ecological and management processes in the vegetation.</p><p>1. Ecological indicators - biomass is a measure of species dominance within the vegetation, since the demand for resources by each species is largely determined by plant size. Biomass also reflects the amount of energy stored in the vegetation, which can indicate the potential productivity at the site. 2. Management indicators - biomass provides a variety of indicators for rangeland management. For example, it is a valuable tool to assess range condition, the carrying capacity of an area, or to make short-term stocking rate adjustments according to the amount of forage reserves and residual biomass. </p><p>Terminology Related to Biomass Sampling</p><p> Browse Current Year's Growth Forage Productivity Residual Biomass Standing Crop </p><p>Special Considerations for Biomass Sampling</p><p>The following critical issues should be considered when designing sampling protocols to determine biomass.</p><p> Biomass Property Dry Matter Content Sampling Date Separation of Dead and Live Material </p><p>Measurement Techniques and Statistical Analysis</p><p> Methods to Determine Biomass Statistical Analysis of Biomass Data </p><p>References and Further Reading 51</p><p>Bonham, C.D. 1989. Measurements for terrestrial vegetation. John Wiley & Sons, New York, NY. pp 199-264.</p><p>Cook, C.W., and J. Stubbendieck. (eds). 1986. Range research: Basic problems and techniques. Society for Range Management, Denver, CO. pp 51-56.</p><p>Daubenmire, R. 1968. Plant communities: A textbook on plant synecology. Harper & Row, New York, NY. pp 51-53.</p><p>Holechek, J.L., Pieper, R.D., and C.H. Herbel. 1995. Range management principles and practices. Prentice Hall, Englewood Cliffs, NJ. 2nd ed. pp 135-136.</p><p>Pieper, R.D. 1988. Rangeland vegetation productivity and biomass. In: P.T. Tueller. (ed). Vegetation science applications for rangeland analysis and management. Handbook of vegetation science, Volume 14. Kluwer Academic Publishers, Dordrecht. pp 449-467.</p><p>Browse</p><p>Browse is the biomass from trees, shrubs, and woody vines that is available for consumption by livestock and wildlife. It includes leaves and small twigs, but excludes branches and trunks that would not be consumed. Browse is also generally restricted to species or parts of plants deemed palatable to animals, but this issue is confused by the subjective nature of palatability and alternative forage sources.</p><p>References and Further Reading</p><p>(Note: pdf files require Adobe Acrobat (free) to view)</p><p>Bonham, C.D. 1989. Measurements of terrestrial vegetation. John Wiley & Sons, New York, NY. p.14.</p><p>Garrison, G.A. 1953. Annual fluctuations in production of some eastern Oregon and Washington shrubs. Journal of Range Management 6:117-121. (pdf)</p><p>Holechek, J.L., Pieper, R.D., and C.H. Herbel. 1995. Range management principles and practices. Prentice Hall, Englewood Cliffs, NJ. 2nd ed. pp 135-136.</p><p>Society for Range Management. 1989. A glossary of terms used in range management. Society for Range Management. Denver, CO. 3rd ed. p 3.</p><p>Current Year's Growth</p><p>Current year's growth is the above-ground biomass produced during the previous 12 months. 52</p><p>In environments with distinctive seasons, such as the monsoonal influence in the southwest, current year's growth is best approximated at the end of the summer growing season. For grasses, the biomass with a greyish hue is rejected as older than the current year's growth. For evergreen woody species, supple twigs and associated leaves are usually included as recent growth. Obviously, these judgements are subjective, and ground rules that present clear guidelines on the separation of old growth are required to maintain consistency among different observers.</p><p>In situations where climatic conditions promote year-long plant production, or where utilization by grazing animals has already removed some biomass, current year's growth is difficult to determine from data collected at a single time.</p><p>References and Further Reading</p><p>Bonham, C.D. 1989. Measurements for terrestrial vegetation. John Wiley & Sons, New York, NY. pp 241-243.</p><p>Forage</p><p>Forage is biomass that is potential food for livestock and wildlife, including herbaceous and woody sources. Only plants that are available and palatable to grazing animals are classified as forage. Therefore, a highly palatable Arizona cottontop (Digitaria californica) protected from grazing animals by a dense patch of prickly pear (Opuntia spp.) does not contribute to forage. Like browse, forage is also generally restricted to species or parts of plants deemed palatable by animals, but this issue is confused by the subjective nature of palatability and alternative feed sources.</p><p>References and Further Reading</p><p>(Note: pdf files require Adobe Acrobat (free) to view)</p><p>Holechek, J.L., Pieper, R.D., and C.H. Herbel. 1995. Range management principles and practices. Prentice Hall, Englewood Cliffs, NJ. 2nd ed. pp 135-136.</p><p>Schweitzer, S.H., Bryant, F.C., and D.B. Wester. 1993. Potential forage species for deer in the southern mixed prairie. Journal of Range Management 46:70-75. (pdf)</p><p>Society for Range Management. 1989. A glossary of terms used in range management. Society for Range Management. Denver, CO. 3rd ed. p 9.</p><p>Taylor, C.R., Brooks, T.D., and N.E. Garza. 1993. Effects of short duration and high- intensity low-frequency grazing systems on forage production and composition. Journal of Range Management 46:118-121. (pdf) 53</p><p>Productivity</p><p>Productivity refers to the biomass produced at a site over a given period of time. Most vegetation assessments are actually limited to estimating net above-ground primary productivity, by ignoring contributions from consumers (livestock, wildlife, microbial populations, etc.). It also circumvents complicated measurements to account for plant respiration and below-ground contributions.</p><p>Productivity is used to indicate energy and nutrient dynamics in the vegetation. Comparisons of productivity from different areas provide an indication of site potential, but in most rangeland areas, moisture availability has the greatest effect on year-to-year fluctuations in productivity.</p><p>Sampling date is a critical consideration to obtain reliable estimates of productivity.</p><p>References and Further Reading</p><p>(Note: pdf files require Adobe Acrobat (free) to view)</p><p>Biondini, M.E., Lauenroth, W.K., and O.E. Sala. 1991. Correcting estimates of net primary production: Are we overestimating plant production in rangelands. Journal of Range Management 44:194-198. (pdf)</p><p>Daubenmire, R. 1968. Plant communities: A textbook on plant synecology. Harper & Row, New York, NY. pp 51-52.</p><p>Defosse, G.E., and M.B. Bertiller. 1991. Comparison of four methods of grassland productivity assessment based on Festuca pallescens phytomass data. Journal of Range Management 44:199-203. (pdf)</p><p>Holechek, J.L., Pieper, R.D., and C.H. Herbel. 1995. Range management principles and practices. Prentice Hall, Englewood Cliffs, NJ. 2nd ed. pp 135-136.</p><p>Martin, M.H., Cox, J.R., and F.F. Ibarra. 1995. Climatic effects on buffelgrass productivity in the Sonoran Desert. Journal of Range Management 48:60-63.</p><p>Residual Biomass</p><p>Residual biomass refers to the weight of vegetation remaining after grazing is concluded. Residual biomass has important rangeland management ramifications because it acts as the source of future plant growth and as soil protection. For these reasons, residual biomass is a useful goal upon which to interpret utilization and base decisions concerning stocking rates. Residual biomass can be directly sampled using any of the methods to determine biomass.</p><p>References and Further Reading 54</p><p>(Note: pdf files require Adobe Acrobat (free) to view)</p><p>Bartolome, J.W., M.C. Stroud, and H.F. Heady. 1980. Influence of natural mulch on forage production on differing California annual range sites. Journal of Range Management 33:4-8. (pdf)</p><p>Bement, R.E. 1969. A stocking rate guide for beef production on blue-grama range. Journal of Range Management 22:83-86. (pdf)</p><p>Heady, H.F., and R.D. Child. 1994. Rangeland ecology and management. Westview Press, Boulder, CO. pp. 175-179.</p><p>McClaran, M.P., and D.N. Cole. 1993. Packstock in wilderness: Use, impacts, monitoring, and management. General Technical Report INT-301. Intermountain Research Station, United States Department of Agriculture, Forest Service. Ogden, UT. pp. 10-12.</p><p>Standing Crop</p><p>Standing crop is the total above-ground plant biomass on the site at a particular point in time. Standing crop includes current year's production together with that produced in previous years. Standing crop at a site fluctuates within and among years, depending on seasonal conditions and utilization by grazing animals.</p><p>One common objective of rangeland monitoring is to try to determine peak standing crop, or the greatest level of biomass at the site during the year. In environments with clearly defined seasons, peak standing crop coincides with the end of the growing season, such as in early fall following the monsoon rainfall in southern Arizona. Information regarding the peak standing crop is taken as an indicator of yearly productivity and used to estimate carrying capacity.</p><p>References and Further Reading</p><p>(Note: pdf files require Adobe Acrobat (free) to view)</p><p>Bonham, C.D. 1989. Measurements of terrestrial vegetation. John Wiley & Sons. New York, NY. pp 216-217.</p><p>Cassels, D.M., Gillen, R.L., McCollum, F.T., Tate, K.W., and M.E. Hodges. 1995. Effects of grazing management on standing crop dynamics in tallgrass prairie. Journal of Range Management 48:81-84.</p><p>Hufstader, R.W. 1976. Precipitation, temperature, and the standing crop of some southern California grassland species. Journal of Range Management 29:433-435. (pdf) 55</p><p>Holechek, J.L., Pieper, R.D., and C.H. Herbel. 1995. Range management principles and practices. Prentice Hall, Englewood Cliffs, NJ. 2nd ed. pp 135-136.</p><p>Malone, C.R. 1968. Determination of peak standing crop biomass of herbaceous shoots by the harvest method. American Midland Naturalist 79:429-435.</p><p>Ratliff, R.D., Duncan, D.A., and S.E. Westfall. 1991. California oak-woodland overstory species affect herbage understory: Management implications. Journal of Range Management 44:306-310. (pdf)</p><p>Soltero, S., Bryant, F.C., and A. Melgoza. 1989. Standing crop patterns under short duration grazing in northern Mexico. Journal of Range Management 42:20-21. (pdf)</p><p>Smith, M.A., Dodd, J.L., Skinner, Q.D., and J.D. Rodgers. 1993. Dynamics of vegetation along and adjacent to an ephemeral channel. Journal of Range Management 46:56-64. (pdf)</p><p>Biomass Property</p><p>Biomass is a general term that encompasses many special attributes. For example, inventory or monitoring programs focusing on grazing applications may only want to estimate available forage, whereas those addressing ecological perspectives may need to estimate productivity. In some instances, the objectives of the sampling program could be tied to current year's production, while at other times an estimate of dead or alive biomass is most relevant.</p><p>The decision of exactly what to measure depends on sampling objectives and availability of resources, and should be clearly supported by ground rules.</p><p>References and Further Reading</p><p>(Note: pdf files require Adobe Acrobat (free) to view)</p><p>Bonham, C.D. 1989. Measurements of terrestrial vegetation. John Wiley & Sons. New York, NY. pp 216-217.</p><p>Cox, J.R. 1992. Lehmann lovegrass live component biomass and chemical composition. Journal of Range Management 45:523-527. (pdf)</p><p>Martin, M.H., Cox, J.R., and F.F. Ibarra. 1995. Climatic effects on buffelgrass productivity in the Sonoran Desert. Journal of Range Management 48:60-63.</p><p>Dry Matter Content</p><p>Biomass is usually determined on a dry matter basis, which is the weight of plant material after the moisture within the plant material has been extracted. Moisture content varies 56 among species and during the year, according to the stage of growth, growth form (herbaceous, woody, succulent), soil moisture levels, and atmospheric humidity. Standardizing weights on a dry matter basis facilitates comparisons of biomass among sites and over time by eliminating these other confounding factors.</p><p>Dry matter content is determined by drying a sample in an oven, usually at 60° C, until a constant weight is obtained. Since moisture levels differ among species, samples of individual species may have to be dried.</p><p>Methods to determine biomass usually demand a subjective correction for moisture content in the field during data collection. With training and experience, observers are mostly able to adequately correct green plant weight to a dry matter basis. However, some situations including the presence of succulents (eg., Opuntia sp.), fresh growth, or quadrats containing mixtures of woody and herbaceous plants always present a challenge to accurately estimate for dry matter content.</p><p>References and Further Reading</p><p>Bonham, C.D. 1989. Measurements of terrestrial vegetation. John Wiley & Sons, New York, NY. p 201.</p><p>Sampling Date</p><p>Due to the constraints of time and labor, it is unusual to conduct biomass sampling at a site more frequently than once a year. However, total standing crop fluctuates during the year due to physical decomposition, utilization by domestic and wild herbivores, and consumption by insects. Furthermore, species composition and species biomass also vary within the year according to the contrasting growth requirements of warm season and cool season plants, making it difficult to obtain a realistic determination of productivity from a single sampling period.</p><p>Therefore, the time of sampling for a yearly measurement must consider the biomass property to sample, and growth dynamics of key species at the site.</p><p>References and Further Reading</p><p>(Note: pdf files require Adobe Acrobat (free) to view)</p><p>Biondini, M.E., Lauenroth, W.K., and O.E. Sala. 1991. Correcting estimates of net primary production: are we overestimating plant production in rangelands. Journal of Range Management 44:194-198. (pdf)</p><p>Bonham, C.D. 1989. Measurements of terrestrial vegetation. John Wiley & Sons, New York, NY. pp 216-217. 57</p><p>Holechek, J.L., Pieper, R.D., and C.H. Herbel. 1995. Range management principles and practices. Prentice Hall, Englewood Cliffs, NJ. 2nd ed. pp 135-136.</p><p>Kelly, J.M., Van Dyne, G.M., and W.F. Harris. 1974. Comparison of three methods of assessing grassland productivity and biomass dynamics. American Midland Naturalist 92:357-369.</p><p>Malone, C.R. 1968. Determination of peak standing crop biomass of herbaceous shoots by the harvest method. American Midland Naturalist 79:429-435.</p><p>Pieper, R.D., Dwyer, D.D., and R.C. Banner. 1974. Primary production of blue grama grassland in southcentral New Mexico under two soil nitrogen levels. Southwestern Naturalist 20:293-302.</p><p>Willms, W.D., Adams, B.W., and J.F. Dormaar. 1996. Seasonal changes of herbage biomass on the fescue prairie. Journal of Range Management 49:100-104.</p><p>URL: http://cals.arizona.edu/OALS/agnic/knowledge/chapter5/samplingdate.html Last Revised: 29 December 1997</p><p>Separation of Dead and Live Material</p><p>The actual components of above-ground biomass that are considered during sampling depend upon the biomass property to sample. Biomass categories that may be identified include: a. Living, actively growing material. b. Recent dead - current year's growth which is no longer actively growing. c. Old dead - produced during previous growing seasons. d. Litter - detached plant material lying on the soil surface.</p><p>The accuracy and precision of data, whether acquired by harvesting to determine biomass or estimation approaches to determine biomass, are prejudiced by the observer's ability to identify current year's growth, and the level of care devoted to conscientiously and consistently separating components during sampling.</p><p>Other ground rules may be needed to describe the treatment of litter, flowers, fruit, etc.</p><p>References and Further Reading</p><p>(Note: pdf files require Adobe Acrobat (free) to view) 58</p><p>Bonham, C.D. 1989. Measurements of terrestrial vegetation. John Wiley & Sons, New York, NY. pp 201-202.</p><p>Culley, M., Campbell, R.S., and R.H. Canfield. 1933. Values and limitations of clipped quadrats. Ecology 14:35-39.</p><p>Gillen, R.L., and K.W. Tate. 1993. The constituent differential method for determining live and dead herbage. Journal of Range Management 46:142-147. (pdf)</p><p>Johnson, M.K. 1986. Estimating ratios of live and dead plant material in clipped plots. Journal of Range Management 39:90. (pdf)</p><p>Lauenroth, W.K., Dodd, J.L., and C.E. Dickinson. 1980. Aboveground biomass dynamics of blue grama Bouteloua gracilis in a shortgrass steppe and evaluation of a method for separating live and dead. Journal of Range Management 33:210-212. (pdf)</p><p>Martin, M.H., Cox, J.R., and F.F. Ibarra. 1995. Climatic effects on buffelgrass productivity in the Sonoran Desert. Journal of Range Management 48:60-63.</p><p>Pieper, R.D. 1988. Rangeland vegetation productivity and biomass. In: P.T. Tueller. (ed). Vegetation science applications for rangeland analysis and management. Handbook of Vegetation Science, Volume 14. Kluwer Academic Publishers, Dordrecht. p 452.</p><p>Methods to Determine Biomass</p><p>Biomass can be determined using either direct or indirect sampling methods. Direct methods involve techniques that weigh or estimate the actual biomass of plants in quadrats. Indirect methods are based on developing a relationship between plant weight and an easier-to-measure attribute such as plant height, rainfall, or cover.</p><p>The most suitable approach to determine biomass in an inventory or monitoring program depends on the type of vegetation, skills of observers, sample size requirements, and time and budgetary constraints.</p><p> Direct Methods to Determine Biomass Indirect Methods Determine Biomass </p><p>References and Further Reading</p><p>Bonham, C.D. 1989. Measurements of terrestrial vegetation. John Wiley & Sons, New York, NY. pp 33-39.</p><p>Cook, C.W., and J. Stubbendieck. (eds). 1986. Range research: Basic problems and techniques. Society for Range Management, Denver, CO. pp 52-56. 59</p><p>Pieper, R.D. 1988. Rangeland vegetation productivity and biomass. In: P.T. Tueller. (ed). Vegetation science applications for rangeland analysis and management. Handbook of Vegetation Science, Volume 14. Kluwer Academic Publishers, Dordrecht. pp 449-460.</p><p>Direct Methods to Determine Biomass</p><p>Biomass sampling is usually conducted using a sample unit with defined boundaries, for example some type of quadrat, so that biomass can be expressed relative to a known area. With these techniques, the quadrats are directly evaluated to assess biomass. This type of sampling is best suited to areas dominated by herbaceous or shorter shrub species, that can be accommodated in relatively small quadrats.</p><p>The most common direct methods of determining biomass are:</p><p> Harvesting to Determine Biomass Estimation Approaches to Determine Biomass Sample Units for Direct Methods to Determine Biomass </p><p>References and Further Reading</p><p>(Note: pdf files require Adobe Acrobat (free) to view)</p><p>Bonham, C.D. 1989. Measurements of terrestrial vegetation. John Wiley & Sons, New York, NY. pp 33-39.</p><p>Cook, C.W., and J. Stubbendieck. (eds). 1986. Range research: Basic problems and techniques. Society for Range Management, Denver, CO. pp 52-56.</p><p>Pieper, R.D. 1988. Rangeland vegetation productivity and biomass. In: P.T. Tueller. (ed). Vegetation science applications for rangeland analysis and management. Handbook of Vegetation Science, Volume 14. Kluwer Academic Publishers, Dordrecht. pp 449-460.</p><p>White, G.C. 1978. Estimation of plant biomass from quadrat data using the lognormal distribution. Journal of Range Management 31:118-120. (pdf)</p><p>Harvesting to Determine Biomass</p><p>Direct harvesting of vegetation from quadrats of a known size is the most straightforward approach to determine biomass at a site. A wide variety of mechanized clippers, lawn mowers, vacuum collectors, etc, have been invented in attempts to making the task less onerous, but plant material is usually painstakingly gathered using clippers. Data are usually collected from many quadrats located along a transect, so that the transect is the sample unit. Therefore, data must be collected from several transects to determine the precision of the sample, for statistical analysis of biomass data. 60</p><p>When samples are carefully collected, harvesting is regarded as the most accurate method to determine biomass. However, clear ground rules are needed to ensure consistency between observers, including</p><p>1. Boundary decisions - are especially important because sampling should incorporate quadrat volume, leading to potential errors in judgement regarding its vertical boundaries. 2. Clipping height - clipping at ground level is recommended for best repeatability, but clipping at a grazed-height gives a more pertinent measure of forage biomass. Clipping height is most sensitive when a greater proportion of the plant biomass occurs close to the ground such as in herbs or prostrate shrub species. 3. Separation of dead and live material - depending on the biomass property to sample, biomass is removed from most samples before weighing. 4. Species groups - identification and separation of species, whether occurring in the field or in the laboratory, needs to be conscientiously and consistently performed between observers. </p><p>Because each quadrat represents only a very small area of the entire site, sample variance is generally high, and many quadrats must be clipped to obtain a sample size that adequately represents the amount of biomass on the site. Therefore, clipping is very time consuming and not practical for inventory or monitoring purposes over extensive areas. Likewise, the method is destructive and not suited to permanent quadrats. In these situations, estimation approaches to determine biomass or indirect methods to determine biomass are often adopted.</p><p>References and Further Reading</p><p>(Note: pdf files require Adobe Acrobat (free) to view)</p><p>Culley, M., Campbell, R.S., and R.H. Canfield. 1933. Values and limitations of clipped quadrats. Ecology 14:35-39.</p><p>Brummer, J.E., Nichols, J.T., Engel, R.K., and K.M. Eskridge. 1994. Efficiency of different quadrat sizes and shapes for sampling standing crop. Journal of Range Management 47:84-89. (pdf)</p><p>Bonham, C.D. 1989. Measurements of terrestrial vegetation. John Wiley & Sons, New York, NY. pp 201-202.</p><p>Glatzle, A., Mechel, A., and M.E. Vaz Lourenco. 1993. Botanical components of annual Mediterranean grassland as determined by point-intercept and clipping methods. Journal of Range Management. 46:271-274. (pdf)</p><p>Heady, H.F., and G.M. Van Dyne. 1965. Prediction of weight composition from point samples on clipped herbage. Journal of Range Management 18:144-148. (pdf) 61</p><p>Johnson, M.K. 1986. Estimating ratios of live and dead plant material in clipped plots. Journal of Range Management 39:90. (pdf)</p><p>Pieper, R.D. 1988. Rangeland vegetation productivity and biomass. In: P.T. Tueller. (ed). Vegetation science applications for rangeland analysis and management. Handbook of Vegetation Science, Volume 14. Kluwer Academic Publishers, Dordrecht. pp 452-454.</p><p>Singh, J.S., Lauenroth, W.K., and R.K. Steinhorst. 1975. Review and assessment of various techniques for estimating net aerial primary production in grasslands from harvest data. Botanical Review 41:181-232.</p><p>Estimation Approaches to Determine Biomass</p><p>Estimation approaches involve techniques that require observers to visually assess biomass in quadrats, rather than harvesting to determine biomass. Three common estimation methods to determine biomass are commonly used:</p><p> Comparative Yield Method Double Sampling Method Weight-Estimate Method </p><p>References and Further Reading</p><p>Bonham, C.D. 1989. Measurements of terrestrial vegetation. John Wiley & Sons, New York, NY. pp 33-39.</p><p>Cook, C.W., and J. Stubbendieck. (eds). 1986. Range research: Basic rroblems and techniques. Society for Range Management, Denver, CO. pp 52-56.</p><p>Comparative Yield Method</p><p>The comparative yield method was developed by Haydock and Shaw in Australia during the 1970's as a rapid method to determine total biomass when sampling in quadrats. Species biomass is not directly evaluated, but a concurrent estimate of species composition is usually obtained by the dry-weight-rank method. Depending on the objectives of the study, the comparative yield method can be used to describe biomass property. It can be applied to a wide variety of vegetation types, particularly grasslands and shrublands, but becomes more complicated in vegetation with a diverse array of species or life-forms.</p><p>Unlike the weight-estimate method or the doubling sampling method, the comparative yield method the difficulty of determining absolute biomass values. Instead, biomass is scored relative to a set of reference quadrats that are established at the start of sampling. The comparative yield method consists of three stages. 62</p><p>1. Selection of Reference Quadrats - a set of 5 quadrats are established at the start of sampling to reflect the range of biomass scores that will be assigned during sampling, with Score 1 representing a typical low-biomass quadrat, Score 5 representing a typical quadrat in a high-biomass area, and Scores 2, 3, and 4 as intermediate biomass at the site. These quadrats may be clipped and weighed as a training exercise, until a set is obtained that shows a constant increment between quadrats (i.e. indicating a linear biomass relationship). The precision of the method is compromised when the biomass of reference quadrats departs from a linear relationship. To ensure a representative reference, a new set of quadrats should be established at each new site. 2. Assessment of Sample Quadrats - scores are assigned to each quadrat during field sampling, keeping in mind the biomass levels that were present in the set of reference quadrats. Half-scores and scores greater than 5 should be assigned when necessary. Quadrat scores are estimated on the basis of dry matter content, which is often difficult when faced with quadrats that include different growth forms and stages of phenology. Failure of data from the sample quadrats to follow a normal distribution indicates poor selection of sample unit size and/or reference quadrats. 3. Collection of Calibration Quadrats - at the conclusion of sampling, another set of quadrats is scored, clipped, dried, and weighed. The number of samples selected for the calibration data set depends on the observer's ability to furnish accurate visual estimates and the variability of the biomass estimates, but should encompass the range of biomass values and the majority of species encountered during sampling (n ~ 10-20 quadrats). Regression analysis is used to compare scores and harvested values of the calibration samples, which allows data collected from the sample quadrats to be converted to actual biomass. A regression equation reflecting a close linear relationship (preferably passing through the origin), will ensure an accurate and precise biomass estimate. </p><p>The comparative yield method is regularly used for rangeland inventory or monitoring applications where only large differences in biomass need to be identified, such as evaluating range condition or carrying capacity. Furthermore, precision is improved by large sample sizes, so it is more efficient to quickly rank many quadrats rather than linger over the correct rank for any one quadrat. Some observers find it beneficial to carry a set of photographs illustrating the reference quadrats during assessment of the sample quadrats.</p><p>Data is usually collected from multiple quadrats located along a transect, so that the sample unit is each transect. Therefore, data must be collected from several transects to determine the precision of the sample, for statistical analysis of biomass data.</p><p>References and Further Reading</p><p>(Note: pdf files require Adobe Acrobat (free) to view)</p><p>Bureau of Land Management. 1996. Sampling vegetation attributes. Interagency Technical Reference, BLM/RS/ST-96/002+1730. pp 112-121. 63</p><p>Despain, D.W., and E.L. Smith. 1989. The comparative yield method for estimating range production. In: G.B. Ruyle. (ed). Some methods for monitoring reangelands and other natural vegetation. Arizona Cooperative Extension Service Report No. 9043. pp 65-83.</p><p>Friedel, M.H., Chewings, V.H., and G.N. Bastin. 1988. The use of comparative yield and dry-weight-rank techniques in arid rangelands. Journal of Range Management 41:430- 434. (pdf)</p><p>Friedel, M.H., and G.N. Bastin. 1988. Photographic standards for estimating comparative yield in arid rangelands. Australian Rangelands Journal 10:34-38.</p><p>Haydock, K.P., and N.H. Shaw. 1975. The comparative yield method for estimating the dry matter yield of pasture. Australian Journal of Experimental Agriculture and Animal Husbandry 15:663-670.</p><p>Tadmor, N.H., Brieghet, A., Noy-Meir, I., Benjamin, R.W., and E. Eyal. 1975. An evaluation of the calibrated weight-estimate method for measuring production in annual vegetation. Journal of Range Management 28:65-69. (pdf)</p><p>Double Sampling Method</p><p>The double sampling method is designed to determine biomass by sampling in quadrats. Depending on the objectives of the study, the double sampling method can be used to describe any biomass property. It can be applied to a wide variety of vegetation types, particularly grasslands and shrublands, but becomes more complicated in vegetation with a diverse array of species or life-forms.</p><p>The double sampling method was developed as a modification of the weight-estimate method, to attempt to overcome the lack of precision among observers and the possibility of unchecked drift in an individual's estimate of biomass over time. In concordance with the weight-estimate method, data is collected by using defined weight-units for each species to visually estimate the biomass in each quadrat. However, a small second calibration data set is also collected, by clipping and weighing selected quadrats after estimation. Regression analysis is used to compare estimated and harvested values of the calibration samples, to determine if tended to underestimate or overestimate the visual estimation, and to provide the appropriate adjustments to be made to all field samples.</p><p>The number of samples selected for the calibration data set depends on the observer's ability to furnish accurate visual estimates, the sample variance of biomass estimates, the diversity of species on the site, and time restrictions. Ideally, calibration data should encompass the range of biomass values and the majority of species encountered during sampling. Fewer calibration samples are needed when the observer's visual estimates closely reflect harvested weights. However, the observer's proficiency cannot be confirmed until after the calibration quadrats are clipped and weighed! Clipping one out 64 of every 5 - 10 quadrats for inclusion in the calibration data set provides a reliable calibration in most situations.</p><p>Data is usually collected form multiple quadrats located along a transect, so that the transect is the sample unit. Therefore, data must be collected from several transects to determine the precision of the sample, for statistical analysis of biomass data.</p><p>The double sampling method is regularly used to determine biomass in range inventory or monitoring programs. It is a little slower than the weight-estimate method and still requires extensive training in the preliminary stages, but these disadvantages are well compensated by improvements in accuracy and precision. By only clipping a selection of quadrats, it is more efficient than harvesting to determine biomass.</p><p>References and Further Reading</p><p>(Note: pdf files require Adobe Acrobat (free) to view)</p><p>Ahmed, J. and C.D. Bonham. 1982. Optimum allocation in multivariate double sampling for biomass estimation. Journal of Range Management 35:777-779. (pdf)</p><p>Ahmed, J., Bonham, C.D., and W.A. Laycock. 1983. Comparison of techniques used for adjusting biomass estimates by double sampling. Journal of Range Management 36:217- 212. (pdf)</p><p>Bonham, C.D. 1989. Measurements for terrestrial vegetation. John Wiley & Sons, New York, NY. p 205.</p><p>Bureau of Land Management. 1996. Sampling vegetation attributes. Interagency Technical Reference, BLM/RS/ST-96/002+1730. pp 102-111.</p><p>Carande, V., and D.A. Jameson. 1986. Combination of weight estimates with clipped sample data. Journal of Range Management 39:88-89. (pdf)</p><p>Cook, C.W., and J. Stubbendieck. (eds). 1986. Range research: Basic problems and techniques. Society for Range Management, Denver, CO. pp 53-54.</p><p>Riech, R.B., Bonham. C.D., and K. Remington. 1993. Double sampling revisited. Journal of Range Management 46:88-90. (pdf)</p><p>Tadmor, N.H., Brieghet, A., Noy-Meir, I., Benjamin, R.W., and E. Eyal. 1975. An evaluation of the calibrated weight-estimate method for measuring production in annual vegetation. Journal of Range Management 28:65-69. (pdf)</p><p>Wilm, H.G., Costello, D.F., and G.E. Kipple. 1944. Estimating forage yield by the double sampling method. Journal of the American Society of Agronomy 36:194-203. 65</p><p>Weight-Estimate Method</p><p>The weight-estimate method is designed to determine biomass from sampling in quadrats. Depending on the objectives of the study, the weight-estimate method can be used to describe any biomass property. It can be applied to a wide variety of vegetation, particularly grasslands and shrublands, but becomes more complicated in vegetation with a diverse array of species or life-forms.</p><p>Observers must initially learn to visually estimate plant biomass for important species in the vegetation by reiteratively comparing estimated weights against clipped weights. Rather than attempting to directly determine total biomass, observers learn to identify a consistent weight-unit for each species, and then estimate the number of weight-units found in the quadrat. Weight-units should be small enough to easily visualize, yet not so small that estimation becomes arduous or haphazard. Estimations are made simpler by selecting a weight-unit that corresponds to a discrete portion of each species, such as an average sized bunchgrasses or a branch for shrubs.</p><p>Data is usually collected form multiple quadrats located along a transect, so that the transect is the sample unit. Therefore, data must be collected from several transects to determine the precision of the sample, for statistical analysis of biomass data.</p><p>Although the training process is tedious, subsequent sampling concentrates on the rapid visual estimation of biomass with few interruptions for clipping. Biomass is usually assessed on a green-weight basis in the field, but corrected to dry-matter content after drying a subsample of each species.</p><p>Experienced observers are able to obtain fairly accurate results using the weight- estimation technique, particularly in relation to the speed with which data can be collected. For these reasons, it was used extensively to conduct range surveys throughout western rangelands of USA in the 1930's. However, shortcomings of the technique, such as the potential lack of precision among observers, the possibility of unchecked drift in an individual's estimate over time, and a tendency to overestimate quadrats with low biomass while underestimating quadrats with high biomass (leading to artificially reduced variances), make it a less suitable technique when an accurate assessment of biomass is required.</p><p>References and Further Reading</p><p>(Note: pdf files require Adobe Acrobat (free) to view)</p><p>Cook, C.W., and J. Stubbendieck. (eds). 1986. Range research: Basic problems and techniques. Society for Range Management, Denver, CO. pp 53-54.</p><p>Francis, R.C., Van Dyne, G.M., and B.K. Williams. 1979. An evaluation of weight estimation double sampling as a method of botanical analysis. Journal of Environmental Management 8:55-72. 66</p><p>Goebel, C.J. 1955. The weight-estimate at work in southeastern Oregon. Journal of Range Management 8:212-213. (pdf)</p><p>Hutchings, S.S., and J.E. Schmautz. 1969. A field test of the relative weight-estimate method for determining herbage production. Journal of Range Management 22:408-411. (pdf)</p><p>Pechanec, J.F., and G.D. Pickford. 1937. A weight-estimate method for the determination of range or pasture production. Journal of the American Society for Agronomy 29:894- 904.</p><p>Shoop, M.C., and E.H. McIlvain. 1963. The micro-unit forage inventory unit. Journal of Range Management 16:172-179. (pdf)</p><p>Smith, A.D. 1944. A study of the reliability of range vegetation estimates. Ecology 25: 441-448.</p><p>Sample Units for Direct Methods to Determine Biomass</p><p>Direct methods to determine biomass typically involve sampling procedures using a sample unit with defined boundaries (ie., some type of quadrat), so that biomass can be expressed relative to a known area. In this way, the biomass values obtained from a small area are subsequently converted to a more conventional scale, such as kilograms/hectare or pounds/acre.</p><p>When determining biomass, it is important to consider the sample unit used to collect biomass data in its three-dimensional form ie, having height as well as area, to properly account for all plant material within a given area. Therefore, ground rules describing boundary decisions should make clear that many situations can be found where only the part of a plant falling within the quadrat volume will be sampled, regardless of its rooted location.</p><p> Specific Guidelines on Sample Unit Size for Biomass Specific Guidelines on Sample Unit Shape for Biomass Specific Guidelines on Sample Size for Biomass </p><p>References and Further Reading</p><p>(Note: pdf files require Adobe Acrobat (free) to view)</p><p>Bonham, C.D. 1989. Measurements of terrestrial vegetation. John Wiley & Sons, New York, NY. pp 33-40.</p><p>Coleman, S.H. 1959. A useful device for laying out forage production plots. Journal of Range Management 12:138-139. (pdf) 67</p><p>Kennedy, R.K. 1972. The sickledrat: A circular quadrat modification for use in grassland studies. Journal of Range Management 25:312-313. (pdf)</p><p>Indirect Methods to Determine Biomass</p><p>Indirect methods to determine biomass are based on developing a relationship between plant weight and an easier-to-measure attribute such as plant height, rainfall, or cover. This approach is usually performed in three stages. First, preliminary sampling must be conducted to establish the relationship using regression analysis, with biomass as the dependent variable. The preliminary work is then followed by rapid measurement of the indirect attribute in the field. The final stage occurs after the data is collected and it is converted to biomass values using the previously established regression equation.</p><p>Indirect methods may be selected over direct methods to determine biomass in inventory or monitoring programs because they are non-destructive, and usually less time consuming. These approaches are most often used for taller trees and shrubs, and when large sample sizes are required to sample extensive or highly variable areas. The disadvantage of these methods is that the relationships between the indirect attribute and biomass are generally restricted in their applicability to the time and place that the preliminary data was collected.</p><p> Climatic Records Plant Dimensions Reference Unit Method </p><p>References and Further Reading</p><p>Bonham, C.D. 1989. Measurements for terrestrial vegetation. John Wiley & Sons, New York, NY. pp 205-213.</p><p>Cook, C.W., and J. Stubbendieck. (eds). 1986. Range research: Basic problems and techniques. Society for Range Management, Denver, CO. pp 54-55.</p><p>Pieper, R.D. 1988. Rangeland vegetation productivity and biomass. In: P.T. Tueller. (ed). Vegetation science applications for rangeland analysis and management. Handbook of Vegetation Science, Volume 14. Kluwer Academic Publishers, Dordrecht. pp 455-460.</p><p>Climatic Records</p><p>Climatic conditions have an obvious effect on plant growth, especially influencing the biomass of annual and perennial herbaceous species. A strong relationship between rainfall and herbaceous biomass has been developed for many rangeland types, including the semi-arid grasslands of southern Arizona, annual grasslands of California, the cold- deserts of the Great Basin, and the prairies of the Great Plains. In areas with distinctive 68 seasonal rainfall patterns, precipitation during the growing season provides a better relationship than does annual rainfall. For example, more than 91% of the year-to-year variation in the ungrazed standing crop in the grasslands around Tucson, Arizona, is accounted for by rain falling in the June-September growing season. In other environments, the inclusion of additional attributes such as temperature or plant residue levels may improve the prediction of biomass.</p><p>The main drawback of this approach is that rainfall and biomass data must be concurrently gathered for a number of years before the relationship can be developed. The relationship is also specific to the population where the data is collected, and should not be extrapolated to other sites with different soils, topography, temperature, or rainfall patterns. On the otherhand, once the relationship is validated, little additional field work is required. Furthermore, the model can be a useful management tool, whereby future stocking rate decisions may be evaluated by considering possible biomass levels in response to various rainfall scenarios.</p><p>References and Further Reading</p><p>(Note: pdf files require Adobe Acrobat (free) to view)</p><p>Bonham, C.D. 1989. Measurements for terrestrial vegetation. John Wiley & Sons, New York, NY. p 212.</p><p>Cable, D.R. 1975. Influence of precipitation on perennial grass production in the semi- desert southwest. Ecology 56:981-986.</p><p>Currie, P.O., and G. Peterson. 1966. Using growing season precipitation to predict crested wheatgrass yields. Journal of Range Management 19:284-288. (pdf)</p><p>Duncan, D.A., and R.G. Woodmansee. 1975. Forecasting forage yield from precipitation in California's annual rangelands. Journal of Range Management 28:327-329. (pdf)</p><p>George, M.R., Raguse, C.A., Clawson, W.J., Wilson, C.B., Willoughby, R.L., McDougald, N.K., Duncan, D.A., and A.H. Murphy. 1988. Correlation of degree-days with annual herbage yields and livestock gains. Journal of Range Management 41:193- 197. (pdf)</p><p>Pieper, R.D. 1988. Rangeland vegetation productivity and biomass. In: P.T. Tueller. (ed). Vegetation science applications for rangeland analysis and management. Handbook of Vegetation Science, Volume 14. Kluwer Academic Publishers, Dordrecht. pp 455-456.</p><p>Sneva, F., and D.N. Hyder. 1962. Estimating herbage production on semi-arid ranges in the Intermountain Region. Journal of Range Management 15:88-93. (pdf) 69</p><p>Wight, J.R., Hansen, C.L., and D. Whitmer. 1984. Using weather records with a forage production model to forecast range forage production. Journal of Range Management 37:3-6. (pdf)</p><p>Wisiol, K. 1984. Estimating grazingland yield from commonly available data. Journal of Range Management 37:471-475. (pdf)</p><p>Plant Dimensions</p><p>Many different attributes describing plant dimensions can be used to determine plant biomass. Measurements on plant height, plant basal area, twig length and diameter, trunk diameter, canopy cover, or canopy volume, are all used as indirect methods to estimate biomass.</p><p>The method involves initially developing a regression relationship, by recording the appropriate dimensions and harvesting a small number of individuals that are chosen to encompass the range in plant size which will be encountered in the population. This step should be completed before further sampling commences, to ensure that the selected dimensions provide a good prediction of biomass (r2 > 0.70, for example). The main sampling then proceeds by taking only dimension measurements, which are converted to biomass values using the regression equation.</p><p>Although a combination of plant dimensions is generally a better predictor of biomass than a single variable, the objective of designing a simpler sampling strategy can sometimes be lost. For example, although a strong relationship is found between biomass and the multiple factors of stem length, leaf length, and number of stems, the effort required to collect such data would be impractical in most rangeland inventory or monitoring situations!</p><p>This approach is routinely adopted in many rangeland inventory or monitoring situations. It is especially suited to shrubs and trees, which are difficult to estimate by harvesting to determine biomass. For meaningful interpretation, the data must be converted from a biomass per plant to a biomass per area basis, requiring an additional estimate for density. The regression relationship is also specific to the population where the data is collected, and caution must be taken when attempting to apply it to other years or other sites.</p><p>References and Further Reading</p><p>(Note: pdf files require Adobe Acrobat (free) to view)</p><p>Andariese, S.W., and W.W. Covington. 1986. Biomass estimation for four common grass species in northern Arizona ponderosa-pine. Journal of Range Management 39:472-473. (pdf)</p><p>Blankenship, J.O., and D.R. Smith. 1966. Indirect estimation of standing crop. Journal of Range Management 19:74-77. (pdf) 70</p><p>Bonham, C.D. 1989. Measurements for terrestrial vegetation. John Wiley & Sons, New York, NY. pp 208-209, 210-212, 229-255.</p><p>Bryant, F.C., and M.M. Kothmann. 1979. Variability in predicting edible browse from crown volume. Journal of Range Management 32:144-146. (pdf)</p><p>Clark, I. 1945. Variability in growth characteristics of forage plants on summer range in central Utah. Journal of Forestry 43:273-283.</p><p>Ludwig, J.A., Reynolds, J.F., and P.D. Whitson. 1975. Size-biomass relationships of several Chihuahuan desert shrubs. American Midland Naturalist 94:451-461.</p><p>Karl, M.G., and R.A. Nicholson. 1987. Evaluation of the forage-disk method in mixed- grass rangelands of Kansas. Journal of Range Management 40:467-471. (pdf)</p><p>Mitchell, J.E., Elderkin, R., and J.K. Lewis. 1993. Seasonal height-weight dynamics of western wheatgrass. Journal of Range Management 46:147-151. (pdf)</p><p>Murray, R.B., and M.Q. Jacobson. 1982. An evaluation of dimension analysis for predicting shrub biomass. Journal of Range Management 35:451-454. (pdf)</p><p>Pieper, R.D. 1988. Rangeland vegetation productivity and biomass. In: P.T. Tueller. (ed). Vegetation science applications for rangeland analysis and management. Handbook of Vegetation Science, Volume 14. Kluwer Academic Publishers, Dordrecht. pp 458-460.</p><p>Ruyle, G.B., Bowns, J.E., and A.F. Schlundt. 1983. Estimating snowberry ( Symphoricarpos oreophilus ) utilization by sheep from twig diameter-weight relations. Journal of Range Management 36:472-474. (pdf)</p><p>Schuster, J.L. 1965. Estimating browse from twig and stem measurements. Journal of Range Management 18:220-223. (pdf)</p><p>Reference Unit Method</p><p>The reference unit method was developed by Andrew, Noble and Lange in Australia in the 1970s as a non-destructive method to determine biomass. Sampling is usually restricted to a key species, and can describe any biomass property depending on the objectives of the program. The reference unit method is most commonly used to estimate browse biomass of shrubs, but is equally applicable to herbaceous species with discrete growth forms such as bunchgrasses. For meaningful interpretation, the data must be converted from a biomass per plant to a biomass per area basis, requiring an additional estimate of density.</p><p>The reference unit method is a natural evolution of the weight-estimate method and the double-sampling method, in that a standard unit is selected as a biomass comparison for other plants during sampling. The reference unit should reflect 10-20% of the foliage on 71 an average sized plant in the area to be sampled, usually consisting of a shrub branch. However, unlike those methods, the weight of the reference unit is not quantified, so that biomass is estimated according to the number of reference units rather than by plant weight. After sampling, the estimate of biomass is obtained by multiplying the number of reference unit tallies by the weight of the reference unit. Possible bias toward overestimating or underestimating biomass can be corrected by applying regression analysis on a calibration subsample, to compare reference unit counts against harvested biomass.</p><p>References and Further Reading</p><p>(Note: pdf files require Adobe Acrobat (free) to view)</p><p>Andrew, M.H., Noble, I.R., and R.T. Lange. 1979. A non-destructive method for estimating the weight of forage on shrubs. Australian Rangeland Journal 1:225-231.</p><p>Andrew, M.H., Noble, I.R., Lange, R.T., and A.W. Johnson. 1981. The measurement of shrub forage weight: three methods compared. Australian Rangeland Journal 3:74-82.</p><p>Bonham, C.D. 1989. Measurements for Terrestrial Vegetation. John Wiley & Sons, New York, NY. pp 228-229.</p><p>Cabral, D.R., and N.E. West. 1986. Reference unit-based estimates of winterfat browse weights. Journal of Range Management 39:187-189. (pdf)</p><p>Kirmse, R.D., and B.E. Norton. 1985. Comparison of the reference unit method and dimension analysis methods for two large shrubby species in the Caatinga Woodlands. Journal of Range Management 38:425-428. (pdf)</p><p>Statistical Analysis of Biomass Data</p><p>When the layout of quadrats for biomass sampling follows the principles of random sampling, each quadrat represents a sample unit, and values describing biomass in each quadrat form the data set used for statistical analysis. Although this approach is straightforward, it is often an inefficient design because the data usually has a large sample variance.</p><p>More often, quadrat layout follows the principles of systematic sampling, where biomass data is usually collected from multiple quadrats located along a transect. The averages calculated for each transect are the sample units contributing to the data set used for statistical analysis, and usually display a smaller sample variance.</p><p>Biomass data usually follows a normal distribution under either sampling design. Departures from this pattern generally indicate that sample unit size was too small. Significant differences between years or among sites are based on conventional 72 inferential statistics, where two sample means are compared by considering the possibility that their respective confidence intervals overlap.</p><p>References and Further Reading</p><p>Greig-Smith, P. 1983. Quantitative plant ecology. Blackwell Scientific Publications, Oxford. 3rd ed. pp 33-36.</p><p>Remington, K.K., Bonham, C.D., and R.M. Reich. 1992. Blue grama-buffalograss responses to grazing: A Weibull distribution. Journal of Range Management 45:272-276.</p><p>Cover</p><p>Cover is the vertical projection of plant material onto the ground when viewed from above. It is usually expressed as a percentage value. For example, 18% cover indicates a birds-eye-view would reveal 18% of the surface area as vegetative material with the remaining 82% as bare ground. In some forestry situations cover is expressed on an area basis, such as square meters/hectare or square feet/acre.</p><p>Cover is one of the most commonly measured attributes in range inventory and monitoring programs. Many of the methods to determine cover can be conducted simply, quickly, and with acceptable levels of accuracy and precision, making it a favored attribute to survey large areas.</p><p>Cover can be measured for the entire vegetation in an area, or can be applied to individual species. In many areas, overlapping plants can mean more than one species contributes to cover. Decisions involving which species to record depend upon the objectives of the study, and should be clearly described as ground rules during the planning stage. Usually, if ground cover is the attribute of interest only the uppermost component is recorded, whereas basal cover is identified by the species occurring at the soil surface.</p><p>Species composition may also be determined from cover data by calculating the relative contribution of each species to the total cover at the site. Sometimes species composition is obtained by recording all leaf area so that underlying species are not excluded, and this approach can cause the value for total cover to be greater than 100%!</p><p>Cover is regarded as an important indicator of ecological and management processes within the vegetation, though many of the direct relationships still have to be quantified.</p><p>1. Ecological indicators - cover is a characteristic expression of ecological dominance, or the degree of influence a particular species exerts in the vegetation. Although ecological dominance is also a function of species biomass, cover values (eg., leaf area index) provide a close reflection of biomass rankings while being easier to determine. Furthermore, cover allows the species of various life forms to be evaluated together on a comparable basis, in contrast to other 73</p><p> attributes such as density and frequency. Canopy cover is usually most meaningful when considering ecological processes, particularly when woody species are included, because measurements based on basal cover do not adequately reflect the extent of their influence within the vegetation. 2. Management indicators - cover provides a variety of interpretations of direct concern to rangeland management, including erosion potential, the value of wildlife habitat, availability of forage, and trends in range condition. Ground cover is considered the best indicator of protection of the landscape against erosion, whereas canopy cover is commonly used to describe wildlife habitat or related to forage availability. Basal cover provides the most reliable measure for monitoring range trend (particularly when focusing on herbaceous components), because it is less sensitive to fluctuations caused by current seasonal conditions or immediate grazing history. </p><p>Terminology Related to Cover</p><p> Basal Cover Canopy Cover Ground Cover Leaf Area Index </p><p>Measurement Techniques and Statistical Analysis</p><p> Methods to Determine Cover Statistical Analysis of Cover Data </p><p>References and Further Reading</p><p>(Note: pdf files require Adobe Acrobat (free) to view)</p><p>Bonham, C.D. 1989. Measurements for terrestrial vegetation. John Wiley & Sons, New York, NY. pp 96-135.</p><p>Cooper, C.F. 1959. Cover vs. density. Journal of Range Management 12:215. (pdf)</p><p>Greig-Smith, P. 1983. Quantitative plant ecology. Academic Press, New York, NY. pp 5- 9.</p><p>Mueller-Dombois, D., and H. Ellenburg. 1974. Aims and methods of vegetation ecology. John Wiley & Sons, New York, NY. pp 80-92.</p><p>Basal Cover</p><p>Basal cover or basal area is determined by considering the cross-sectional area of plants near the ground, usually taken at a height of 2.5 cm for herbaceous plants and 140 cm for trees. Although basal cover is generally regarded as a more stable measure of cover than 74 canopy cover, particularly for perennial grasses, it can still be sensitive to factors including stage of growth, seasonal conditions, and the history of utilization by herbivores.</p><p>References and Further Reading</p><p>(Note: pdf files require Adobe Acrobat (free) to view)</p><p>Daubenmire, R. 1968. Plant communities: A textbook on plant synecology. Harper & Row, New York, NY. pp 47-49.</p><p>Gibbens, R.P., and R.F. Beck. 1988. Changes in grass basal area and forb densities over a 64-year period on grassland types of the Jornada Experimental Range. Journal of Range Management 41:186-92. (pdf) </p><p>Lister, P.B., and F.X. Shumaker. 1937. The influence of rainfall upon tuft area and height growth of three semidesert range grasses in southern Arizona. Journal Agricultural Research 54:109-121.</p><p>Canopy Cover</p><p>Canopy cover or crown cover describes the area represented by the vertical projection of plant foliage onto the ground. Canopy cover is determined as the perimeter of the plant at its widest horizontal plane. It generally assumes that small gaps within the foliage are included and an average crown perimeter is imagined to smooth irregular edges.</p><p>References and Further Reading</p><p>Daubenmire, R. 1968. Plant communities: A textbook on plant synecology. Harper & Row, New York, NY. pp 42-43.</p><p>Stephenson, S.N., and M.F. Buell. 1965. The reproducibility of shrub cover sampling. Ecology 46:379-380.</p><p>Ground Cover</p><p>Ground cover describes the proportion of the soil surface covered by some type of protective material, which usually includes litter, gravel, rocks, microphytic crusts, in addition to standing plant material. Unprotected bare soil can be determined as</p><p>Bare soil = 100% - Ground Cover</p><p>References and Further Reading 75</p><p>Society for Range Management. 1989. A glossary of terms used in range management. Society for Range Management, Denver, CO. 3rd ed. p 8.</p><p>Leaf Area Index</p><p>Leaf area index is the total area of all leaves of plants relative to the ground surface, within a give area. It is slightly different than a simple measure of canopy cover because it accounts for all layers of vegetation rather than only the top most layer. Leaf area index is expressed as a percentage, which can exceed 100% in abundant vegetation. Leaf area is an indicator of biomass at a site.</p><p>References and Further Reading</p><p>(Note: pdf files require Adobe Acrobat (free) to view)</p><p>Ansley, R.J., Price, D.L., Lawrence, B.K., and P.W. Jacoby. 1988. A truck-mounted mobile screen for photodigital estimation of whole plant leaf area. Journal of Range Management 41:355-358. (pdf)</p><p>Barbour, M.G., Burk, J.H., and W.D. Pitts. 1987. Terrestrial plant ecology. Benjamin Cummins Publishing Co, Menlo Park, CA. 2nd ed. p 161.</p><p>Daubenmire, R. 1968. Plant communities: A textbook on plant synecology. Harper & Row, New York, NY. p 42.</p><p>Svejcar, T. 1990. Root length, leaf area, and biomass of crested wheatgrass and cheatgrass seedlings. Journal of Range Management 43:446-448. (pdf)</p><p>Methods to Determine Cover</p><p>Much effort and imagination have been devoted to developing methods to estimate cover, reflecting its long tradition as an important attribute for rangeland inventory or monitoring purposes. Unlike other attributes such as biomass, density, and frequency, techniques involving sampling in quadrats to determine cover play a minor role. Instead, approaches incorporating points, lines and plotless approaches are more regularly adopted. Many of these techniques are able to assess a larger proportion of the vegetation and integrate the small scale spatial patterns present within each sample unit, which leads to more efficient sampling procedures.</p><p>One of the problems in assessing the accuracy of various methods is that actual cover can never really be determined for a site, unlike density where all plants can be carefully counted or biomass where all plant material can be harvested and weighed. In these situations, the adequacy of a method can only be evaluated by its precision, which is determined by assessing the repeatability of results for a site among observers and over time. 76</p><p>The following techniques are the standard methods of determining cover:</p><p> Point Sampling to Determine Cover Line Sampling to Determine Cover Sampling in Quadrats to Determine Cover Plotless Sampling to Determine Cover </p><p>References and Further Reading</p><p>Bonham, C.D. 1989. Measurements for terrestrial vegetation. John Wiley & Sons, New York, NY. pp 11-12, 56-61.</p><p>Cook, C.W., and J. Stubbendieck. (eds). 1986. Range research: Basic problems and techniques. Society for Range Management, Denver, CO. pp 56-61.</p><p>Greig-Smith, P. 1983. Quantitative plant ecology. Academic Press, New York, NY. pp 5- 9.</p><p>Mueller-Dombois, D., and H. Ellenburg. 1974. Aims and methods of vegetation ecology. John Wiley & Sons, New York, NY. pp 80-92.</p><p>Point Sampling to Determine Cover</p><p>Point sampling is one of the most common approaches to estimate cover of a site, since being conceived by New Zealand pasture scientists, Levy and Madden, in the 1920's. It is based on placing a number of points within an area, and determining the proportion of the points that hit (ie., intercept) vegetation. In this manner, total cover can be calculated as the percentage of hits, relative to the total number of points sampled. Cover of individual species can also be estimated by recording the plant species when intercepted by a point. Species composition is the contribution of hits for each species and is expressed as a percentage of the total number of points where vegetation was recorded as a hit. Ground cover, basal cover, canopy cover, and leaf area index can all be measured by point methods, depending on the ground rules established to guide decisions regarding which species will be recorded when multiple hits are encountered if overlapping canopies are vertically intercepted.</p><p>Point Sampling Methods</p><p>There are three common point sampling methods used in rangeland inventory or monitoring to determine cover:</p><p> Point Frame Method Point Transect Method Step Point Method </p><p>Special Considerations for Point Sampling 77</p><p>These critical issues should be considered when designing sampling protocols involving methods of point sampling to determine cover.</p><p> Angle of Points Arrangement of Points Point Size </p><p>References and Further Reading</p><p>(Note: pdf files require Adobe Acrobat (free) to view)</p><p>Bonham, C.D. 1989. Measurements for terrestrial vegetation. John Wiley & Sons, New York, NY. pp 11-12, 20-24.</p><p>Greig-Smith, P. 1983. Quantitative plant ecology. Academic Press, New York, NY. pp 41-45.</p><p>Hofmann, L., and R.E. Ries. 1990. An evaluation of sample adequacy for point analysis of ground cover. Journal of Range Management 43:545-549. (pdf)</p><p>Levy, E.B., and E.A. Madden. 1933. The point method of pasture analysis. New Zealand Journal of Agriculture 46:267-279.</p><p>Mueller-Dombois, D., and H. Ellenburg. 1974. Aims and methods of vegetation ecology. John Wiley & Sons, New York, NY. pp 84-90.</p><p>Point Frame Method</p><p>The point frame method, based on point sampling to determine cover, was first suggested as an instrument to measure cover by Levy and Madden in the early 1930's. It consists of a standing frame that holds a set of vertically aligned pins, that are lowered as sampling points to record hits. The optimum number and arrangement of points within the frame depends on vegetation spatial patterns, distance between plants, and size of individual plants. A common configuration consists of 10 pins each 5 cm apart. Actual dimensions of the point frame should be selected so that the same plant is not hit by all pins.</p><p>Each point frame is usually considered the sample unit, so commonly cover data can be assessed in 10% intervals. Data from several frames are required for statistical analysis of cover data to compare differences between years or among sites. Sometimes separate data is kept for each pin within the frame, but a possible lack of independence between points can lead to problems in statistical analysis.</p><p>According to the objectives of the study, either ground cover, basal cover, canopy cover, or leaf area index can be determined by this method. A periscope can be attached to the point frame to determine overstory cover. Species composition is also obtained from the point frame technique by recording the species associated with each hit. 78</p><p>The point frame provides reasonably accurate cover data, as long as enough points are observed. It is a much slower technique than sampling using the step point method, but eliminates much of the bias arising from subjective pacing. The point frame is best suited for grasslands and other low-growing vegetation. The point frame becomes impractical in taller shrublands because of difficulties in placing the point frame above tall plants. It is also best suited to vegetation with dense cover, where there is less likelihood that all points within the frame will record bare ground.</p><p>References and Further Reading</p><p>(Note: pdf files require Adobe Acrobat (free) to view)</p><p>Bonham, C.D. 1989. Measurements for terrestrial vegetation. John Wiley & Sons, New York, NY. pp 21-24, 110.</p><p>Bureau of Land Management. 1996. Sampling vegetation attributes. Interagency Technical Reference, B.M./RS/ST-96/002+1730. pp 78-85.</p><p>Fisser, H.G., and G.M. Van Dyne. 1966. Influence of number and spacing of points on accuracy and precision of basal cover estimates. Journal of Range Management 19:205- 211. (pdf)</p><p>Glatzle, A., Mechel, A., and M.E. Vaz Lourenco. 1993. Botanical components of annual Mediterranean grassland as determined by point-intercept and clipping methods. Journal of Range Management 46:271-274. (pdf)</p><p>Goodall, D.W. 1952. Some considerations on the use of point quadrats for the analysis of vegetation. Australian Journal of Scientific Research, Series B. 5:1-41.</p><p>Levy, E.B., and E.A. Madden. 1933. The point method for pasture analysis. New Zealand Journal of Agriculture 46:267-279.</p><p>Morrison, R.G., and G.A. Yarranton. 1970. An instrument for rapid and precise point sampling of vegetation. Canadian Journal of Botany 48:293-297.</p><p>Sharrow, S.H., and D.A. Tober. 1979. A simple, light weight point frame. Journal of Range Management 32:75-76. (pdf)</p><p>Stanton, F.W. 1960. Ocular point frame. Journal of Range Management 13:153. (pdf)</p><p>Point Transect Method</p><p>The point transect method is a technique based on point sampling to determine cover. To follow this method, point readings are taken at either systematic or random locations along a tape that is extended to create a transect across the site. A variety of devices, 79 including sighting tubes, bayonets, and plumb-bobs have been used to ensure a vertical reading of the point through the tape.</p><p>The length and number of sampling points along the transect depend upon the vegetation, but it is usually more efficient to record more transects with fewer points per transect. Each transect is considered a sample unit, and summarized data from several transects are required for statistical analysis of cover data to compare differences among years or sites.</p><p>According to the objectives of the study, either ground cover, basal cover, canopy cover, and species composition can be determined by this method. Leaf area index can also be determined by recording all layers of the vegetation that are hit at each pin placement.</p><p>The point transect method is regularly used for rangeland inventory or monitoring purposes because it is easy to follow. Although it is a slower technique than the step point method, it eliminates much of the bias arising from subjective pacing. Using a tape instead of the point frame method is also less cumbersome in many vegetation types.</p><p>References and Further Reading</p><p>(Note: pdf files require Adobe Acrobat (free) to view)</p><p>Bonham, C.D. 1989. Measurements for terrestrial vegetation. John Wiley & Sons, New York, NY. pp 119-121.</p><p>Bureau of Land Management. 1996. Sampling vegetation attributes. Interagency Technical Reference, B.M./RS/ST-96/002+1730. pp 78-85.</p><p>Fisser, H.G., and G.M. Van Dyne. 1966. Influence of number and spacing of points on accuracy and precision of basal cover estimates. Journal of Range Management 19:205- 211. (pdf)</p><p>Goodall, D.W. 1952. Some considerations on the use of point quadrats for the analysis of vegetation. Australian Journal of Scientific Research, Series B. 5:1-41.</p><p>Heady, H.F., Gibbens, R.P., and R.W. Powell. 1959. A comparison of the charting, line intercept, and line point methods of sampling shrub types of vegetation. Journal of Range Management 12:180-188. (pdf)</p><p>Poissonet, P.S., Daget, P.M., Poissonet, J.A., and G.A. Long. 1972. Rapid point survey by bayonet blade. Journal of Range Management. 25:313. (pdf)</p><p>Step Point Method</p><p>The step point method, an approach based on point sampling to determine cover, was developed by Evans and Love in the 1950's. To implement this procedure, the observer uses a mark placed on the tip of their boot (often a pin or a notch) as the sampling point. 80</p><p>Hits are recorded by identifying whatever falls directly beneath the mark along a paced transect. A distance of 5 paces is commonly recommended between sampling points, although the primary goal is an interval that ensures independence between consecutive observations and rarely includes the same plant. The pace-transect is considered the sample unit, and summarized data from several transects are required for statistical analysis of cover data to compare differences among years or sites.</p><p>According to the objectives of the study, either ground cover, basal cover or canopy cover can be determined by this method. Sometimes the step point method is used to estimate leaf area index by recording several strata in the vegetation, which requires the observer to identify hits within the herbage layer, as well as viewing directly upwards from the boot tip to determine overstory cover. Species composition is also obtained from step point data, although the technique is sometimes modified to include a composition tally if sparse cover precludes the collection of sufficient data to provide an accurate sample of the species present. In these situations, the species occurring closest to the sampling point is recorded as additional information whenever bare ground is hit.</p><p>The step point method is regularly used for rangeland inventory or monitoring purposes because it is easy to learn and rapid to employ, allowing large areas to be quickly described. In homogenous vegetation types, 300-500 points provide an adequate sample; but many more are usually required to estimate the cover of less common species. Also, undesirably large variability often occurs among observers, caused by bias associated with point size and foot placement. Some modifications, including a pointed rod that extends beyond the toe and the wheel point apparatus, have been developed in an attempt to overcome the biases arising from subjective pacing. Nonetheless, in all situations the best results are obtained from the step point method in open grassland communities because maintaining the transect bearing and ensuring objective point placement becomes a challenge in rugged, shrubby, or cactus-dominated areas.</p><p>References and Further Reading</p><p>(Note: pdf files require Adobe Acrobat (free) to view)</p><p>Bonham, C.D. 1989. Measurements for terrestrial vegetation. John Wiley & Sons, New York, NY. pp 121-123.</p><p>Bureau of Land Management. 1996. Sampling vegetation attributes. Interagency Technical Reference, B.M./RS/ST-96/002+1730. pp 70-77.</p><p>Evans, R.A., and R.M. Love. 1957. The step-point method of sampling: A practical tool in range research. Journal of Range Management 10:208-212. (pdf)</p><p>Owensby, C.E. 1973. Modified step-point system for botanical composition and basal cover estimates. Journal of Range Management 26:302-303. (pdf) 81</p><p>Tidmarsh, C.E.M., and C.M. Havenga. 1955. The wheel-point method of survey and measurement of semi-open grasslands and Karoo vegetation in South Africa. Botanical Survey South Africa, Memoir 29. 49 pp.</p><p>Angle of Points</p><p>With most techniques involving point sampling to estimate cover, points are placed vertically to the ground. However, some studies indicate that points inclined at about a 45° angle provide more accurate data for the cover of narrow-leaved species, particularly grasses.</p><p>References and Further Reading</p><p>Bonham, C.D. Measurements of terrestrial vegetation. John Wiley & Sons, New York, NY. p 111-115.</p><p>Greig-Smith, P. 1983. Quantitative plant ecology. Academic Press, New York, NY. pp 41-45.</p><p>Warren-Wilson, J. 1963. Estimation of foliage denseness and foliage angle by inclined point quadrats. Australian Journal of Botany 11:95-105.</p><p>Winkworth, R.E. 1955. The use of point quadrats for the analysis of heath land. Australian Journal of Botany 3:68-81.</p><p>Arrangement of Points</p><p>Observations based on single points, such as the step point method, tend to take less time to record and give a more precise estimate of cover than do the same number of points sampled as a group, such as the point frame method.</p><p>However, this generalization should be reevaluated for each situation, because if individual sampling points are widely spaced or difficult to locate, grouped points can generate time advantages and greater sampling efficiency. Measurements obtained from single points are also more accurate, whereas groups of points may overestimate cover in vegetation with patchy spatial patterns. Randomly located points are more desirable for the statistical analysis of cover data, but systematic allocation following a randomly determined starting point is generally more practical.</p><p>References and Further Reading</p><p>(Note: pdf files require Adobe Acrobat (free) to view)</p><p>Bonham, C.D. Measurements of terrestrial vegetation. John Wiley & Sons, New York, NY. pp 110-111. 82</p><p>Greig-Smith, P. 1983. Quantitative plant ecology. Academic Press, New York, NY. pp 41-45.</p><p>Goodall, D.W. 1952. Some considerations in the use of point quadrats for the analysis of vegetation. Australian Journal of Scientific Research 5:1-41.</p><p>Fisser, H.G., and G.M. Van Dyne. 1966. Influence of number and spacing of points on accuracy and precision of basal cover estimates. Journal of Range Management 19:205- 211. (pdf)</p><p>Kemp, C.D., and A.W. Kemp. 1956. The analysis of point quadrat data. Australian Journal of Botany 4:167-174.</p><p>Point Size</p><p>An underlying assumption in point sampling to determine cover is that the sample unit is theoretically reduced to an infinitely small, dimensionless point. In practice, however, most instruments employed as points possess some dimension, with increasingly large points leading to a greater loss of accuracy by overestimating cover. A wide variety of improvisions have been used as points during field sampling, including pins or sharpened rods, bayonet tips, intersections on a grid-frame, etc. Crosswires of telescopes and sighting scopes probably provide the finest type of point, but their use is often cumbersome. Viewing the ground vertically through the point will also ensure that the point is as small as possible.</p><p>Bias becomes critical if an absolute measure of cover is required, but may be acceptable if the same sized point is used when monitoring differences in cover among sites or over time.</p><p>References and Further Reading</p><p>(Note: pdf files require Adobe Acrobat (free) to view)</p><p>Bonham, C.D. Measurements of terrestrial vegetation. John Wiley & Sons, New York, NY. p.111-115.</p><p>Burzlaff, D.F. 1966. The focal point method of vegetation inventory. Journal of Range Management. 19:222-223. (pdf)</p><p>Cook, C.W., and T.W. Box. 1961. A comparison of the loop and point methods of analyzing vegetation. Journal of Range Management 14:22-27. (pdf)</p><p>Greig-Smith, P. 1983. Quantitative plant ecology. Academic Press, New York, NY. pp 41-45. 83</p><p>Goodall, D.W. 1952. Some considerations in the use of point quadrats for the analysis of vegetation. Australian Journal of Scientific Research 5:1-41</p><p>Poissonet, P.S., Daget, P.M., Poissonet, J.A., and G.A. Long. 1972. Rapid point survey by bayonet blade. Journal of Range Management 25:313. (pdf)</p><p>Warren-Wilson, J. 1963. Errors resulting from thickness of point quadrats. Australian Journal of Botany 11:178-188.</p><p>Winkworth, R.E., and D.W. Goodall. 1962. A cross-wire sighting tube for point-quadrat analysis. Ecology 43:342-343.</p><p>Line Sampling to Determine Cover</p><p>Another common approach to determine cover involves extending a line (usually a tape) across the site and recording the proportion intercepting plant material. In theory, line methods are a specialized form of point sampling to determine cover, where an infinitely large number of points are systematically arranged in a consecutive sequence. Such an order allows points to be located in a more practical manner, and posts can be easily placed at either end to permanently locate the line-transect. In general, greater precision is attained by sampling shorter lines at more locations, provided that each is a length that includes the scale of the spatial pattern within the vegetation.</p><p> More details on the Line Intercept Method </p><p>References and Further Reading</p><p>Bonham, C.D. 1989. Measurements for terrestrial vegetation. John Wiley & Sons, New York, NY. pp 21-24, 117-123.</p><p>Hasel, A.A. 1941. Estimation of vegetation-type areas by linear measurements. Journal of Forestry 39:34-40.</p><p>Line Intercept Method</p><p>The line intercept method was developed by Canfield in the 1940's to estimate cover in the grasslands of southwestern USA and has been widely adopted in rangeland inventory and monitoring applications. To follow this method, a tape is extended to create a transect across the site. The observer proceeds along the line-transect, identifies plants intercepted by the tape, and records intercept distance. Cover is calculated by adding all intercept distances and expressing this total as a proportion of tape length. Each transect is regarded as one sample unit, so multiple transects must be measured to estimate sample variance and conduct statistical analyses of cover data.</p><p>Measurements can be made for either basal cover or canopy cover, according to the objectives of the study. Cover of individual species is easily obtained by collecting 84 intercept data on a species basis. Species composition can be estimated from the proportional representation of each species. Transect length depends on the vegetation and type of plants which are to be measured. In many instances, 15 m transects have been found suitable in dense vegetation, while 30-50 m is needed to obtain a representative sample in sparse vegetation.</p><p>The line intercept method is easy to learn, simple to use, and provides an accurate estimate of cover. In fact, line intercept sampling is often used as the standard comparison when testing other methods to determine cover. Its primary drawback is that sampling can be time consuming, particularly in dense vegetation or when intercept distances are difficult to define because of many gaps or irregular edges within the canopy. Therefore, the line intercept technique is best suited for vegetation characterized by discrete plants, such as bunchgrasses or compact shrubs.</p><p>References and Further Reading</p><p>(Note: pdf files require Adobe Acrobat (free) to view)</p><p>Brun, J.M., and T.W. Box. 1963. A comparison of line intercepts nd random point frames for sampling desert shrub vegetation. Journal of Range Management 16:21-25. (pdf)</p><p>Bureau of Land Management. 1996. Sampling vegetation attributes. Interagency Technical Reference, B.M./RS/ST-96/002+1730. pp 65-69.</p><p>Canfield, R.H. 1941. Application of the line interception method in sampling range vegetation. Journal of Forestry 39:399-394.</p><p>Hanley, T.A. 1978. A comparison of the line-estimation and quadrat estimation methods of determining shrub canopy coverage. Journal of Range Management 31:60-62. (pdf)</p><p>Hormay, A.L. 1949. Getting better records of vegetation changes with line interception method. Journal of Range Management 2:67-69. (pdf)</p><p>Kinsinger, F.E., Eckert, R.E., and P.O. Currie. 1960. A comparison of the line interception, variable plot, and loop methods as used to estimate shrub ground cover. Journal of Range Management 13:17-21. (pdf)</p><p>Parker, K.W., and D.A. Savage. 1944. Reliability of the line interception method in measuring vegetation on the Southern Great Plains. Journal of American Society of Agronomy. 36:97-110.</p><p>Ruthven, D.C., Fulbright, T.E., Beason, S.L., and E.C. Hellgren. 1993. Long-term effects of root plowing on vegetation in the eastern south Texas plains. Journal of Range Management 46:351-354. (pdf) 85</p><p>Sampling in Quadrats to Determine Cover</p><p>Although other approaches are generally preferred, a variety of methods are available to determine cover quadrats. Depending on the technique, measurements can be made for ground cover, basal cover or canopy cover. Species cover and species composition can also be estimated with most methods.</p><p>The general sampling principles involving sample unit shape, sample unit size, and sample size apply to these techniques, and need not be discussed with further. Because each quadrat represents a only a very small area of the site, sample variance is generally high, many quadrats must be taken to obtain a sample size that adequately represents the site.</p><p>Early attempts, mainly for plant demographic studies, involved the labor intensive procedure of mapping quadrats, followed in the office by a second step to estimate cover from the paper charts using an approach of point sampling to determine cover or line sampling to determine cover. Similar procedures followed today have the advantages of modern technology; where quadrats can be initially depicted by vertical photography, and cover digitally estimated using computer-based analysis packages. Although time consuming, these techniques have the advantage of providing additional detailed information indicating the source of changes in cover over time.</p><p>Other techniques have been designed to estimate the area represented by vegetation within the quadrat, by assuming that plants are circular in shape and measuring their diameter. Clear ground rules are needed, particularly guiding boundary decisions, because including material extending outside the quadrat would overestimate cover. In other cases, cover has been quantified by placing a grid over the quadrat, and counting the number of squares dominated by vegetation. In a 10 x 10 grid, each square filled by vegetation represents 1% cover. Conversely, counting those intersections of the grid which 'hit' plants is a type of point sampling to determine cover.</p><p>A third approach to determine cover in quadrats is based on ocular estimation. Some techniques, such as the square-foot-density method and the area-list method, attempt to directly estimate a cover value (e.g., according to 1% or 5% intervals), but the more successful approaches to assess cover adopt broad cover classes. Both approaches rely on extensive training and are vulnerable to the personal bias associated with subjective estimation. However, broad cover classes confer the advantages of speed, somewhat less training, and lower the demand that each quadrat be finitely assessed.</p><p>The Daubenmire Cover Class Method is discussed in greater detail as a method for determining cover using quadrats that has been most widely adopted in the USA for rangeland monitoring.</p><p>References and Further Reading</p><p>(Note: pdf files require Adobe Acrobat (free) to view) 86</p><p>Daubenmire, R.F. 1959. Canopy coverage method of vegetation analysis. Northwest Science 33:43-64.</p><p>Elkson, L. 1942. A comparison of methods quadratting short grass vegetation. Journal of Agricultural Research 64:595-614.</p><p>Goebel, C.J., DeBano, L., and D. Lloyd. 1958. A new method of determining forage cover and production on desert shrub vegetation. Journal of Range Management 11:244- 246. (pdf)</p><p>Hanson, H.C., and D. Love. 1930. Comparison of methods of quadratting. Journal of Ecology 11:743-748.</p><p>Hatton, T.J., West, N.E., and P.S. Johnson. 1986. Relationships of the error associated with ocular estimation and actual total cover. Journal of Range Management 39:91-92. (pdf)</p><p>Pease, K. 1945. An area-list method for measuring range plants. Ecology 16:573-579.</p><p>Poulton, C.E., and T.W. Tisdale. 1961. A quantitative method for the description and classification of range vegetation. Journal of Range Management 14:13-21. (pdf)</p><p>Smith, A.D. 1944. A study of the reliability of range vegetation estimates. Ecology 25:441-448.</p><p>Stewart, G., and S.S. Hutchings. 1936. The point-observation-plot (square-foot-density) method of vegetation assessment. Journal of the American Society of Agronomy 28:714- 727.</p><p>Wells, K.F. 1971. Measuring vegetation changes on fixed quadrats by vertical ground stereography. Journal of Range Management 24:233-236.</p><p>Wright, R.G. 1972. Computer processing of chart quadrat maps and their use in plant demographic studies. Journal of Range Management 25:476-478. (pdf)</p><p>Daubenmire Cover Class Method</p><p>The Daubenmire cover class method was developed in the 1950s by Daubenmire, an ecologist who worked in the forests and rangelands of northwest USA. It is accepted as a relatively accurate method that has been used extensively to determine cover in rangeland situations.</p><p>The technique involves visually designating one of the 6 cover classes (Table 1) to each quadrat. Each species within the quadrat is usually assessed separately. Canopy cover is typically considered, simply because ground cover and basal cover are difficult to estimate if obscured by other plants. Originally, 20 cm x 50 cm quadrats were used, but 87 sample unit size and sample unit shape should be selected to suit the vegetation patterns at the site. Sections of the quadrat frame can also be delineated into various colors, to provide a visual guideline of the area that would represent cover in 10% or 25% increments.</p><p>Table 1. Daubenmire Cover Classes.</p><p>Cover Class Range of Cover Midpoint of Class (%) (%) 1 0 - 5 2.5 2 5 - 25 15.0 3 25 - 50 37.5 4 50 - 75 62.5 5 75 - 95 85.0 6 95 - 100 97.5</p><p>Once sampling is completed, species cover can be estimated by multiplying the number of times a class was recorded by the midpoint of that cover class, adding the results for each class, and calculating an average by dividing by the total number of quadrats sampled. Data is usually collected from many quadrats (n$20) located along a transect, so that the transect is the sample unit. Therefore, data must be collected from several transects to determine the precision of the sample, for statistical analysis of cover data.</p><p>This method recognizes the difficulty in accurately assigning an exact percent cover value to each quadrat, since even highly experienced workers are unlikely to be able to visually estimate closer than about 5% cover! Assigning broad cover classes provides an equally accurate result as long as the data follows a normal distribution around the midpoint within each class. The narrower upper and lower classes of the Daubenmire scale protect against skewed data in extremely sparse or dense vegetation.</p><p>Ranking data into broad classes is also relatively rapid procedure, since observers are not required to spend as much time contemplating quadrat cover to the nearest percent. In fact, rapid evaluation of each quadat is the key to success with this approach, since a large sample is less sensitive to the occasional incorrect ranking. However, the method is still subject to personal bias, and sampling accuracy and precision will benefit if time is devoted to training observers.</p><p>References and Further Reading</p><p>Bailey, A.W., and C.E. Poulton. 1968. Plant communities and environmental relationships in a portion of the Tillamook burn, Northwest Oregon. Ecology 49:1-13.</p><p>Bureau of Land Management. 1996. Sampling vegetation attributes. Interagency Technical Reference, B.M./RS/ST-96/002+1730. pp 55-63. 88</p><p>Cook, C.W., and J. Stubbendieck. (eds) 1986. Range research: Basic problems and techniques. Society for Range Management, Denver, CO. pp 56-57.</p><p>Daubenmire, R.F. 1959. Canopy coverage method of vegetation analysis. Northwest Science 33:43-64.</p><p>Hanson, H.C., and E. Dahl. 1957. Some grassland communities in the mountain-front zone of northern Colorado. Vegetatio 7:249-270.</p><p>Statistical Analysis of Cover Data</p><p>The appropriate statistical model for analyses to identify differences in cover between years or among sites depends on the method to determine cover that was followed, and decisions defining sample units made during preliminary planning.</p><p>If each point is considered an independent sample unit when point sampling to determine cover, the data will follow a binomial distribution, and analyses should be based on binomial statistics. In this situation, binomial confidence intervals are used to assess if two sample means are significantly different. The binomial confidence interval for a given cover value remains constant, according to sample size and the level of probability, and tables listing the width of confidence intervals have been developed for commonly used sample sizes (typically n=100 and n=200) and probability levels (typically p=0.1 or p=0.05). If the confidence intervals (for the correct sample size and probability level) for the sample means being compared overlap, it is concluded that these values are not significantly different.</p><p>In most methods of point sampling to determine cover, however, points are grouped in frames or transects before the raw cover data is derived, and the collected data tends toward a normal distribution. Methods involving line sampling to determine cover, sampling in quadrats to determine cover, or plotless sampling to determine cover also tend to generate normal distributions. In situations where cover data has been collected using broad intervals, such as the point frame method or the Daubenmire cover class method, angular transformations are useful to meet assumptions of a normal distribution. Under normal distributions, significant differences between two sample means are evaluated by considering the possibility that their respective confidence intervals overlap.</p><p>References and Further Reading</p><p>Greig-Smith, P. 1983. Quantitative plant ecology. University of California Press, Berkeley, CA. 3rd ed. pp 45-46.</p><p>89</p><p>Density</p><p>Density describes the number of individual plants in a given area. In situations where identification of individuals is ambiguous, density measurements may be based on some other counting unit, such as culms or shoots for sod-forming grasses or the basal stems for shrubs.</p><p>Units to express density should be selected so that actual plant numbers are easy to visualize. For example, it is more meaningful to express an infrequently occurring shrub as 50 shrubs/ha rather than 0.005 shrubs/m2, whereas 15 seedlings/m2 is easier to imagine than 150,000 seedlings/ha for the density of an abundant annual grass.</p><p>Density is an attribute that is tedious to measure but easy to interpret. It is most often applied to larger plants, such as tress, shrubs, and more prominent perennial forbs. Density is often used as a baseline inventory of the structure of rangeland or forest vegetation, by quantifying different species or various ages within a single species. Density data is also gathered to monitor the effect of various land use treatments, such as plant survival following burning or herbicide application, particularly for woody species.</p><p>Density measurements are sometimes unsuitable for the herbaceous layer, especially when there are numerous plants to count or identification of individuals is difficult. Nonetheless, density is regularly used to evaluate seedling emergence and survival in a rangeland reseeding program. Density is also commonly described in autecological studies, that trace the demography of herbaceous or woody populations.</p><p>Species composition can be determined from density data, by calculating the contribution of each species to the total plant count of the sample. However, the relative density of species of differing sizes provides little information about their actual biomass, ecological dominance or community function. For example, the carpets of annual poppy seedlings that establish following cool season rains in the Sonora Desert may have a high density, yet contribute little to the biomass compared to a less dense, but larger species such as triangle leaf bursage (Ambrosia deltoidea). For this reason, expressions of species composition estimated from density data are usually restricted to a particular life form, such as the shrub layer or herbaceous layer.</p><p>Density can provide useful indicators in an inventory and monitoring program to determine range condition and range trend because it remains relatively stable from year to year, regardless of changes in biomass that result from rainfall fluctuations or short- term grazing patterns.</p><p>Terminology Related to Density</p><p>The following term is associated with sampling to estimate density.</p><p> Mean Area 90</p><p>Special Considerations for Density Sampling</p><p>Here are some critical issues to be considered when designing sampling protocols to determine density:</p><p> Boundary Decisions Identification of Individuals Influence of Spatial Patterns Rooted or Canopy Dimensions Species Groups </p><p>Measurement Techniques and Statistical Analysis</p><p> Methods to Determine Density Statistical Analysis of Density Data </p><p>References and Further Reading</p><p>(Note: pdf files require Adobe Acrobat (free) to view)</p><p>Bonham, C.D. 1989. Measurements for terrestrial vegetation. John Wiley & Sons, New York, NY. pp 127-197.</p><p>Cook, C.W., and J. Stubbendieck. (eds). 1986. Range research: Basic problems and techniques. Society for Range Management, Denver, CO. pp 61-63.</p><p>Cooper, C.F. 1959. Cover vs. density. Journal of Range Management 12:215. (pdf)</p><p>Daubenmire, R. 1968. Plant communities: A textbook on plant synecology. Harper & Row, New York, NY. pp 40-41.</p><p>Greig-Smith, P. 1983. Quantitative plant ecology. Blackwell Scientific Publications, Oxford.3rd ed. pp 26-33.</p><p>Laycock, W.A. 1985. Density as a method for measuring rangeland vegetation. In W.C. Krueger (Chairman) Symposium on use of frequency and density for rangeland monitoring. Proceeding 38th Annual Meeting, Society for Range Management, Salt Lake City, UT, February 1985. pp 91-100.</p><p>Mueller-Dombois, D., and H. Ellenburg. 1974. Aims and methods of vegetation ecology. John Wiley & Sons, New York. pp 68-70. 91</p><p>Mean Area</p><p>Mean area, or the average space available to each plant, is another way to consider density. Mean area is the mathematical reciprocal of density, and is the basis of the various distance methods developed to estimate plant density.</p><p>References and Further Reading</p><p>Bonham, C.D. 1989. Measurements for terrestrial vegetation. John Wiley & Sons, New York, NY. pp 148.</p><p>Boundary Decisions</p><p>Boundary decisions, or edge effects, refer to the problem of deciding whether to include plants intersected by the sample unit boundary. Boundary decisions are more frequently encountered in vegetation with abundant plant populations, and are a less significant issue when sampling areas with sparse plant abundance. The probability of having to boundary decision problems is also influenced by sample unit size and sample unit shape because these characteristics determine the actual boundary length relative to the area encompassed by the boundary of the quadrat or transect.</p><p>A consistent personal inclination to admit or reject boundary plants will compromise sample accuracy, either overestimating (due to consistent admission) or underestimating (due to consistent omission) the attribute being measured. The accuracy of vegetation attributes measured by counting, such as frequency or density, are particularly susceptible to bias arising from boundary decisions. Data obtained by direct methods to determine biomass can also be strongly biased by boundary decisions, because all vertical sides of the quadrat must be considered.</p><p>Clearly defined ground rules help mediate some of the inaccuracies caused by boundary decisions. Some people decide to admit plants with half or more of their basal area within the sample unit. Others may decide to include all plants intersected by the right and upper boundaries of the sample unit, while rejecting plants touching the two remaining sides.</p><p>References and Further Reading</p><p>Sukhatme, P.V. 1947. The problem of plot size in large-scale yield surveys. Journal of American Statistics Association 42:297-310.</p><p>Identification of Individuals</p><p>Attributes that involve counting, particularly density, are subject to error in situations where it is difficult to distinguish discrete individual plants. Problems are most frequently 92 confronted with stoloniferous or rhizomatous grasses, such as black grama (Bouteloua eriopoda) or tobosa (Hilaria mutica) but can also be encountered when mature bunchgrass tussocks break apart. Woody plants that feature multiple stems, such as duned mesquite (Prosopis torreyana) or vegetative propagation, such as aspen (Populus tremuloides) present similar challenges.</p><p>In these cases, selection of alternative counting units such as stalks, culms, tillers, or stems, should be based on morphological characteristics of the species and the objectives of the study. Clear ground rules defining the counting unit are needed to reduce personal bias and provide repeatable data among observers and over time.</p><p>References and Further Reading</p><p>(Note: pdf files require Adobe Acrobat (free) to view)</p><p>Bartos, D.L., Brown, J.K., and G.D. Booth. 1994. Twelve years biomass response in aspen communities following fire. Journal of Range Management 47:79-83. (pdf)</p><p>Bonham, C.D. 1989. Measurements of terrestrial vegetation. John Wiley & Sons, New York, NY. pp 138-140.</p><p>Kalmbacher, R.S., Martin, F.G., and J.E. Rechcigl. 1993. Effect of N-P-K fertilization on yield and tiller density of creeping bluestem. Journal of Range Management 46:452-457. (pdf)</p><p>Mueller-Dombois, D., and H. Ellenburg. 1974. Aims and methods of vegetation ecology. John Wiley & Sons, New York. pp 68-69.</p><p>Influence of Spatial Patterns</p><p>Spatial patterns exhibited by the species we want to sample have an important influence on the suitability of certain methods to determine density, and the sampling distribution of the collected data. Most theories associated with sampling to determine density assumes the species have a random spatial pattern, but often rangeland vegetation assumes a clumped or patchy pattern.</p><p>If counting individual plants, sample unit size can be manipulated to ensure that the collected data follows a normal distribution, regardless of underlying spatial patterns. However, some of the distance methods are sensitive to bias when the species being sampled does not exhibit a random spatial pattern.</p><p>References and Further Reading</p><p>Bonham, C.D. 1989. Measurements for terrestrial vegetation. John Wiley & Sons, New York, NY. pp 127-197. 93</p><p>Greig-Smith, P. 1983. Quantitative plant ecology. Blackwell Scientific Publications, Oxford.3rd ed. pp 26-33.</p><p>Rooted or Canopy Dimensions</p><p>The ground rule for determining the frequency of herbaceous species is conventionally based on the presence of the base of the plant in the sample unit (i.e., rooted frequency). In some cases, particularly for shrubs, inclusion of a plant is based on canopy overhanging the quadrat (i.e., canopy frequency). This approach allows the same sized sample unit to be used to measure small and abundant herbaceous species as well as larger shrubby plants.</p><p>However, density measurements of herbaceous or woody species should be restricted to those plants found to be rooted in the sample unit, because also counting overhanging plants would bias the result by causing an effective increase in sample unit size.</p><p>References and Further Reading</p><p>Despain, D.W., Ogden, P.R., and E.L. Smith. 1991. Plant frequency sampling for monitoring rangelands. In: G.B. Ruyle. (ed). Some methods for monitoring rangelands and other natural area vegetation. University of Arizona, College of Agriculture, Extension Report 9043. p 13.</p><p>Laycock, W.A. 1985. Density as a method for measuring rangeland vegetation. In W.C. Krueger. (Chairman). Symposium on use of frequency and density for rangeland monitoring. Proceeding 38th Annual Meeting, Society for Range Management, Salt Lake City, UT, February 1985. p 93.</p><p>Greig-Smith, P. 1983. Quantitative plant ecology. Blackwell Scientific Publications, Oxford. 3rd ed. p 10, 17.</p><p>Species Groups</p><p>Considering each individual species at the site, even to determine species composition, is often a time consuming and inefficient approach to collect data, especially for attributes determined by counting, such as frequency or density. Accuracy can be compromised from species misidentification (particularly for seedlings, grazed, or rare plants), and such detailed information may be unnecessary to satisfy many inventory or monitoring objectives. Many statistical inference procedures are also ineffective when a species is rarely recorded.</p><p>Instead, many studies focus on close inspection of a few key species that are nominated to meet the goals of the sampling program. Remaining species of interest can be allocated to broad groups that are based on either morphological characteristics, eg., Aristida sp., or functional features, eg., perennial forage grasses or succulents. Clear ground rules are needed to ensure that species are consistently designated to their correct group. 94</p><p>References and Further Reading</p><p>(Note: pdf files require Adobe Acrobat (free) to view)</p><p>Bartos, D.L., Brown, J.L., and G.D. Booth. 1994. Twelve years of biomass response in aspen communities following fire. Journal of Range Management 47:79-83. (pdf)</p><p>Mueller-Dombois, D., and H. Ellenburg. 1974. Aims and methods of vegetation ecology. John Wiley & Sons, New York. pp. 140-145.</p><p>Haferkamp, M.R., Volesky, J.D., Borman, M.M., and Heitschmidt, R.K., and P.O. Currie. 1993. Effects of mechanical treatments and climatic factors on the productivity of Northern Great Plains rangelands. Journal of Range Management 46:346-350. (pdf)</p><p>Hartnett, D.C., Hickman, K.R., and L.E. Walter. 1996. Effects of bison grazing, fire, and topography on floristic diversity in tallgrass prairie. Journal of Range Management 49:413-420.</p><p>Jameson, D.A. 1991. Effects of a single season and rotation harvesting on cool- and warm-season grasses of a mountain grassland. Journal of Range Management 44:327- 329. (pdf)</p><p>Methods to Determine Density</p><p>Two general approaches can be adopted to determine density. With the first approach, density is directly determined by counting plants within a defined sample unit, whereas the second approach is a plotless method based on measuring the distance or spacing between plants.</p><p>Both these methods are described in further detail in the following sections.</p><p> Counting Individual Plants Distance Methods </p><p>Counting Individual Plants</p><p>Counting plants within clearly defined sample units is one of the earliest techniques used to determine density rangeland vegetation. Counting individuals is usually adopted for herbaceous or small shrub species, because it is easy to count all individual plants in the small sample unit required to accommodate these compact and relatively common species. However, counting becomes more complicated as sample unit size expands to conform to the scale of larger plants, due to problems of defining quadrat or transect boundaries, bias arising from boundary decisions, and our ability to systematically inventory an extensive area.</p><p> Sample Units for Counting Individual Plants to Determine Density 95</p><p> Data Recording and Calculations when Counting Individual Plants to Determine Density </p><p>References and Further Reading</p><p>(Note: pdf files require Adobe Acrobat (free) to view)</p><p>Becker, D.A., and J.J. Crockett. 1973. Evaluation of sampling techniques on tallgrass prairie. Journal of Range Management 26:61-65. (pdf)</p><p>Bonham, C.D. 1989. Measurements for terrestrial vegetation. John Wiley & Sons, New York, NY. pp. 141-148.</p><p>Cook, C.W., and J. Stubbendieck. (eds). 1986. Range research: Basic problems and techniques. Society for Range Management, Denver, CO. p. 62.</p><p>Laycock, W.A. 1985. Density as a method for measuring rangeland vegetation. In W.C. Krueger (Chairman) Symposium on use of frequency and density for rangeland monitoring. Proceeding 38th Annual Meeting, Society for Range Management, Salt Lake City, UT, February 1985. pp. 92-93.</p><p>Mueller-Dombois, D., and H. Ellenburg. 1974. Aims and methods of vegetation ecology. John Wiley & Sons, New York. pp. 68-70.</p><p>Sample Units for Counting Individual Plants to Determine Density</p><p>Counting individual plants to determine density typically involve sampling procedures using a sample unit with defined boundaries (some type of quadrat), so that density can be expressed relative to a known area. In this way, the density values obtained from a small area are subsequently converted to a more conventional scale, such as plants/hectare or plants/acre.</p><p> Specific Guidelines on Sample Unit Size for Density Specific Guidelines on Sample Unit Shape for Density Specific Guidelines on Sample Size for Density </p><p>Data Recording and Calculations when Counting Individual Plants to Determine Density</p><p>Only a simple field sheet format is required when following the approach of counting individual plants to determine density that includes one column for species identification and additional columns to tally the number of individuals found in each quadrat (or transect). If nested quadrats are used, more than one column is needed to separately recorded each quadrat. Additional information on vegetation structure can be obtained by 96 recording seedlings apart from mature plants (especially if the inventory or monitoring program is conducted soon after germination and seedling survival is uncertain) or by separating a species into discrete height classes (most appropriate for woody species). Including information about sample unit size is important for future calculations.</p><p>Once field work is completed, data is summarized to estimate density for the sample. Part of the data summary involves adjusting values according to sample unit size, so that density is expressed on a standardized area (ie., m2, ft2, ha, acre).</p><p>Procedures followed to summarize the data depend upon definition of the sample unit.</p><p> Belt Transects to Determine Density Randomly Located Quadrats to Determine Density Quadrats Arranged as a Group of Subsamples to Determine Density </p><p>References and Further Reading</p><p>Bonham, C.D. 1989. Measurements for terrestrial vegetation. John Wiley & Sons, New York. pp. 145-146.</p><p>Bureau of Land Management. 1996. Sampling vegetation attributes. Interagency Technical Reference, BLM/RS/ST-96/002+1730. pp. 101-102.</p><p>Belt Transects to Determine Density</p><p>When the sampling layout is based on belt transects, density is calculated by dividing species tallies by belt transect area to estimate average density (number of individuals/transect area). In this case, each belt transect is regarded as a separate sample unit contributing to the values used in statistical analysis of density data.</p><p>References and Further Reading</p><p>Bonham, C.D. 1989. Measurements for terrestrial vegetation. John Wiley & Sons, New York. pp. 145-146.</p><p>Bureau of Land Management. 1996. Sampling vegetation attributes. Interagency Technical Reference, BLM/RS/ST-96/002+1730. pp. 101-102.</p><p>Lindsey, A.A. 1955. Testing the line-strip method against tallies in diverse forest types. Ecology 36:485-495.</p><p>Randomly Located Quadrats to Determine Density</p><p>When the sampling layout involves quadrats located under the principles of random sampling, each quadrat represents a sample unit and values describing density in each quadrat (number of individuals/quadrat area must be calculated for each quadrat) are used 97 in the statistical analysis of density data. Although this approach is straightforward, it can be an inefficient design to measure density because the sample usually has a large sample variance.</p><p>References and Further Reading</p><p>Bonham, C.D. 1989. Measurements for terrestrial vegetation. John Wiley & Sons, New York. pp. 145-146.</p><p>Bureau of Land Management. 1996. Sampling vegetation attributes. Interagency Technical Reference, BLM/RS/ST-96/002+1730. pp. 101-102.</p><p>Lindsey, A.A. 1955. Testing the line-strip method against tallies in diverse forest types. Ecology 36:485-495.</p><p>Quadrats Arranged as a Group of Subsamples to Determine Density</p><p>When the sampling layout for density involves quadrats arranged as a group of subsamples under the principles of systematic sampling, the group of quadrats (usually positioned to traverse an area) forms the sample unit. Density for each quadrat group must be calculated by obtaining a total group tally and then dividing by the number of quadrats, before dividing this value by the quadrat area to standardize the units of measurement. These sample means for each quadrat group comprise the values used in the statistical analysis of density data, and usually have a smaller sample variance compared to data from randomly located quadrats to determine density.</p><p>References and Further Reading</p><p>Bonham, C.D. 1989. Measurements for terrestrial vegetation. John Wiley & Sons, New York. pp. 145-146.</p><p>Bureau of Land Management. 1996. Sampling vegetation attributes. Interagency Technical Reference, BLM/RS/ST-96/002+1730. pp. 101-102.</p><p>Lindsey, A.A. 1955. Testing the line-strip method against tallies in diverse forest types. Ecology 36:485-495.</p><p>Distance Methods</p><p>Distance methods to determine density that involve plotless sampling regimes are based on determining the mean area associated with each plant by measuring the spacing between them. This approach builds on the pattern that when plants are crowded together in dense vegetation there is a small distance between individuals. 98</p><p>Distance methods are most commonly used in forests and shrublands (although some have been tried in bunchgrass), and work best when estimating the density of a single key species. These methods are usually adopted when a large area must be sampled, where it is difficult to follow the counting individual plants approach which requires that we delineate boundaries and methodically count all individuals. Inaccuracies caused by boundary decisions should also be prevented using a plotless method.</p><p>Nonetheless, distance methods can also be time consuming and arduous, and a second person may be needed to identify the correct neighboring plant and accurately measure the distance. This is especially true in dense vegetation, where it can be troublesome to see the key species or difficult to choose between several plants that are a similar distance from the sampling point. In contrast, excessive time may be spent scouting for individuals of an infrequently occurring species or in less dense vegetation.</p><p>However, the greatest shortcoming of many distance methods is the assumption that the sampled key species follow a random spatial pattern. This assumption rarely holds for herbaceous plants or shrubs (but is more likely to be tenable for some tree species) and nonrandom psatial patterns cause density to be underestimated. Some distance methods have been developed in an attempt to circumvent this assumption (Table 1).</p><p>Table 1. Plotless or distance methods developed to estimate density </p><p>Method Spatial Patterns Should be Reference Random</p><p>Closest Individual Method yes Cottam and Curtis (1956)</p><p>Nearest Neighbor Method yes Clark and Evans (1954)</p><p>Random Pairs Method yes Cottam and Curtis (1949)</p><p>Point Centered Quarter yes Cottam and Curtis Method (1956)</p><p>Wandering Quarter Method no Catana (1953)</p><p>Angle Order Method no Morisita (1957)</p><p>Corrected Point Distance no Bachelor (1971) Method 99</p><p>To provide an idea of the concepts and procedures involved with distance methods, three techniques are described in greater detail.</p><p> Closest Individual Method Point-Centered Quarter Method Wandering Quarter Method </p><p>References and Further Reading</p><p>(Note: pdf files require Adobe Acrobat (free) to view)</p><p>Bachelor, C.L. 1971. Estimating density from a sample of joint point and nearest- neighbor distances. Ecology 52:703-709.</p><p>Bonham, C.D. 1989. Measurements for terrestrial vegetation. John Wiley & Sons, New York. pp. 148-177.</p><p>Catana, A.J. 1953. The wandering quarter method of estimating population density. Ecology 44:349-360.</p><p>Clark, P.J., and F.C. Evans. 1954. Distance to nearest neighbor as a measure of spatial relationships in populations. Ecology 35:445-453.</p><p>Cook, C.W., and J. Stubbendieck. (eds). 1986. Range research: Basic problems and techniques. Society for Range Management, Denver, CO. pp. 62-63.</p><p>Cottam, G., and J.T. Curtis. 1949. A method for making rapid surveys of woodlands by means of pairs of randomly selected trees. Ecology 30:101-104.</p><p>Cottam, G., and J.T. Curtis. 1956. The use of distance measures in phytosociological sampling. Ecology 37:451-460.</p><p>Greig-Smith, P. 1983. Quantitative plant ecology. Blackwell Scientific Publications, Oxford.3rd ed. pp. 47-53.</p><p>Laycock, W.A. 1965. Adaptation of distance measurements for range sampling. Journal of Range Management 18:205-211. (pdf)</p><p>Laycock, W.A. 1985. Density as a Method for Measuring Rangeland Vegetation. In W.C. Krueger (Chairman) Symposium on use of frequency and sensity for rangeland monitoring. Proceeding 38th Annual Meeting, Society for Range Management, Salt Lake City, UT, February 1985. pp. 93-96.</p><p>Mueller-Dombois, D., and H. Ellenburg. 1974. Aims and methods of vegetation ecology. John Wiley & Sons, New York. pp. 68-70. 100</p><p>Morisita, M. 1957. A new method for the estimation of density by the spacing method applicable to non-randomly distributed populations. Physiology and Ecology 7:134-144. (In Japanese)</p><p>Closest Individual Method</p><p>The closest individual method was developed by Cottam and Curtis in the 1950's as a plotless technique to estimate density. It is a straightforward distance method to perform, but provides the most variable and least accurate result. Some other distance methods, such as nearest-neighbor and random-pairs methods, are adaptations that have been developed in an attempt to improve on this original simple method. All these distance methods assume that the key species follow a random spatial pattern, and therefore these methods are sensitive to bias when plants exhibit a more contagious or regular arrangement.</p><p>In the closest individual method, a set of points (usually positioned along a transect to traverse the area) is initially selected. At each point the closest plant is identified, and its distance (d) from the point is measured and recorded. At the conclusion of data collection, the sample mean of the distance ( ) between plants for the sample area is calculated.</p><p>Mean area (MA) can then be estimated as:</p><p>Density (plants/area) is then derived by calculating the inverse of MA (1/MA).</p><p>When following this procedure, each set of sample points is regarded as a separate sample unit, contributing to the values used in statistical analysis of density data.</p><p>References and Further Reading</p><p>(Note: pdf files require Adobe Acrobat (free) to view)</p><p>Bonham, C.D. 1989. Measurements for terrestrial vegetation. John Wiley & Sons, New York. pp. 149-152.</p><p>Cottam, G., and J.T. Curtis. 1956. The use of distance measures in phytosociological sampling. Ecology 37:451-460.</p><p>Laycock, W.A., and C.L. Batchelor. 1975. Comparison of distance-measurement techniques for sampling tussock grassland species in New Zealand. Journal of Range Management 28:235-239. (pdf) 101</p><p>McNeill, L., Kelly, R.D., and D.L. Barnes. 1977. The use of quadrat and plotless methods in the analysis of the tree and shrub component of woodland vegetation. Proceedings of the Grassland Society of Southern Africa 12:109-123.</p><p>Oldemeyer, J.L., and W.L. Regelin. 1980. Comparison of nine methods for estimating density of shrubs and saplings in Alaska. Journal of Wildlife Management 44:662-666.</p><p>Point-Centered Quarter Method</p><p>The point-centered quarter method was developed by Cottam and Curtis in the 1950's as a plotless technique to estimate density. It assumes that the key species follow a random spatial pattern, and is sensitive to bias when plants exhibit a more contagious arrangement. It also requires extra field effort and computational input than many of the other distance methods. Despite these disadvantages, the point-centered quarter method has been used in a wide variety of vegetation types, including some attempts to sample herbaceous vegetation.</p><p>In the point-centered quarter method, a set of points (usually positioned along a transect to traverse the area) is initially selected. The area around each point is divided into four 90° quadrants, and the plant closest to the point in each quadrant is identified. The distance between the central point and selected plant in each quadrant is measured, and then averaged across the four to represent the distance (d) at each sample point. At the conclusion of data collection, the average distance ( ) for all sample points is calculated.</p><p>Mean area (MA) can then be estimated as:</p><p>Density (plants/area) is then derived by calculating the inverse of MA.</p><p>When following this procedure, each set of points is regarded as a separate sample unit, contributing to the values used in statistical analysis of density data.</p><p>References and Further Reading</p><p>Beesom, S.L., and H. Haucke. 1975. A comparison of four distance sampling techniques in South Texas live oak mottes. Journal of Range Management 28:142-144.</p><p>Bonham, C.D. 1989. Measurements for terrestrial vegetation. John Wiley & Sons, New York. pp. 159-164.</p><p>Cottam, G., and J.T. Curtis. 1956. The use of distance measure in phytosociological sampling. Ecology 37:451-460. 102</p><p>Dix, R.L. 1961. An application of the point-centered quarter method to the sampling of grassland vegetation. Journal of Range Management 14:63-69.</p><p>Heyting, A. 1968. Discussion and development of the point-centered quarter method of sampling grassland vegetation. Journal of Range Management 21:370-380.</p><p>Penfold, W.T. 1963. A modification of the point-centered quarter method for grassland analysis. Ecology 44:175-176.</p><p>Risser, P.G., and P.H. Zedler. 1968. An evaluation of the grassland quarter method. Ecology 49:1006-1009.</p><p>Wandering Quarter Method</p><p>The wandering quarter method was developed by Catana in the 1950's as a plotless technique to estimate density. Adapted from the point-center quarter method, the wandering quarter method is based on non-parametric statistical procedures. Therefore, its validity is not restricted to species following random spatial patterns, and it is less sensitive to bias when plants exhibit contagious or regular arrangements.</p><p>In the wandering quarter procedure, a starting point is selected and then a compass is used to decide a direction to proceed from that point. From the first plant encountered, a 90° exclusion angle is determined forwards along the directional line (centered to form a 45° angle either side of the line). The nearest plant within this exclusion angle is identified and its distance is measured and recorded. This procedure is repeated after progressing to that plant, keeping the exclusion angle at the same alignment as at the starting point.</p><p>Density is calculated by nonparametric techniques which use sample mean and modal values to identify the scale of the contiguous spatial pattern and to determine distances within and between pattern clumps. Details of these mathematical techniques are described in Bonham (1989) or Catana (1953). The complicated computational requirements are a disadvantage of the wandering quarter method.</p><p>When following this procedure, the wandering transect is regarded as a separate sample unit contributing to the values used in statistical analysis of density data.</p><p>References and Further Reading</p><p>(Note: pdf files require Adobe Acrobat (free) to view)</p><p>Becker, D.A., and J.J. Crockett. 1973. Evaluation of sampling techniques on a tall-grass prairie. Journal of Range Management 26:61-65. (pdf)</p><p>Bonham, C.D. 1989. Measurements for terrestrial tegetation. John Wiley & Sons, New York. pp. 169-174. 103</p><p>Catana, A.J. 1953. The wandering quarter method of estimating population density. Ecology 44:349-360.</p><p>Lyon, J.L. 1968. An evaluation of density sampling methods in a shrub community. Journal of Range Management 21:16-20. (pdf)</p><p>Statistical Analysis of Density Data</p><p>Density measurements obtained by counting individual plants may follow a skewed or Poisson distribution, caused by a preponderance of low or zero values. The degree to which the data is skewed is influenced by sample unit size, because fewer individuals are typically found in small quadrats. Poisson distributions present a dilemma for conventional inferential statistical procedures, which involve the key assumption that the collected data fits a normal distribution. Careful consideration of sample unit shape and size (eg., adopting a design incorporating belt transects to determine density), helps to ensure that the collected data approaches a normal distribution. Alternatively, a design based on quadrats arranged as a group of subsamples to determine density is also more likely to generate data tending toward a normal distribution. Subjecting density values to a square-root transformation (which improves data symmetry) before statistical tests are conducted can also facilitate the assumption of normality.</p><p>Density measurements obtained by distance methods, such as the closest individual method, the point-center quarter method, or the wandering quarter method, are likely to approach a normal distribution because each value of the data set is derived from multiple readings. Providing this assumption is met, differences between sites or years can be determined by conventional inferential statistical procedures. If the confidence intervals for the two sample means being compared overlap, it is concluded that these values are not significantly different.</p><p>References and Further Reading</p><p>Greig-Smith, P. 1983. Quantitative plant ecology. Blackwell Scientific Publications, Oxford.3rd ed. pp. 33-36.</p><p>Frequency</p><p>Frequency is the vegetation attribute that describes the probability of finding a species within a particular area. The probability is based on the occurrence of that species in a series of sample units. For example, if a species has a frequency of 75%, we expect it to occur in three out of every four quadrats examined.</p><p>Frequency is expressed as a value between 0% and 100%, representing the proportion of quadrats where the particular species was found during sampling. For example, if we observed 200 quadrats and found the target species in 156 of those quadrats, frequency would be 104</p><p>Frequency is a simple vegetation attribute to measure because it only requires identification of the species in each quadrat, and does not require that individuals are distinguished, measured, or counted. Therefore, data collection is usually a more rapid procedure than for other vegetation attributes such as biomass, cover, or density, which involve counting or subjective quantification. This advantage is most apparent in rangeland vegetation characterized by a relatively low species richness, but diminishes in vegetation with complex species composition.</p><p>Frequency values are determined for individual species because an overall frequency for the entire vegetation cannot be obtained, in contrast to other attributes such as biomass, cover, or density. Likewise, it is not possible to obtain a meaningful expression of species composition from frequency data because the absolute abundance is not measured, only the presence of a species is measured.</p><p>Frequency can be a sensitive method to detect vegetation changes at a site. Its ease and speed of data collection means that frequency is suited to large areas, so it is sometimes adopted by State and Federal agencies for descriptive rangeland inventory or monitoring programs.</p><p>However, causes of the differences in frequency estimated between repeated sampling can be difficult to interpret because frequency is an index that integrates the density and spatial patterns of key species. For example, if frequency declines over time, it may be because individual plants are more sparsely scattered over the entire area, or because the range of the species distribution has constricted. In some cases, just knowing that there has been a change in the vegetation may meet the objectives of the monitoring program. In other situations, frequency can be used as a general monitoring procedure, that is followed by more detailed investigations to understand the cause of a change in frequency.</p><p>Special Considerations for Frequency Sampling</p><p>The following aspects of sample units are also important to consider when designing sampling protocols to determine frequency.</p><p> Nested Quadrats Rooted or Canopy Dimensions Species Groups Specific Guidelines on Sample Unit Size for Frequency Sources of Sampling Error </p><p>Measurement Techniques and Statistical Analysis</p><p> Methods to Determine Frequency 105</p><p> Statistical Analysis of Frequency Data </p><p>References and Further Reading</p><p>Bonham, C.D. 1989. Measurements for terrestrial vegetation. John Wiley & Son, New York, NY. pp. 90-96.</p><p>Cook, C.W., and J. Stubbendieck. (eds). 1986. Range research: Basic problems and techniques. Society for Range Management, Denver, CO. p. 63.</p><p>Daubenmire, R. 1968. Plant communities: A textbook on plant synecology. Harper & Row, New York, NY. pp. 53-57.</p><p>Despain, D.W., Ogden, P.R., and E.L. Smith. 1991. Plant frequency sampling for monitoring rangelands. In: G.B. Ruyle. (ed). Some methods for monitoring rangelands and other natural area vegetation. University of Arizona, College of Agriculture, Extension Report 9043. pp. 7-25.</p><p>Greig-Smith, P. 1983. Quantitative plant ecology. Blackwell Scientific Publications, Oxford. 3rd ed. p. 9.</p><p>Hironaka, M. 1985. Frequency approaches to monitor rangeland vegetation. In: W.C. Krueger. (chairman). Proceeding 38th Annual Meeting, Society for Range Management, Salt Lake City, UT, February 1985. pp. 84-86.</p><p>Mueller-Dombois, D., and H. Ellenberg. 1974. Aims and methods of vegetation ecology. John Wiley and Sons, New York, NY. pp. 70-76.</p><p>Sources of Sampling Error</p><p>One of the advantages of measuring frequency is that there are fewer opportunities for observer bias due to inadequate or inconsistent ground rules than when determining biomass, cover, or density.</p><p>Some error may arise from boundary decisions, due to the absolute 'present-or-absent' feature associated with frequency. This is particularly so for species occurring at lower frequencies, where an individual falling near the boundary is probably the only individual representing that species in the quadrat. With more abundant species, other individuals found within the quadrat negate the problem of deciding how to treat the individual at the edge of the quadrat.</p><p>The other source of error is associated with identification of the species within the quadrat. Overlooking certain species (particularly if small), or species misidentification, will compromise the accuracy of frequency data.</p><p>References and Further Reading: 106</p><p>Cook, C.W., and J. Stubbendieck. (eds). 1986. Range research: Basic problems and techniques. Society for Range Management, Denver, CO. p. 63.</p><p>Methods to Determine Frequency</p><p>There is only one general approach to determining frequency and it is based on identifying key species in each quadrat. It does not require that individuals are distinguished or counted. Therefore, variations of the basic method to determine frequency relate to properties of the sample unit and data manipulation.</p><p>Sample Units to Determine Frequency</p><p>Frequency data is usually collected using a sample unit with defined boundaries (some type of quadrat) because we need a clearly defined area within which to decide whether the species will be recorded as present.</p><p> Specific Guidelines on Sample Unit Size for Frequency Specific Guidelines on Sample Unit Shape for Frequency Specific Guidelines on Sample Size for Frequency </p><p>Data Recording and Calculations to Determine Frequency</p><p>References and Further Reading</p><p>Bonham, C.D. 1989. Measurements for terrestrial vegetation. John Wiley & Son, New York, NY. pp. 90-96.</p><p>Despain, D.W., Ogden, P.R., and E.L. Smith. 1991. Plant frequency sampling for monitoring rangelands. In: G.B. Ruyle. (ed). Some methods for monitoring rangelands and other natural area vegetation. University of Arizona, College of Agriculture, Extension Report 9043. pp. 7-25.</p><p>Mueller-Dombois, D., and H. Ellenburg. 1974. Aims and methods of vegetation ecology. John Wiley & Sons, New York, NY. pp. 72-76.</p><p>Data Recording and Calculations to Determine Frequency</p><p>The field sheet to record frequency usually includes a species list and a tally is made for every quadrat where the species is recorded. Only a single tally is made for each quadrat, which represents the presence of the species regardless of its abundance. If nested quadrats are used, more than one column is needed to separately recorded each quadrat.</p><p>When data is collected in this manner, it is not possible to combine several species groups after the field work has been completed. For example, in a monitoring program to assess Lehmann lovegrass (Eragrostis lehmanniana) spread within a pasture, we may decide to distinguish seedlings from established plants by providing each a separate category in the 107 species list on our field sheet, to find that seedlings occur at a 45% frequency and established plants at 30% frequency. However, this does not mean that frequency of the entire Lehmann lovegrass population is 75% (45% + 30%), because it is likely that seedlings and established plants both occurred in some quadrats, so that we may have double counted when only a single tally is permitted. This shortcoming can be overcome by recording species presence for each individual quadrat, but then the technique loses some of its speed and simplicity.</p><p>Once field work is completed, data is summarized to estimate frequency for each species in the sample. Procedures followed to summarize the data depend upon the definition of the sample unit, that was determined by the quadrat layout selected during the planning stages</p><p> Randomly Located Quadrats to Determine Frequency Quadrats Arranged As a Group of Subsamples to Determine Frequency </p><p>References and Further Reading</p><p>Despain, D.W., Ogden, P.R., and E.L. Smith. 1991. Plant Frequency Sampling for Monitoring Rangelands. In: G.B. Ruyle. (ed). Some Methods for Monitoring Rangelands and Other Natural Area Vegetation. University of Arizona, College of Agriculture, Extension Report 9043. pp. 15-16.</p><p>Randomly Located Quadrats to Determine Frequency</p><p>When the quadrat layout follows the principles of random sampling, frequency is calculated as the proportion of quadrats where the species was found. Each quadrat represents a sample unit, giving the sample data set a binomial distribution. Therefore, binomial procedures should be followed for the statistical analysis of frequency data collected in this manner.</p><p>References and Further Reading</p><p>Despain, D.W., Ogden, P.R., and E.L. Smith. 1991. Plant frequency sampling for monitoring rangelands. In: G.B. Ruyle. (ed). Some methods for monitoring rangelands and other natural area vegetation. University of Arizona, College of Agriculture, Extension Report 9043. pp. 15-16.</p><p>Whysong, G.L., and W.H. Miller. An evaluation of random and systematic plot placement for estimating frequency. Journal of Range Management 40:475-479.</p><p>Quadrats Arranged as a Group of Subsamples to Determine Frequency</p><p>Quadrats arranged as a group of subsamples under the principles of systematic sampling is the most common sampling design used for frequency. The quadrat-groups (usually 108 positioned along a transect to traverse an area) are taken to represent the sample unit. Frequency for each transect is determined from the quadrat subsample by calculating the proportion within that group where the species was found. Frequency values for each transect contribute to the sample data set that usually tends toward a normal distribution. Therefore, conventional inferential statistical procedures can be followed for the statistical analysis of frequency data collected in this manner.</p><p>References and Further Reading</p><p>Bonham, C.D. 1989. Measurements for terrestrial vegetation. John Wiley & Son, New York, NY. pp. 95-96.</p><p>Despain, D.W., Ogden, P.R., and E.L. Smith. 1991. Plant frequency sampling for monitoring rangelands. In: G.B. Ruyle. (ed). Some methods for monitoring rangelands and other natural area vegetation. University of Arizona, College of Agriculture, Extension Report 9043. pp. 15-16.</p><p>Whysong, G.L., and W.H. Miller. An evaluation of random and systematic plot placement for estimating frequency. Journal of Range Management 40:475-479.</p><p>Statistical Analysis of Frequency Data</p><p>Frequency data may be analyzed by several different techniques, depending upon how the sample units were located and how the data was collected.</p><p>Values collected from randomly located quadrats to determine frequency follow a binomial distribution. In this case, the comparison of sample means (evaluating significant differences between years or among sites, should be based on binomial statistics). In this situation, binomial confidence intervals are used to assess if two sample means are significantly different. The binomial confidence interval for a given frequency remains constant, according to sample size and the level of probability. Tables listing the width of confidence intervals have been developed for commonly used sample sizes (typically n=100 and n=200) and probability levels. If the confidence intervals (for the correct sample size and probability level) for the sample means being compared overlap, it is concluded that these values are not significantly different.</p><p>However, if the design is based on quadrats arranged as a group of subsamples to determine frequency, the data set of transect sample means follows a normal distribution. In this case, evaluating significant differences between years or sites can be based on conventional inferential statistics, whereby two sample means can be compared by considering the possibility that their respective confidence intervals overlap.</p><p>References and Further Reading:</p><p>(Note: pdf files require Adobe Acrobat (free) to view) 109</p><p>Despain, D.W., Ogden, P.R., and E.L. Smith. 1991. Plant frequency sampling for monitoring rangelands. In: G.B. Ruyle. (ed). Some methods for monitoring rangelands and other natural area vegetation. University of Arizona, College of Agriculture, Extension Report 9043. pp. 16-18.</p><p>Greig-Smith, P. 1983. Quantitative plant ecology. Blackwell Scientific Publications, Oxford. 3rd ed. pp. 36-41.</p><p>Hironaka, M. 1985. Frequency approaches to monitor rangeland vegetation. In: W.C. Krueger. (chairman). Proceeding 38th Annual Meeting, Society for Range Management, Salt Lake City, UT, February 1985. p. 85.</p><p>Whysong, G.L., and W.W. Brady. 1987. Frequency sampling and type II errors. Journal of Range Management 40:472-474. (pdf) </p><p>Whysong, G.L., and W.H. Miller. An evaluation of random and systematic plot placement for estimating frequency. Journal of Range Management 40:475-479. (pdf)</p><p>Species Composition</p><p>Species composition refers to the contribution of each plant species to the vegetation. Botanical composition is another term used to describe species composition. Species composition is generally expressed as a percent, so that all species components add up to 100%.</p><p>Species composition can be expressed on either an individual species basis, or by species groups that are defined according to the objectives of the inventory or monitoring program (eg., Aristida spp., perennial forage grasses, etc.).</p><p>Species composition is a commonly determined attribute in rangeland inventory and monitoring. It is regarded as an important indicator of ecological and management processes at a site.</p><p>Ecological indicators - species composition provides the essential description of the character of the vegetation at a site. Certain images are readily understood when major species are mentioned, eg., pinon (Pinus sp.) - juniper (Juniperus sp.) woodland, and other common species are also presumed to be present as one becomes familiar with the vegetation. These distinctions form the basis of rangeland mapping and the delineation of range site boundaries.</p><p>The relative contribution of a species also signifies its dominance in the vegetation and its ability to capture resources. Slightly different inferences of competitive ability are suggested if species composition is expressed on the basis of cover, density, or biomass measurements. 110</p><p>Management indicators - most objectives in rangeland management are directly concerned with the assessment or manipulation of species composition. For example, carrying capacity is influenced by the relative abundance of desirable forage species at a site. Wildlife habitat is also influenced by the relative contribution of various species that provide sources of shelter and food. Species composition is used to determine range condition and range trend, which are valuable tools to judge the impact of previous management and guide future decisions.</p><p>Special Considerations for Species Composition Sampling</p><p>Here is a critical issue to be considered when designing sampling protocols to determine species composition:</p><p> Species Groups </p><p>Methods to Determine Species Composition</p><p>Species composition is generally determined by sampling methods based on assessing the contribution of individual species or species groups in sample units. This section describes methods commonly used to determine species composition.</p><p> Dry Weight Rank Method Species Data from Other Attributes </p><p>References and Further Reading</p><p>Barbour, M.G., Burk, J.H., and W.D. Pitts. 1987. Terrestrial plant ecology. Benjamin Cummins Publishing Co, Menlo Park, CA. 2nd ed. pp. 191-193.</p><p>Bureau of Land Management. 1996. Sampling Vegetation Attributes. Interagency Technical Reference, BLM/RS/ST-96/002+1730. pp. 28-29.</p><p>Society for Range Management. 1989. A Glossary of Terms Used in Range Management. Society for Range Management, Denver, CO. 3rd ed. p.13.</p><p>Dry-Weight Rank Method</p><p>The dry-weight rank method is specifically designed to determine species composition by providing a measure of the relative contribution of various species to the total biomass (based on dry matter content) for a site.</p><p>Dry-weight rank results are expressed only as percentage values, and do not quantify the actual biomass for each species. For example, dry-weight rank sampling may indicate that black grama (Bouteloua eriopoda) makes up 11% of herbaceous biomass and burroweed (Isocoma tenuisectus) makes up another 43% of the total biomass, but we do not have the information to convert these values to quantify the actual biomass (kg/ha or 111 lb/acre) for either species. However, this problem can be circumvented by also determining the total biomass for the site, which is then proportioned to various species according to the percentage values derived from the dry-weight rank method. The comparative yield method complements procedures followed for the dry-weight rank method, and is usually employed concurrently to estimate total biomass.</p><p>To follow this technique, in each quadrat the observer identifies the first, second, and third most abundant species (on a dry weight basis), to which the ranks of 1, 2, and 3, are respectively assigned. When only 2 species occur in the quadrat, one of them should be given two ranks. For example, in a quadrat dominated by Lehmann lovegrass (Eragrostis lehmanianna) but including a few small croton (Croton spp.), the first and second rank may be assigned to Lehmann lovegrass, while croton is allocated the third rank. If only one species is found, it receives all three ranks for that quadrat.</p><p>At the end of sampling, ranks are tallied for each species, and weighted by a set of multipliers, usually 0.7 for Rank 1, 0.2 for Rank 2 and 0.1 for Rank 3. The weighted values of the three ranks are then added together for each species, and the result represents species composition. For many observers, the seemingly arbitrary multipliers are a source of bemusement, because it effectively assumes the highest ranked species within the quadrat contributes 70% of the biomass, the second contributes 20%, and the third ranked species 10%, while other less conspicuous species are disregarded. However, these multipliers have been tested across a wide variety of vegetation types in USA, Australia and Southern Africa, and found to provide reasonably accurate and precise results.</p><p>The main advantage of the dry-weight rank method is that little preliminary training is required, except to correctly identify species and to rank dry matter content of species with different phenological status or growth habits. Because the technique is based on ranking rather than quantification, data can be quickly collected in the field. In fact, rapid evaluation of each quadrat is the key to success for this technique, since a large sample is less sensitive to an occasional incorrect ranking. A sample size of 50-200 records is recommended for accurate results.</p><p>The main shortcoming of the dry-weight rank method rests on an underlying assumption that there is no relationship between a species occurring in the quadrat and its biomass. In reality, this assumption is often breached, because species that are characterized by large individuals tend to be found in high-biomass quadrats, whereas small species tend to be associated with low-biomass quadrats. The outcome is that species composition of regularly dispersed small plants, such as annual ground covers, will be overestimated. This problem can be solved by collecting dry-weight rank and biomass as paired data, so that the allocation of biomass to individual species can be computed on a quadrat by quadrat basis.</p><p>References and Further Reading</p><p>(Note: pdf files require Adobe Acrobat (free) to view) 112</p><p>Barnes, D.L., Odendall, J.J. and B.H. Beukes. 1982. Use of the dry-weight rank method of botanical analysis in the eastern Transvaal highveld. Proceedings of the Grassland Society of Southern Africa 17:79-82.</p><p>Bureau of Land Management. 1996. Sampling vegetation attributes. Interagency Technical Reference, BLM/RS/ST-96/002+1730. pp. 50-54.</p><p>Friedel, M.H., Chewings, V.H., and G.N. Bastin. 1988. The use of comparative yield and dry-weight rank techniques for monitoring arid rangelands. Journal of Range Management 41:430-434. (pdf)</p><p>Gillen, R.L., and E.L. Smith. 1986. Evaluation of the dry-weight-rank method for determining species composition in tall grass vegetation. Journal of Range Management 39:283-285. (pdf)</p><p>Jones, R.M., and J.N.G. Hargreaves. 1979. Improvements to the dry-weight-rank method for measuring botanical composition. Grass and Forage Science 34:181-189.</p><p>Mazaika, R., and P.R. Krausman. 1991. Use of dry-weight rank multipliers for desert vegetation. Journal of Range Management 44:409-411. (pdf)</p><p>Reese, G.A., Bayn, R.L., and N.E. West. 1980. Evaluation of double sampling estimators of subalpine herbage production. Journal of Range Management 33:300-306. (pdf)</p><p>Smith, E.L., and D.W. Despain. 1991. The dry-weight rank method of estimating plant species composition. In: G.B. Ruyle (ed). Some methods for monitoring rangelands and other natural area vegetation. University of Arizona, College of Agriculture, Extension Report 9043. pp. 27-47. t'Mannetje, L.H., and K.P. Haydock. 1963. The dry-weight-rank method for the botanical analysis of pasture. Journal of the British Grassland Society 18:286-275.</p><p>Species Data from Other Attributes</p><p>A description of species composition can be obtained when procedures are followed to determine other attributes, such as biomass, cover, or density, provided the data is collected at the species or species group level. Species composition is calculated by expressing the contribution of each species relative to the value determined for the entire site, ie.,</p><p>This calculation must be repeated for each species or species group. Obviously, species composition cannot be correctly evaluated if all components of the vegetation are not included in the data, such as when sampling objectives focus on a few key species. 113</p><p>Frequency is generally regarded as an unsuitable basis for the description of species composition, because simply recording the presence of a species does not indicate its absolute amount.</p><p>References and Further Reading</p><p>Barbour, M.G., Burk, J.H., and W.D. Pitts. 1987. Terrestrial plant ecology. Benjamin Cummins Publishing Co, Menlo Park, CA. 2nd ed. pp. 191-193.</p><p>Heady, H.F., and G.M. Van Dyne. 1965. Prediction of weight composition from point samples on clipped herbage. Journal of Range Management 18:144-148.</p><p>Laycock, W.A. 1985. Density as a Method for Measuring Rangeland Vegetation. In W.C. Krueger. (Chairman). Symposium on use of frequency and density for rangeland monitoring. Proceeding 38th Annual Meeting, Society for Range Management, Salt Lake City, UT, February 1985. p. 96.</p><p>Rangeland Evaluation</p><p>Introduction | General Principles | Sampling Concepts | Vegetation Attributes Rangeland Evaluation | Management Applications | Chapter Outline</p><p>Rangeland evaluation is the process of determining the status of natural rangeland resources. Historically, data describing vegetation attributes is collected by sampling in an inventory and monitoring program. From this information, the status of rangeland vegetation can be rated. Repeated measurements over time provides an indication of whether the vegetation is improving or declining compared to predetermined standards or goals.</p><p>Originally, these procedures were developed to offer guidelines for ranch management requirements, such as grazing management, reseeding or brush control. Today, however, public pressure demands more complex assessments based on appropriate ecological standards, in addition to land use demands. Furthermore, a good deal of intuition is required to reconcile interpretations of rangeland assessment with the practical management of livestock or wildlife. For example, a single evaluation provides little information concerning causes of current condition or of appropriate management responses, although the immediate impacts from grazing are often inferred. Even with repeated measurements over time, it is difficult to isolate grazing effects from climatic fluctuations, site variability and sampling error. Approaches that attempt to directly 114 address grazing issues, namely the evaluation of utilization patterns and of carrying capacity, are discussed under Management Implications.</p><p>The following sections discuss important concepts associated with conventional methods employed to evaluate the status of rangeland resources, as well as selected initiatives proposed to alleviate weaknesses in the current approaches. Variations adopted by different land management agencies (Natural Resources Conservation Service, Forest Service, and Bureau of Land Management) are not discussed in specific detail, although such inconsistencies represent a major problem when comparing rangelands held under different tenure.</p><p> Range Sites Range Condition Range Trend Recent Proposals for Evaluating the Status of Rangeland Resources </p><p>References and Further Reading:</p><p>Pearson, H.A., and J.W. Thomas. 1984. Adequacy of inventory data for management interpretations. In: National Research Council/National Academy of Sciences. Developing strategies for rangeland management. Westview Press. pp. 745-763.</p><p>Smith, E.L. 1984. Use of inventory and monitoring data for range management purposes. In: National Research Council/National Academy of Sciences. Developing strategies for rangeland management. Westview Press. pp. 809-842.</p><p>Task Group on Unity in Concepts and Terminology. 1995. New concepts for assessment of range condition. Journal of Range Management 48:271-282.</p><p>West, N.E., McDaniel, K., Smith, E.L., and S. Leonard. 1994. Monitoring and interpreting ecological integrity on arid and semi-arid lands of the western United States. Range Improvement Task Force, Las Cruces, NM. pp. 1-15.</p><p>Range Sites</p><p>Range sites are the principal units of rangeland classification that are based on categorizing vegetation according to site potential. Site potential is defined as the capacity of an area to support a distinct species composition and/or total biomass. Range sites act as the primary organizational element to obtain inventory and monitoring information during sampling. Therefore, the vegetation represented by a range site must be sufficiently uniform for the valid interpretation and extrapolation of data for management applications, yet incorporate the inherent variability expected in rangeland landscapes. 115</p><p>The range site concept was developed by the Soil Conservation Service (SCS, now Natural Resources Conservation Service) by adopting ideas previously introduced into forestry science. Other government agencies with land management responsibilities (ie., Bureau of Land Management, and Forest Service) use similar, but not always equivalent, concepts and terms to classify rangelands, such as ecological site, ecological type, or habitat type.</p><p>Most important, range site classification is centered on identifying differences in potential productivity, rather than simply categorizing the existing vegetation. A classification based on site potential is more meaningful to planning and management because it encourages the consideration of a wider range of options, rather than being restricted to existing conditions. However, deciding the nature of the potential plant community is also subject to the biases of personal judgement, particularly when the vegetation has been modified under a long tradition of land use.</p><p>These differences among range sites reflect the combined influence of environmental factors, including climate (total precipitation, seasonality of precipitation, temperature, frost free days, etc.), soils (soil surface texture, water holding capacity, etc.), topography, and fire. Therefore, common principles of ecological succession are incorporated into the range site concept, by assuming that only one distinctive plant community (the climax community), can evolve under a certain set of environmental conditions.</p><p>The importance of soil characteristics and topography on plant growth is well recognized, and these factors are usually included when naming range sites (e.g. Limestone Hills, Sandy Loam Upland, Clayey Bottom, etc.). Soil maps are often adopted as the foundation of range site maps, but extensive field reconnaissance, coupled with sampling of vegetation attributes such as biomass and species composition, must also be followed during the process of delineating range sites within an area. The same range site can occur at different locations across an extensive region, wherever the vegetation is considered to exhibit a similar potential for productivity and species composition.</p><p>Comprehensive descriptions have been developed for each range site, based on research, field surveys and professional observation. The description includes climate, topography, soils, potential vegetation, and management interpretations such as carrying capacity and resource value ratings. A major disparity between the various government agencies is that descriptions of the potential plant community are restricted to native species under Natural Resource Conservation Service (NRCS) procedures, whereas other agencies include naturalized introduced plants. This difference has considerable consequences, particularly when using the descriptions as benchmarks of site potential for range condition assessments.</p><p> USDA/NRCS Ecological Site Descriptions </p><p>References and Further Reading</p><p>(Note: pdf files require Adobe Acrobat (free) to view) 116</p><p>Anderson, E.W. 1983. Ecological site / range site / habitat type: A viewpoint. Rangelands 5:187-188. de Oliveira, J.G.B. 1979. Characterization of range sites. Ph.D. Dissertation, University of Arizona. pp. 1-105.</p><p>Driscoll, R.S. 1984. Classification for stratification, mapping, and data display for range inventories. In: National Research Council/National Academy of Sciences. Developing strategies for rangeland management. Westview Press. pp. 615-626.</p><p>Frost, W.E., and E.L. Smith. 1991. Biomass productivity and range condition on range sites in southern Arizona. Journal of Range Management 44:64-66. (pdf)</p><p>Leonard, S.G., Staidl, G.J., Gebhardt, K.A., and D.E. Prichard. 1992. Viewpoint: Range site/ecological site information requirements for classification of riverine riparian ecosystems. Journal of Range Management 45:431-435. (pdf)</p><p>Mueggler, W.F. 1984. Classification for stratification, mapping, and data display for range inventory: Comments and discussion. In: National Research Council/National Academy of Sciences. Developing strategies for rangeland management. Westview Press. pp. 627-630.</p><p>National Research Council. 1994. Rangeland health: New methods to classify, inventory and monitor rangelands. National Academy Press. pp. 66-75, 82-85.</p><p>Shiftlet, T.N. 1973. Range sites and soils in the United States. In: D.N. Hyder. (ed.) Arid shrublands. Proceedings of the Third Workshop of the United States/Australia Rangelands Panel. Society for Range Management, Denver, CO. pp. 26-33.</p><p>Society for Range Management. 1983. Guidelines and terminology for range inventories and monitoring. Report of the Range Inventory Standardization Committee. Society for Range Management, Denver, CO.</p><p>Soil Conservation Service. 1976. National range handbook. United States Department of Agriculture.</p><p>Task Group on Unity in Concepts and Terminology. 1995. New concepts for assessment of range condition. Journal of Range Management 48:271-282.</p><p>Weixelman, D., Zamudio, D., Zamudio, K., and R. Tausch. 1997. Classifying ecological types and evaluating site degradation. Journal of Range Management 50:315-321.</p><p>Range Condition</p><p>Range condition describes an evaluation of the current status of rangeland vegetation. Condition assessments provide the framework to register information obtained by range 117 inventories on the basic status of existing vegetation, and to gauge changes or range trend through monitoring. In addition, range condition is used as a guide to ensure sustainable land use, to determine carrying capacity and adjust stocking rates, to identify potential responses to range improvement programs such as brush control or reseeding, and to evaluate the best locations of fences and water facilities to improve utilization within a pasture.</p><p>In earlier days of rangeland management, range condition was a general term describing the status of resources at a site with particular reference to livestock grazing. Today, range condition usually carries a specific connotation, reflecting current status of the vegetation and soils occupying a site in comparison to the site potential expected if the climax vegetation was present. Therefore, an initial and critical step in evaluating range condition is to classify range sites to determine site potential.</p><p>The best-known procedure to assess range condition is the Quantitative Climax Method, used by the Soil Conservation Service (now Natural Resources Conservation Service) since the 1950s. This method compares species or species groups in the existing vegetation with that expected in the climax vegetation, to give a percentage reflecting the similarity between the two. A value close to 100% indicates that species composition of the existing vegetation closely reflects the composition of the climax vegetation, whereas lower values indicate a greater level of departure from perceived climax conditions. Although range condition is evaluated on a continuous scale from 0% to 100%, arbitrary classes are generally reported to illustrate range condition (Table 1).</p><p>Table 1. Range condition classes used in the Quantitative Climax Method </p><p>Range Condition Class Percent of Climax</p><p>Excellent 76 -100</p><p>Good 50 - 75</p><p>Fair 26 - 50</p><p>Poor 0 - 25</p><p>However, assumptions and interpretation of the quantitative climax method of range condition assessment presently attract widespread criticism and dispute for a number of reasons. a. Founded on inapplicable theories of plant succession - the current ecological approach using the climax vegetation as the benchmark to compare current vegetation status reflects a historical acceptance of the Clementsian theories of plant succession. Under these ideas, vegetation is expected to recover to an original climax composition following the reduction in grazing. However, these patterns are rarely apparent in many arid and 118 semiarid vegetation such as creosote (Larrea tridentata) flats, or former grasslands now infested by mesquite (Prosopis spp.), but instead better conform to the widely accepted 'state-and-transition' model that is the basis of current ecological theory. Other approaches to evaluate resource status, such as the site conservation rating, desired plant community, and rangeland health initiatives, are evolving as an attempt to accommodate the non-linear responses to disturbance observed in the vegetation on many rangeland areas. b. Sensitive to choice of benchmark - comparing current vegetation to potential productivity is both a strength and a weakness of range condition assessment. Site potential is highly meaningful to evaluation and management, because it provides the ultimate benchmark against which to base expectations regarding the type and amount of vegetation, natural erosion rates, comparisons of current site productivity, and alternative land uses. However, a realistic evaluation of range condition relies on selecting a benchmark that genuinely reflects potential site productivity. For example, sites of inherently low potential will always be categorized in 'poor' condition if inadvertently compared to a benchmark of intrinsically higher potential, even when there is no capacity to improve. c. Disregards nonnative species - in some agencies (particularly Natural Resources Conservation Service), range site potential excludes the presence of non-native species. This approach results in an automatic penalty at locations dominated by nonnative species, such as Lehmann lovegrass (Eragrostis lehmanianna) in the Southwest, crested wheatgrass (Agropyron cristatum) in the Intermountain Basin, or the Californian annual grasslands. In many cases, these non-native species contribute a significantly to the vegetation, offer forage and habitat for livestock and wildlife, and provide valuable soil protection. d. Excludes other important attributes influencing resource status - sustaining the productive potential at a site depends on maintaining the integrity of all resources, especially the soil. Current methods based comparing species composition are insensitive indicators of soil responses because many different compositions of vegetation could all provide an equivalent level of soil protection. Newer proposals to evaluate resource status, such as site conservation ratings or rangeland health initiatives, consider soil factors as range condition criteria in an attempt to correct this deficiency. e. Poorly reflects management objectives - range condition was originally developed to evaluate the impact of livestock grazing, based on the assumption that highest forage biomass was found on areas in near-climax species composition, and that excessive grazing pressure caused vegetation to undesirably depart from climax. In fact, some early proposals that suggested alternative approaches to evaluate range condition were strongly livestock oriented, and focused on relating current forage biomass to potential forage production for a site. However, the connection between climax vegetation and management goals are less explicit when considering nonnative species (discussed above) and multiple use objectives. For example, 'excellent' condition rangeland may not offer adequate habitat for certain wildlife species. Contemporary concepts, such as desired 119 plant communities or resource value ratings, attempt to evaluate management goals separately from the direct assessment of potential species composition for a site. f. Interpretation of condition labels - the terms excellent, good, fair, and poor are linked to emotive interpretations, often unrelated to the stability, diversity and productivity of the current vegetation. Several agencies have replaced these terms with low seral, mid seral, high seral, and potential natural to emphasize the ecological context of range condition, but interpretation is still open to criticism by those who believe that the climax vegetation must be the exclusive management goal. In many cases, a better understanding of the effect of current land use practices on rangeland resources is realized by highlighting range trends or similarities to the desired plant community, rather than solely being based on climax species composition. g. Unable to isolate the effects of grazing from other factors - vegetation and species composition vary between locations and over time in response to diversity of factors (including site variability, fire regimes, climatic conditions, etc.), yet range condition and range trend are commonly interpreted using the assumption that the impact of livestock grazing is primary.</p><p>References and Further Reading</p><p>(Note: pdf files require Adobe Acrobat (free) to view)</p><p>Dyksterhuis, E.J. 1949. Condition and management of rangeland based on quantitative ecology. Journal of Range Management 2:104-115. (pdf)</p><p>Ellison, L. 1949. The ecological basis for judging condition and trend on mountain rangeland. Journal of Forestry 47:785-795.</p><p>Friedel, M.H. 1991. Range condition assessment and the concept of thresholds: A viewpoint. Journal of Range Management 44:422-426. (pdf)</p><p>Frost, W.E., and E.L. Smith. 1991. Biomass productivity and range condition on range sites in southern Arizona. Journal of Range Management 44:64-67. (pdf)</p><p>Goebel, C.J., and C.W. Cook. 1960. Effect of range condition on plant vigor, production and nutritive value of forage. Journal of Range Management 13:307-313. (pdf)</p><p>Humphrey, R.R. 1949. Field comments on the range condition method of forage survey. Journal of Range Management 2:1-10. (pdf)</p><p>Joyce, L.A. 1993. The life cycle of the range condition concept. Journal of Range Management 46:132-138. (pdf)</p><p>Lauenroth, W.K., and W. A. Laycock. (eds). 1989. Secondary succession and the evaluation of range condition. Westview Press, Denver, CO. pp. 163. 120</p><p>Laycock, W.A. 1991. Stable states and thresholds of range condition on North American rangelands: A viewpoint. Journal of Range Management 44:427-433. (pdf)</p><p>Mosely, J.C., Bunting, S.C., and M. Hironaka. 1986. Determining range condition from frequency data in mountain meadows of central Idaho. Journal of Range Management 39:561-565. (pdf)</p><p>National Research Council. 1994. Rangeland health: New methods to classify, inventory and monitor rangelands. National Academy Press. pp. 75-81.</p><p>Pamo, E.T., Pieper, R.D., and R.F. Beck. 1991. Range condition analysis: comparison of two methods in southern New Mexico grasslands. Journal of Range Management 44:374- 378. (pdf)</p><p>Pieper, R.D., and R.F. Beck. 1990. Range condition from an ecological perspective: Modification to recognize multiple use objectives. Journal of Range Management 43:550-552. (pdf)</p><p>Scarnecchia, D.L. 1991. Review of "Secondary succession and the evaluation of range condition". Journal of Range Management 44:525. (pdf)</p><p>Soil Conservation Service. 1976. National range handbook. United States Department of Agriculture.</p><p>Task Group on Unity in Concepts and Terminology. 1995. New concepts for assessment of range condition. Journal of Range Management 48:271-282.</p><p>Tiedeman, J.A., Beck, R., and R.V. Ecret. 1991. Dependence of standing crop on range condition rating in New Mexico. Journal of Range Management 44:602-605. (pdf)</p><p>Tueller, PT., and W.H. Blackburn. 1974. Condition and trend of the big sagebush/needle- and-thread habitat type in Nevada. Journal of Range Management 27:36-40. (pdf)</p><p>Westoby, M., Walker, B., and I. Noy-Meir. 1989. Opportunistic management for rangelands not at equilibrium. Journal of Range Management 42:266-274. (pdf)</p><p>Wilson, A.D., and G.J. Tupper. 1982. Concepts and factors applicable to the measurement of range condition. Journal of Range Management 35:684-689. (pdf)</p><p>Workman, J.P. 1995. The value of increased forage from improved rangeland condition. Rangelands 17:46-48.</p><p>Range Trend</p><p>Range trend refers to the change in the status of resources at a site detected by monitoring and is usually expressed as improving, declining, or stable. It originally pertained to any 121 goal defined by management such as changing vegetation cover by adjusting stocking rates or grazing practices. The general association of range trend with data describing any vegetation attribute in a monitoring program is still theoretically valid, but today the term carries a more specific interpretation relating to the comparison of consecutive assessments of range condition in a monitoring program.</p><p>Therefore, weaknesses in methods to assess range condition are manifested in the evaluation of range trend. Most important, range trend is an ecological assessment relating current species composition to that perceived as the climax vegetation at a site, and without connotations to the goals of management. For example, improving range trends usually reflect more desirable conditions for livestock production and watershed stability, but could have undesirable consequences to the habitat of wildlife species that require a high proportion of forbs in their diet, (e.g., pronghorn). These interpretations are better indicated from other concepts such as the desired plant community or resource value ratings.</p><p>Because land resources must be monitored for an extended period to assess range trend, apparent trend is sometimes appraised from data describing site conditions collected at a single point in time. Apparent trend is determined by ranking soil and water criteria believed to reflect the probable changes in resource status, including the presence of unpalatable species, plant vigor, and soil surface conditions. Although a subjective appraisal relying on professional judgement, apparent trend provides land managers with useful information to guide short-term tactical decisions.</p><p>Site responses to climate and management must be distinguished to credibly interpret range trends and apparent trends. Repeated records based on quantitative comparisons over a long period provide the best indication of overall trends due to management, but such intensive monitoring strategies can be impractical in many situations. Even then, range trend assessments can be insensitive to detect early phases of improvement or deterioration, when masked by the yearly fluctuations in species composition caused by variable precipitation patterns. Although evaluation of range trends should be restricted to data collected at the same site to avoid the possible confounding by site variability, exclosures can act as valuable comparison areas to provide supplementary evidence describing the effect of current weather conditions.</p><p>References and Further Reading</p><p>(Note: pdf files require Adobe Acrobat (free) to view)</p><p>Barker, S., and K.M. Egen. 1993. Range trend monitoring in southern Arizona. Rangelands 15:166-167.</p><p>Bonham, C.D. 1989. Measurements for Terrestrial Vegetation. John Wiley & Sons, New York, NY. pp. 273-275. 122</p><p>Holechek, J.L., Pieper, R.D., and C.H. Herbel. 1995. Range Management Principles and Practices. Prentice Hall, Englewood Cliffs, NJ. 2nd ed. p. 166.</p><p>National Research Council. 1994. Rangeland Health: New Methods to Classify, Inventory and Monitor Rangelands. National Academy Press. pp. 81-82.</p><p>Ratliff, R.R. 1993. Viewpoint: trend assessment by similarity index. Journal of Range Management 46:139-141. (pdf)</p><p>Tueller, PT., and W.H. Blackburn. 1974. Condition and trend of the big sagebush/needle- and-thread habitat type in Nevada. Journal of Range Management 27:36-40. (pdf)</p><p>West, N.E., McDaniel, K., Smith, E.L., and S. Leonard. 1994. Monitoring and Interpreting Ecological Integrity on Arid and Semi-Arid Lands of the Western United States. Range Improvement Task Force, Las Cruces, NM. p. 11.</p><p>Wikeem, B.M., and M.D. Pitt. 1991. Grazing effects and range trend assessment on California bighorn sheep range. Journal of Range Management 44:466-470. (pdf)</p><p>Recent Proposals for Evaluating the Status of Rangeland Resources</p><p>Dissatisfaction with long-standing procedures to describe and interpret range condition and range trend has stimulated much discussion concerning alternative approaches to assess the status of rangeland resources. New approaches have been proposed to accommodate modern ecological theory and the demands of multiple use management. However, it is becoming increasingly apparent that a single procedure or index is unable to simultaneously satisfy the goals of ecological assessment and multiple use management. Therefore, most of the initiatives focus on either singularly assessing the status of rangeland resources, or presenting interpretations related to land use planning and management. Furthermore, many of these proposals have been developed or endorsed by national committees, representing the interests of many land management agencies and clients, which may encourage the adoption of common techniques. A selection of these topical initiatives are discussed in further detail.</p><p> Desired Plant Community Rangeland Health Resource Value Rating Site Conservation Rating </p><p>References and Further Reading</p><p>National Research Council. 1994. Rangeland health: New methods to classify, inventory and monitor rangelands. National Academy Press. pp. 180. 123</p><p>Task Group on Unity in Concepts and Terminology. 1995. New concepts for assessment of range condition. Journal of Range Management 48:271-282.</p><p>West, N.E., McDaniel, K., Smith, E.L., and S. Leonard. 1994. Monitoring and interpreting ecological integrity on arid and semi-arid lands of the western United States. Range Improvement Task Force, Las Cruces, NM. pp. 1-15.</p><p>Desired Plant Community</p><p>The desired plant community is selected as the one species composition that is most compatible with management objectives for a site. This decision depends on the relative value expected to be obtained from alternative land uses, as well as the feasibility of implementing actions required to change the present vegetation to a more desirable type. It is unlikely that the desired plant community would feature substandard levels of soil protection, as indicated by an unsatisfactory site conservation rating, because it is assumed that maintaining site potential should be an intrinsic goal of any management plan.</p><p>Concepts associated with the desired plant community recognizes that current methods to assess the status of rangeland resources do not always adequately reflect management goals, particularly those attempting to reconcile the conflicts of multiple uses such as livestock grazing, water quality, wildlife habitat, and recreation. The other strength of the desired plant community concept is it accepts that more than one species composition of the vegetation is possible at a site, which conforms to the widely accepted state-and- transition model at the center of current ecological theory. Various types of vegetation composition correspond to the various "states", and management actions required to alter species composition are the "transitions".</p><p>Therefore, the desired plant community represents the benchmark against which to compare existing vegetation and provides a system to evaluate the success of current practices in meeting management objectives. The desired plant community approach should be used as complementary information that specifically addresses management goals, rather than as a replacement to assessing ecological status, for example range condition or rangeland health evaluations.</p><p>References and Further Reading</p><p>Borman, M.M., and D.A. Pyke. 1994. Successional theory and the desired plant community approach. Rangelands 16:82-84.</p><p>National Research Council. 1994. Rangeland health: New methods to classify, inventory and monitor rangelands. National Academy Press. pp. 94-96.</p><p>Task Group on Unity in Concepts and Terminology. 1995. New concepts for assessment of range condition. Journal of Range Management 48:271-282. 124</p><p>Westoby, M., B. Walker, and I. Noy-Meir. 1989. Opportunistic management for rangelands not at equilibrium. Journal of Range Management 42:226-274.</p><p>Rangeland Health</p><p>In 1994, the National Research Council Committee on Rangeland Classification Systems published a report addressing current methods and possible improvements to assess the status of rangeland resources. The report recommended that assessments be based on rangeland health, which embodies the degree to which the integrity of soil and ecological processes within the ecosystem are sustained. Proper functioning of these processes is assumed to ensure that rangelands have the capacity to produce commodities and satisfy values on a sustainable basis.</p><p>The report acknowledged that the changing status of rangeland resources involves complex interactions that cannot be adequately determined by a single factor. Instead, rangeland health should be judged with several indicators that include:</p><p>1. Degree of soil stability and watershed function - assessed from soil surface characteristics such as depth of A-horizon, pedestalling, rills and gullies, sheet erosion, and sedimentation. 2. Nutrient cycling and energy flow - assessed by the distribution of plants, litter, roots, and age-classes. 3. Presence and functioning of recovery mechanisms - assessed by plant vigor, and the presence of seedlings and germination microsites. </p><p>From these measured indicators, rangeland health could be evaluated according to three categories:</p><p>1. Healthy - the capacity to satisfy values and produce commodities is being sustained, 2. At Risk - rangeland resources are indicating a reversible loss in capacity, or 3. Unhealthy - rangeland resources are indicating that a threshold has been crossed causing an irreversible loss in capacity, so that external inputs (eg., brush control, reseeding) are required to regain ecosystem integrity. </p><p>Equally important, the measured indicators of rangeland health might identify conditions where the ecosystem is particularly vulnerable to undesirable change, an early warning point, that marks the boundary between healthy and at risk rangelands, or a threshold that signals the beginning of irreversible changes associated with unhealthy rangelands. This approach corresponds to the widely accepted 'state-and-transition' model that is at the center of current ecological theory. It also has many similarities to threshold concepts included in the site conservation rating approach.</p><p>The National Research Council proposal is an innovative scheme to assess the ecological status of rangelands, but much of the detail required for implementation and interpretation remains undeveloped. Nonetheless, the report represents a major change in 125 the philosophy of range assessment, by emphasizing soil surface characteristics as a fundamental component of inventory and monitoring, and by accepting that the vegetation on many rangeland areas fails to follow the successional patterns expected under Clementsian ecological theory.</p><p>References and Further Reading</p><p>(Note: pdf files require Adobe Acrobat (free) to view)</p><p>Friedel, M.H. 1991. Range condition assessment and the concept of thresholds: A viewpoint. Journal of Range Management 44:422-426. (pdf)</p><p>Laycock, W.A. 1991. Stable states and thresholds of range condition on North American rangelands: A viewpoint. Journal of Range Management 44:427-433. (pdf)</p><p>National Research Council. 1994. Rangeland health: New methods to classify, inventory and monitor rangelands. National Academy Press. pp. 180.</p><p>Westoby, M., B. Walker, and I. Noy-Meir. 1989. Opportunistic management for rangelands not at equilibrium. Journal of Range Management 42:266-274. (pdf)</p><p>Resource Value Rating</p><p>Resource value ratings have been proposed as an approach to meet the demands of multiple use principles, by evaluating the suitability of the current vegetation for a specific use, such as livestock grazing, desert tortoise habitat, and recreation. Therefore, it is appropriate to assign several resource value ratings to each range site.</p><p>This approach contrasts with current rangeland assessment methods that are based on evaluating the ecological status without direct consideration of management goals. However, a resource value rating should be considered as complementary, rather than a replacement, to any assessment of ecological status, such as range condition or rangeland health.</p><p>References and Further Reading</p><p>(Note: pdf files require Adobe Acrobat (free) to view)</p><p>Hann, W.J. 1986. Evaluation of resource values in the northern region of the Forest Service. Rangelands 8:159-161.</p><p>National Research Council. 1994. Rangeland health: New methods to classify, inventory and monitor rangelands. National Academy Press. pp. 94-96. 126</p><p>Pieper, R.D., and R.F. Beck. 1990. Range condition from an ecological perspective: Modification to recognize multiple use objectives. Journal of Range Management 43:550-552. (pdf)</p><p>Society for Range Management. 1983. Guidelines and terminology for range inventories and monitoring. Report of the Range Inventory Standardization Committee. Society for Range Management, Denver, CO.</p><p>Task Group on Unity in Concepts and Terminology. 1995. New concepts for assessment of range condition. Journal of Range Management 48:271-282.</p><p>Wagner, B. 1986. Resource value ratings in relation to livestock forage values. Rangelands 8:155-157.</p><p>Wilson, A.D. 1989. The development of systems of assessing the condition of rangeland in Australia. In: W.K. Lauenroth and W.A. Laycock. (eds.) Secondary succession and the evaluation of rangeland condition. Westview Press, Boulder, CO. pp. 77-102.</p><p>Site Conservation Rating</p><p>Site conservation ratings assess the capacity of the current vegetation community to protect against the decline in productive potential of a range site. Evaluations are determined by comparing the soil protection provided by the existing vegetation with the site conservation threshold. The site conservation threshold is judged to be the minimum vegetation needed to prevent accelerated soil erosion. Therefore, separate site conservation thresholds must be determined for each site, based on criteria related to soil protection, such as plant cover, biomass or density. A satisfactory site conservation rating is assigned when the protective criteria exceed the minimum level indicated by the site conservation threshold, otherwise an unsatisfactory rating is specified.</p><p>Proposed by a Task Group created by the Society for Range Management, the concept aims to develop a scheme to evaluate rangeland status that integrates both soil and vegetation factors. By contrast, current methods of range condition assessment are based exclusively on vegetation characteristics, even though soil degradation has a greater influence on site potential. The site conservation rating concedes that various different types of vegetation are possible on a site without compromising the prevention of accelerated soil erosion.</p><p>References and Further Reading</p><p>Task Group on Unity in Concepts and Terminology. 1995. New concepts for assessment of range condition. Journal of Range Management 48:271-282.</p><p>Watters, S.E., Weltz, M.A., and E.L. Smith. 1996. Evaluation of a site conservation rating system in southeastern Arizona. Journal of Range Management 49:277-284. 127</p><p>Rangeland Inventory, Monitoring, and Evaluation: Management Applications </p><p>Introduction | General Principles | Sampling Concepts | Vegetation Attributes Rangeland Evaluation | Management Applications | Chapter Outline</p><p>Grazing by animals, both domestic livestock and wildlife, remains a primary land use on rangelands. Therefore, many rangeland inventory and monitoring programs must focus on obtaining information that can be interpreted to ensure productive and sustainable forage consumption within the management unit.</p><p> Carrying Capacity Utilization </p><p>References and Further Reading</p><p>Pearson, H.A., and J.W. Thomas. 1984. Adequacy of inventory data for management interpretations. In: National Research Council/National Academy of Sciences. Developing strategies for rangeland management. Westview Press. pp. 745-763.</p><p>Smith, E.L. 1984. Use of inventory and monitoring data for range management purposes. In: National Research Council/National Academy of Sciences. Developing strategies for rangeland management. pp. 809-842.</p><p>West, N.E., K. McDaniel, E.L. Smith, and S. Leonard. 1994. Monitoring and interpreting ecological integrity on arid and semi-arid lands of the western United States. Range Improvement Task Force, Las Cruces, NM. pp. 1-15.</p><p>Carrying Capacity</p><p>Carrying capacity describes the number of grazing animals a management unit is able to support without depleting rangeland vegetation or soil resources. While stocking rates may fluctuate in the short-term in response to fluctuations in the amount and quality of forage, carrying capacity reflects the average level of sustainable production over the long-term.</p><p>Determining carrying capacity is a fundamental component of rangeland evaluation, because it is an important management tool that connects forage supply and forage consumption. 128</p><p>Evaluating carrying capacity is an important application of rangeland inventory and monitoring programs, because it represents the key management tool to ensure sustainable use of natural resources. Although carrying capacity is a concept that typically relate to rangeland grazing for livestock production, wildlife considerations are equally relevant under the objectives of conservation and multiple use.</p><p>Inventories to determine carrying capacity form the basis of the stocking rate decisions that are particularly important for public land management agencies (eg., Forest Service, and Bureau of Land Management) seeking an objective platform for arbitrating grazing use. Information regarding the carrying capacity of a management unit is also useful for interpreting potential economic returns on ranch developments, such as developing watering points to improve livestock distribution or enhancing wildlife habitat. Moreover, an assessment of carrying capacity forms the basis of ranch value on the real estate market.</p><p>Monitoring trends in carrying capacity and stocking rates can often form the basis for changes in livestock management. For example, a decline in carrying capacity is usually interpreted as an indicator of poor resource management and a typical response would be to determine a carrying capacity that would improve range condition.</p><p>Although carrying capacity has important implications to management, shortcomings associated with its application should also be recognized. The primary complication in interpreting carrying capacity involves the incorporation of spatial and temporal variability. That is, both forage and animal intake are dynamic factors that vary according to site selection, time of sampling, species composition of the vegetation, utilization patterns, dietary preferences, livestock nutritive requirements, and resources available to the manager. Therefore, an evaluation of carrying capacity should be treated as a preliminary gauge to animal numbers for the management unit that will be revised in the light of monitoring information and immediate forage conditions.</p><p>Terminology Related to Carrying Capacity</p><p>These are some important terms and concepts related to carrying capacity.</p><p> Animal Units Animal Unit Equivalents Animal Unit Months Forage Stocking Rate </p><p>Measurement Techniques and Statistical Analysis</p><p> Methods to Determine Carrying Capacity </p><p>References and Further Reading 129</p><p>Holechek, J.L., R.D. Pieper, and C.H. Herbel. 1995. Range management principles and practices. Prentice Hall, Englewood Cliffs, NJ. 2nd ed. pp. 177-214.</p><p>Vallentine, J.F. 1990. Grazing management. Academic Press. San Diego, CA. pp. 294- 320.</p><p>Animal Units</p><p>Animal units (AU) are used as a basis to standardize and express stocking rates among different kinds and classes of livestock with similar dietary preferences. An AU is essentially a measure of forage demand by a theoretical animal unit that can then be converted according to the relative demands of different animal classes (based primarily on metabolic bodyweight) (Table 1).</p><p>Table 1. Suggested Animal Unit Conversion Factors for Various Classes of Cattle</p><p>Class of Cattle Relative Animal Unit</p><p>Mature Bull (>24 months) 1.5</p><p>Young Bull (18-24 months) 1.15</p><p>Cow and Calf Pair 1.35</p><p>Mature Cow, Non-Lactating 1.0</p><p>Pregnant Heifer, Non-Lactating (>18 months) 1.0</p><p>Yearlings (18-24 months) 0.9</p><p>Yearlings (15-18 months) 0.8</p><p>Yearlings (12-15 months) 0.7</p><p>Calves (weaning -12 months) 0.6</p><p>Calves (weaning at 8 months) 0.5</p><p>Source: Vallentine (1990), p 279.</p><p>However, inconsistencies persist among land management agencies and within published literature regarding approaches to quantify AU. For example, some interpretations loosely define an AU as the forage demanded by a mature cow, although this value could vary widely depending upon breed and reproductive status of the cow. Others advocate 130 that an AU represents the forage demanded by a standard livestock unit weighing 450 kg. Another straightforward approach is to simply estimate daily intake as 2%-3% of the animal's body weight.</p><p>References and Further Reading</p><p>(Note: pdf files require Adobe Acrobat (free) to view)</p><p>Cordova, F.J., J.D. Wallace, and R.D. Pieper. 1978. Forage intake by grazing livestock: A review. Journal of Range Management 31:430-438. (pdf)</p><p>Forero, L., L.R. Rittenhouse, and J.E. Mitchell. 1989. A cow-calf vs. yearling substitution ratio for shortgrass steppe. Journal of Range Management 42:343-345. (pdf)</p><p>Hinnant, R.T. 1994. What is an animal unit? A time to conform. Rangelands 16:33-35.</p><p>Holechek, J.L., R.D. Pieper, and C.H. Herbel. 1995. Range Management Principles and Practices. Prentice Hall, Englewood Cliffs, NJ. 2nd ed. pp. 268-272.</p><p>Perrier, G.K. 1996. Viewpoint: The animal unit as an ecological concept. Rangelands 18:30-31.</p><p>Scarnecchia, D.L. 1985. The animal-unit and animal-unit-equivalent concepts in range science. Journal of Range Management 38:346-349. (pdf)</p><p>Scarnecchia, D.L., and M.M. Kothmann. 1982. A dynamic approach to grazing management terminology. Journal of Range Management 35:262-264. (pdf)</p><p>Society for Range Management. 1989. A glossary of terms used in range management. Society for Range Management. Denver, CO. 3rd ed. p. 3.</p><p>Vallentine, J.F. 1990. Grazing Management. Academic Press. San Diego, CA. pp 276- 280.</p><p>Animal Unit Equivalents</p><p>Animal unit equivalents (AUE) have been developed to standardize grazing demand among different herbivore species. An AUE expresses the quantitative forage demand of a particular kind and class of animal relative to that of an animal unit, based primarily on metabolic bodyweight (Table 1).</p><p>Table 1. Suggested Factors Describing Animal Unit Equivalents for Various Herbivores </p><p>Type of Herbivore Animal Unit Equivalents 131</p><p>Mature Cow, Non-Lactating 1.0</p><p>Sheep, Mature, Non-Lactating 0.2</p><p>Ewe and Lamb Pair 0.3</p><p>Saddle Horse, Mature 1.25</p><p>Elk, Mature 0.65</p><p>Mule Deer, Mature 0.23</p><p>Whitetail Deer, Mature 0.17</p><p>Antelope, Mature 0.17</p><p>Source: Vallentine (1990), p 279.</p><p>In reality, however, AUE provides a poor basis to express carrying capacity in relation to different animal species because dietary preferences are ignored. For example, although AUE suggest that about 5 deer confer the same grazing demand as 1 cow (Table 1), their different diet (deer prefer browse and cattle prefer grass) means that this direct substitution is rarely observed. Furthermore, inventories conducted to determine biomass of available forage often focus on a particular land use, such as cattle grazing, and plants important to other herbivores may be excluded from the sampling protocol.</p><p>References and Further Reading</p><p>(Note: pdf files require Adobe Acrobat (free) to view)</p><p>Hansen, R.M., R.C. Clark, and W. Lawhorn. 1977. Foods of wild horses, deer, and cattle in the Douglas Mountain area, Colorado. Journal of Range Management 30:116-118.</p><p>Hobbs, N.T., and L.H. Carpenter. 1986. Viewpoint: Animal-unit equivalents should be weighted by dietary differences. Journal of Range Management 39: 470-471. (pdf)</p><p>Scarnecchia, D.L. 1985. The animal-unit and animal-unit-equivalent concepts in range science. Journal of Range Management 38:346-349. (pdf)</p><p>Scarnecchia, D.L. 1986. Viewpoint: Animal-unit equivalents cannot be meaningfully weighted by indices of dietary overlap. Journal of Range Management 39:471. (pdf)</p><p>Scarnecchia, D.L. 1990. Concepts of carrying capacity and substitution rations: a systems viewpoint. Journal of Range Management 43:553-555. (pdf) 132</p><p>Scarnecchia, D.L., and M.M. Kothmann. 1982. A dynamic approach to grazing management terminology. Journal of Range Management 35:262-264. (pdf)</p><p>Smith, A.D. 1965. Determining common use grazing capacities by application of the key species concept. Journal of Range Management 18:196-201. (pdf)</p><p>Society for Range Management. 1989. A Glossary of Terms Used in Range Management. Society for Range Management. Denver, CO. 3rd ed. p. 3.</p><p>Vallentine, J.F. 1990. Grazing Management. Academic Press. San Diego, CA. pp 278- 280.</p><p>Animal Unit Months</p><p>Animal unit months (AUM) is commonly adopted in rangeland situations as the unit used to express carrying capacity. AUM is a measure of forage supply within the management unit, based on the amount required to support an animal unit for 1 month. Animal unit days (AUD) and animal unit years (AUY) can also be adopted, but AUM better accommodates flexible or season-long grazing schedules.</p><p>Attempts have been made to convert AUM into a constant value that quantifies that amount of forage needed to support an animal unit for a month. For example, 1AU (~450 kg animal) x 2% intake/day x 30 days = 270 kg usable forage will support a 450 kg animal for 30 days. However, the actual weight assigned to an animal unit or for daily intake remains an issue of contention. As it is, AUM provides a useful rule-of-thumb to describe the carrying capacity within a management unit, and to serve as a common measure to compare sites or years.</p><p>References and Further Reading</p><p>(Note: pdf files require Adobe Acrobat (free) to view)</p><p>Scarnecchia, D.L. 1985. The animal-unit and animal-unit-equivalent concepts in range science. Journal of Range Management 38:346-349. (pdf)</p><p>Scarnecchia, D.L., and M.M. Kothmann. 1982. A dynamic approach to grazing management terminology. Journal of Range Management 35:262-264. (pdf)</p><p>Society for Range Management. 1989. A glossary of terms used in range management. Society for Range Management. Denver, CO. 3rd ed. p. 3.</p><p>Vallentine, J.F. 1990. Grazing management. Academic Press. San Diego, CA. pp 280- 284. 133</p><p>Forage</p><p>Forage is biomass that is potential food for livestock and wildlife, including herbaceous and woody sources. Only plants that are available and palatable to grazing animals are classified as forage. Therefore, a highly palatable Arizona cottontop (Digitaria californica) protected from grazing animals by a dense patch of prickly pear (Opuntia spp.) does not contribute to forage. Like browse, forage is also generally restricted to species or parts of plants deemed palatable by animals, but this issue is confused by the subjective nature of palatability and alternative feed sources.</p><p>References and Further Reading</p><p>(Note: pdf files require Adobe Acrobat (free) to view)</p><p>Holechek, J.L., Pieper, R.D., and C.H. Herbel. 1995. Range management principles and practices. Prentice Hall, Englewood Cliffs, NJ. 2nd ed. pp 135-136.</p><p>Schweitzer, S.H., Bryant, F.C., and D.B. Wester. 1993. Potential forage species for deer in the southern mixed prairie. Journal of Range Management 46:70-75. (pdf)</p><p>Society for Range Management. 1989. A glossary of terms used in range management. Society for Range Management. Denver, CO. 3rd ed. p 9.</p><p>Taylor, C.R., Brooks, T.D., and N.E. Garza. 1993. Effects of short duration and high- intensity low-frequency grazing systems on forage production and composition. Journal of Range Management 46:118-121. (pdf)</p><p>Stocking Rate</p><p>Stocking rate expresses the actual number of animals on a management unit throughout the time period of grazing. Therefore, stocking rates are the management interface that relate livestock consumption to forage supply. Stocking rate decisions have important ramifications on rangeland vegetation, livestock and economic responses. Although stocking rates may vary from year to year, average long-term stocking rates should closely reflect carrying capacity to ensure the optimal and sustainable grazing of range resources. For this reason, stocking rate considerations form the interpretative basis of many inventory or monitoring programs.</p><p>In year-long grazing situations, such as southwestern United States, stocking rates are generally expressed in animal units/acre/year (or /section/year in areas of low carrying capacity). In regions where grazing is restricted to part of the year, such as the Great Basin, stocking rate is generally expressed in animal unit months/acre.</p><p>References and Further Reading</p><p>(Note: pdf files require Adobe Acrobat (free) to view) 134</p><p>Holechek, J.L., R.D. Pieper, and C.H. Herbel. 1995. Range management principles and practices. Prentice Hall, Englewood Cliffs, NJ. 2nd ed. pp. 177-214.</p><p>Scarnecchia, D.L., and M.M. Kothmann. 1982. A dynamic approach to grazing management terminology. Journal of Range Management 35:262-264. (pdf)</p><p>Society for Range Management. 1989. A glossary of terms used in range management. Society for Range Management. Denver, CO. 3rd ed. p. 14.</p><p>Vallentine, J.F. 1990. Grazing management. Academic Press. San Diego, CA. pp. 321- 351.</p><p>Methods to Determine Carrying Capacity</p><p>Determining carrying capacity and setting stocking rates are some of the most challenging procedures connected with effective rangeland management. In fact, there are methods of sampling vegetation attributes and utilization that are both rapid and simple to use, and provide accurate and precise results. At best, the methods provide initial suggestions for stocking rates that should be adjusted in the light of subsequent monitoring of rangeland condition and rangeland trend.</p><p>Nonetheless, applications of carrying capacity are important tools in rangeland management, and are regularly expressed in inventory or monitoring objectives. Therefore, two approaches commonly adopted to determine carrying capacity have been selected for discussion in greater detail.</p><p> Matching Forage Supply and Animal Demand Range Site Guidelines </p><p>References and Further Reading</p><p>Jasmer, G.E., and J.L. Holechek. 1984. Determining grazing intensity on rangeland. Journal of Soil and Water Conservation 39:32-35.</p><p>McCall, T., R. Brown, and L Bender. 1997. Comparison of techniques for determining the nutritional carrying capacity for white-tailed deer. Journal of Range Management 50:33-37.</p><p>Smith, E.L. 1984. Use of inventory and monitoring data for range management purposes. In: National Research Council/National Academy of Sciences. Developing strategies for rangeland management. Westview Press. pp. 810-816, 822-824.</p><p>Vallentine, J.F. 1990. Grazing management. Academic Press. San Diego, CA. pp. 302- 308. 135</p><p>Matching Forage Supply and Animal Demand</p><p>A straightforward approach to determine the number of animals the management unit can support over a period of time is to divide the total forage biomass (ie., forage supply) by the total amount of forage consumed by a grazing animal during the grazing period (ie., forage demand). Calculations based on long term average forage production provide an appraisal of carrying capacity, whereas existing forage levels give an estimate of shorter term stocking rates.</p><p>When following this procedure, forage supply is derived from an estimate of biomass produced over the entire management unit. This estimate of biomass is the result of either sampling using a method to determine biomass, past experience, or professional judgement. Under sustainable management objectives, however, the actual amount of forage available to grazing animals must be considered to be less than total biomass. For example, an adjustment for allowable use must be incorporated into the calculation to ensure that some ungrazed residual biomass is maintained to protect soil and vegetation resources. Further adjustments should be made to discount the contribution of less accessible forage, such as areas that are distant from water (Table 1) or having steep, rugged terrain (Table 2).</p><p>Table 1. Suggested Reductions in Cattle Carrying Capacity with Distance from Water</p><p>Distance from Water Reduction in Carrying Capacity (km) (%)</p><p>0 - 1.6 None</p><p>1.6 - 3.2 50</p><p>>2 100</p><p>Source: Holechek et al. (1995), p 197.</p><p>Table 2. Suggested Reductions in Cattle Carrying Capacity for Different Slopes</p><p>Slope Reduction in Carrying Capacity (%) (%)</p><p>0 - 10 None</p><p>11 - 30 30</p><p>>21 - 60 60 136</p><p>>60 100</p><p>Source: Holechek et al. (1995), p 199.</p><p>Forage demand is usually a simple calculation that assumes that most rangeland ruminants have a daily consumption equivalent to about 2% of their body weight. However, attempts to allocate forage sources between various herbivores using animal unit equivalents create a more complicated situation, because plants rated as forage for one species may not be significant in the diet of another.</p><p>Obviously, this method to estimate carrying capacity involves many assumptions that are subject to a considerable amount of subjective interpretation. On the supply side of the equation, it is difficult to obtain accurate estimates of forage availability over extensive areas. Similarly, the willingness of animals to graze less accessible areas depends on the availability of alternative forage sources within the pasture, and the level of allowable use varies according to species composition of the vegetation, seasonal conditions and management objectives. On the demand side of the equation, animal intake is affected by breed, age, reproductive status and forage quality; and it is also difficult to quantify consumption by wildlife and invertebrate herbivores. Therefore, these calculations should be regarded only as initial guidelines for animal numbers in the management unit that will require further strategic adjustments depending upon specific management objectives, personal experience, immediate forage conditions, and inventory or monitoring that describes rangeland condition and rangeland trend assessments.</p><p> Example to Calculate Carrying Capacity by Matching Forage Supply and Animal Demand </p><p>References and Further Reading</p><p>(Note: pdf files require Adobe Acrobat (free) to view)</p><p>Cordova, F.J., J.D. Wallace, and R.D. Pieper. 1978. Forage intake by grazing livestock: A review. Journal of Range Management 31:430-438. (pdf)</p><p>Holechek, J.L. 1988. An approach for setting the stocking rate. Rangelands 10:10-14.</p><p>Holechek, J.L., and R.D. Pieper. 1992. Estimation of stocking rate on New Mexico rangeland. Journal of Soil and Water Conservation. 47:116-119.</p><p>Holechek, J.L., R.D. Pieper, and C.H. Herbel. 1995. Range management principles and practices. Prentice Hall, Englewood Cliffs, NJ. 2nd ed. pp. 197-204.</p><p>Martin, S.C. 1975. Stocking strategies and net cattle sales on semi-desert range. US Department of Agriculture, Forest Service Research Paper. RM-146. 137</p><p>Troxel, T.R., and L.D. White. 1989. Balancing forage demand with forage supply. Texas A & M University Extension Service Publication. B-1606.</p><p>Workman, J.P., and D.W. MacPherson. 1973. Calculating yearlong carrying capacity: An algebraic approach. Journal of Range Management 26:274-277. (pdf)</p><p>Example to Calculate Carrying Capacity by Matching Forage Supply and Animal Demand</p><p>Problem</p><p>A 4,000 ha ranch in the black grama (Bouteloua eriopoda) semi-desert ranges of south- eastern Arizona produces 300 kg/ha/year of key forage species in an average year. Because range condition across the ranch is currently fair, allowable use is set at 30% with the objective of improving range condition.</p><p>The terrain is generally flat, so grazing distribution is unrestricted by slope. However, inadequate drinking water development on the ranch results in 60% of the area being less than 1.6 km from water, another 25% is 1.6-3.2 km from water, and the remaining 15% of the ranch is rarely grazed because it is further than 3.2 km from water.</p><p>What is the grazing capacity of the ranch, assuming it is stocked with cows having an average weight of 400 kg, and the area is grazed for an entire year?</p><p>Solution a. Calculate total forage on the ranch that is accessible to livestock:</p><p>Total forage = forage production x area = 300 kg/ha x 4,000 ha = 1,200,000 kg.</p><p>However, not all of this forage is accessible to livestock because grazing access is restricted by distance from water:</p><p> 60% of the ranch is < 1.6 km from water (i.e., 100% accessible), 4,000 ha x 60% x 300 kg/ha x 100% accessible = 720,000 kg forage available in this zone. 25% of the ranch is 1.6 - 3.2 km from water (i.e., 50% accessible), 4,000 x 25% x 300 kg/ha x 50% = 150,000 kg forage available in this zone. 15% of the ranch is > 3.2 km from water (i.e., 100% unaccessible), 4,000 x 15% x 300 kg/ha x 0% accessible = 0 kg forage available in this zone. </p><p>Therefore, there is</p><p> 720,000 kg + 150,000 kg + 0 kg = 870,000 kg forage on the ranch which is accessible for grazing. 138 b. Calculate usable forage by adjusting for allowable use:</p><p> Usable forage = total accessible forage x allowable use = 870,000 kg x 30% = 261,000 kg forage available for use by livestock. c. Calculate the forage demanded by each cow for a year:</p><p> Forage demand = 2% x bodyweight x grazing days = 2% x 400 kg x 365 days = 2,920 kg forage is needed to feed each cow for one year. d. Calculate grazing capacity:</p><p> Grazing capacity = forage supply ÷ forage demand/cow = 261,000 kg ÷ 2,920 kg/cow = 89 cows/year. </p><p>Conclusion</p><p>The grazing capacity of the ranch is estimated to be 89 cows/year, while meeting the concurrent objective of improving range condition. Adjustments to this stocking rate may be required according to seasonal conditions and information from on-going monitoring.</p><p>References and Further Reading</p><p>Holechek, J.L., R.D. Pieper, and C.H. Herbel. 1995. Range management principles and practices. Prentice Hall, Englewood Cliffs, NJ. 2nd ed. pp. 197-204.</p><p>Example to Calculate Carrying Capacity by Matching Forage Supply and Animal Demand</p><p>Problem</p><p>A 4,000 ha ranch in the black grama (Bouteloua eriopoda) semi-desert ranges of south- eastern Arizona produces 300 kg/ha/year of key forage species in an average year. Because range condition across the ranch is currently fair, allowable use is set at 30% with the objective of improving range condition.</p><p>The terrain is generally flat, so grazing distribution is unrestricted by slope. However, inadequate drinking water development on the ranch results in 60% of the area being less than 1.6 km from water, another 25% is 1.6-3.2 km from water, and the remaining 15% of the ranch is rarely grazed because it is further than 3.2 km from water.</p><p>What is the grazing capacity of the ranch, assuming it is stocked with cows having an average weight of 400 kg, and the area is grazed for an entire year? 139</p><p>Solution a. Calculate total forage on the ranch that is accessible to livestock:</p><p>Total forage = forage production x area = 300 kg/ha x 4,000 ha = 1,200,000 kg.</p><p>However, not all of this forage is accessible to livestock because grazing access is restricted by distance from water:</p><p> 60% of the ranch is < 1.6 km from water (i.e., 100% accessible), 4,000 ha x 60% x 300 kg/ha x 100% accessible = 720,000 kg forage available in this zone. 25% of the ranch is 1.6 - 3.2 km from water (i.e., 50% accessible), 4,000 x 25% x 300 kg/ha x 50% = 150,000 kg forage available in this zone. 15% of the ranch is > 3.2 km from water (i.e., 100% unaccessible), 4,000 x 15% x 300 kg/ha x 0% accessible = 0 kg forage available in this zone. </p><p>Therefore, there is</p><p> 720,000 kg + 150,000 kg + 0 kg = 870,000 kg forage on the ranch which is accessible for grazing. b. Calculate usable forage by adjusting for allowable use:</p><p> Usable forage = total accessible forage x allowable use = 870,000 kg x 30% = 261,000 kg forage available for use by livestock. c. Calculate the forage demanded by each cow for a year:</p><p> Forage demand = 2% x bodyweight x grazing days = 2% x 400 kg x 365 days = 2,920 kg forage is needed to feed each cow for one year. d. Calculate grazing capacity:</p><p> Grazing capacity = forage supply ÷ forage demand/cow = 261,000 kg ÷ 2,920 kg/cow = 89 cows/year. </p><p>Conclusion</p><p>The grazing capacity of the ranch is estimated to be 89 cows/year, while meeting the concurrent objective of improving range condition. Adjustments to this stocking rate may be required according to seasonal conditions and information from on-going monitoring.</p><p>References and Further Reading 140</p><p>Holechek, J.L., R.D. Pieper, and C.H. Herbel. 1995. Range management principles and practices. Prentice Hall, Englewood Cliffs, NJ. 2nd ed. pp. 197-204.</p><p>Range Sites</p><p>Range sites are the principal units of rangeland classification that are based on categorizing vegetation according to site potential. Site potential is defined as the capacity of an area to support a distinct species composition and/or total biomass. Range sites act as the primary organizational element to obtain inventory and monitoring information during sampling. Therefore, the vegetation represented by a range site must be sufficiently uniform for the valid interpretation and extrapolation of data for management applications, yet incorporate the inherent variability expected in rangeland landscapes.</p><p>The range site concept was developed by the Soil Conservation Service (SCS, now Natural Resources Conservation Service) by adopting ideas previously introduced into forestry science. Other government agencies with land management responsibilities (ie., Bureau of Land Management, and Forest Service) use similar, but not always equivalent, concepts and terms to classify rangelands, such as ecological site, ecological type, or habitat type.</p><p>Most important, range site classification is centered on identifying differences in potential productivity, rather than simply categorizing the existing vegetation. A classification based on site potential is more meaningful to planning and management because it encourages the consideration of a wider range of options, rather than being restricted to existing conditions. However, deciding the nature of the potential plant community is also subject to the biases of personal judgement, particularly when the vegetation has been modified under a long tradition of land use.</p><p>These differences among range sites reflect the combined influence of environmental factors, including climate (total precipitation, seasonality of precipitation, temperature, frost free days, etc.), soils (soil surface texture, water holding capacity, etc.), topography, and fire. Therefore, common principles of ecological succession are incorporated into the range site concept, by assuming that only one distinctive plant community (the climax community), can evolve under a certain set of environmental conditions.</p><p>The importance of soil characteristics and topography on plant growth is well recognized, and these factors are usually included when naming range sites (e.g. Limestone Hills, Sandy Loam Upland, Clayey Bottom, etc.). Soil maps are often adopted as the foundation of range site maps, but extensive field reconnaissance, coupled with sampling of vegetation attributes such as biomass and species composition, must also be followed during the process of delineating range sites within an area. The same range site can occur at different locations across an extensive region, wherever the vegetation is considered to exhibit a similar potential for productivity and species composition. 141</p><p>Comprehensive descriptions have been developed for each range site, based on research, field surveys and professional observation. The description includes climate, topography, soils, potential vegetation, and management interpretations such as carrying capacity and resource value ratings. A major disparity between the various government agencies is that descriptions of the potential plant community are restricted to native species under Natural Resource Conservation Service (NRCS) procedures, whereas other agencies include naturalized introduced plants. This difference has considerable consequences, particularly when using the descriptions as benchmarks of site potential for range condition assessments.</p><p> USDA/NRCS Ecological Site Descriptions </p><p>References and Further Reading</p><p>(Note: pdf files require Adobe Acrobat (free) to view)</p><p>Anderson, E.W. 1983. Ecological site / range site / habitat type: A viewpoint. Rangelands 5:187-188. de Oliveira, J.G.B. 1979. Characterization of range sites. Ph.D. Dissertation, University of Arizona. pp. 1-105.</p><p>Driscoll, R.S. 1984. Classification for stratification, mapping, and data display for range inventories. In: National Research Council/National Academy of Sciences. Developing strategies for rangeland management. Westview Press. pp. 615-626.</p><p>Frost, W.E., and E.L. Smith. 1991. Biomass productivity and range condition on range sites in southern Arizona. Journal of Range Management 44:64-66. (pdf)</p><p>Leonard, S.G., Staidl, G.J., Gebhardt, K.A., and D.E. Prichard. 1992. Viewpoint: Range site/ecological site information requirements for classification of riverine riparian ecosystems. Journal of Range Management 45:431-435. (pdf)</p><p>Mueggler, W.F. 1984. Classification for stratification, mapping, and data display for range inventory: Comments and discussion. In: National Research Council/National Academy of Sciences. Developing strategies for rangeland management. Westview Press. pp. 627-630.</p><p>National Research Council. 1994. Rangeland health: New methods to classify, inventory and monitor rangelands. National Academy Press. pp. 66-75, 82-85.</p><p>Shiftlet, T.N. 1973. Range sites and soils in the United States. In: D.N. Hyder. (ed.) Arid shrublands. Proceedings of the Third Workshop of the United States/Australia Rangelands Panel. Society for Range Management, Denver, CO. pp. 26-33. 142</p><p>Society for Range Management. 1983. Guidelines and terminology for range inventories and monitoring. Report of the Range Inventory Standardization Committee. Society for Range Management, Denver, CO.</p><p>Soil Conservation Service. 1976. National range handbook. United States Department of Agriculture.</p><p>Task Group on Unity in Concepts and Terminology. 1995. New concepts for assessment of range condition. Journal of Range Management 48:271-282.</p><p>Weixelman, D., Zamudio, D., Zamudio, K., and R. Tausch. 1997. Classifying ecological types and evaluating site degradation. Journal of Range Management 50:315-321.</p><p>Utilization</p><p>Utilization refers to the amount of plant material that has been removed by animals during the grazing period. It can be based on either individual plants, key species, or an assessment of the entire management unit. However, most commonly the current year's production of accessible forage plants is considered when assessing utilization. Utilization is usually expressed as a percentage, that is calculated on a total biomass basis.</p><p>Utilization data are included in many rangeland inventory or monitoring programs as effective management tools to evaluate and modify the impact of grazing animals on vegetation resources. Livestock distribution patterns across the management unit can be classified into utilization zones, that become the basis for management decisions concerning the location of additional range improvements, such as water points, salt grounds, and fencelines. Utilization also acts as an useful index to compare the impact of different stocking strategies such as season of grazing</p><p>Comparisons of desired use and actual utilization levels during the grazing season are also valuable for evaluating stocking rates in the management unit, and adjusting livestock numbers. A common danger, however, lies in misinterpreting allowable use factors as specific management objectives, rather than as general guidelines for management decisions. Allowable use recommendations are too precise to be realistic goals for any given year, since actual percent utilization varies according to forage biomass. In addition, other factors such as the season and intensity of grazing, as well as temperature and soil moisture, have a much greater immediate effects on plant responses. Therefore, management should aim to achieve a level of actual use that, in the long term, will average to allowable use recommendations.</p><p>Some managers recommend basing utilization guidelines on residual biomass rather than forage removal. This approach better reflects the fundamental objectives of rangeland management, such as ensuring an appropriate leaf area index for initiating future growth, and protecting the surface condition and nutrient status of soils. Furthermore, residual biomass levels can be directly sampled by adopting a method to determine biomass, in 143 contrast to other methods to determine utilization which generally attempt to account for the proportion of vegetation already removed.</p><p>Terminology Related to Utilization</p><p>The following terms are used in discussing important concepts related to utilization.</p><p> Allowable Use Current Year's Growth Forage Key Area Key Species Residual Biomass </p><p>Measurement Techniques and Statistical Analysis</p><p> Methods to Determine Utilization </p><p>References and Further Reading</p><p>Cook, C.W., and J. Stubbendieck. (eds.). 1986. Range research: Basic problems and techniques.Society for Range Management, Denver, CO. pp. 120-132.</p><p>Frost, W.E., E.L. Smith, and P.R. Ogden. 1994. Utilization guidelines. Rangelands 16:256-259.</p><p>Society for Range Management. 1989. A glossary of terms used in range management. Society for Range Management. Denver, CO. 3rd ed. 15p.</p><p>Vallentine, J.F. 1990. Grazing management. Academic Press. San Diego, CA. pp. 294- 320.</p><p>Allowable Use</p><p>Allowable use, or proper use, refers to the level of utilization that promotes maximum grazing of forage sources without damaging the vegetation. Allowable use may refer to the entire management unit; or it may be based on selected key species.</p><p>Therefore, allowable use concepts aim to integrate the plant's physiological requirements into a management framework by considering their ability to maintain an acceptable level of vigor and reproductive capacity when defoliated. Great effort has been devoted to developing allowable use factors for key species, according to specific regions, range condition, seasons, and type of livestock. Some of these figures have been based on clipping studies, while others have relied on professional judgement. However, such applications of utilization measurements should only be accepted as general guidelines, 144 because too many other plant, animal, and environmental factors also influence the resilience of vegetation to grazing.</p><p>References and Further Reading</p><p>Frost, W.E., E.L. Smith, and P.R. Ogden. 1994. Utilization guidelines. Rangelands 16:256-259.</p><p>Hedrick, D.W. 1958. Proper utilization - problem in evaluating the physiological response of plants to grazing: a review. Journal of Range Management 11:34-43.</p><p>Sharp, L., K. Sanders, and N. Rimbey. 1994. Management decisions based on utilization - is it really management? Rangelands 16:38-40.</p><p>Current Year's Growth</p><p>Current year's growth is the above-ground biomass produced during the previous 12 months.</p><p>In environments with distinctive seasons, such as the monsoonal influence in the southwest, current year's growth is best approximated at the end of the summer growing season. For grasses, the biomass with a greyish hue is rejected as older than the current year's growth. For evergreen woody species, supple twigs and associated leaves are usually included as recent growth. Obviously, these judgements are subjective, and ground rules that present clear guidelines on the separation of old growth are required to maintain consistency among different observers.</p><p>In situations where climatic conditions promote year-long plant production, or where utilization by grazing animals has already removed some biomass, current year's growth is difficult to determine from data collected at a single time.</p><p>References and Further Reading</p><p>Bonham, C.D. 1989. Measurements for terrestrial vegetation. John Wiley & Sons, New York, NY. pp 241-243.</p><p>Forage</p><p>Forage is biomass that is potential food for livestock and wildlife, including herbaceous and woody sources. Only plants that are available and palatable to grazing animals are classified as forage. Therefore, a highly palatable Arizona cottontop (Digitaria californica) protected from grazing animals by a dense patch of prickly pear (Opuntia spp.) does not contribute to forage. Like browse, forage is also generally restricted to species or parts of plants deemed palatable by animals, but this issue is confused by the subjective nature of palatability and alternative feed sources. 145</p><p>References and Further Reading</p><p>(Note: pdf files require Adobe Acrobat (free) to view)</p><p>Holechek, J.L., Pieper, R.D., and C.H. Herbel. 1995. Range management principles and practices. Prentice Hall, Englewood Cliffs, NJ. 2nd ed. pp 135-136.</p><p>Schweitzer, S.H., Bryant, F.C., and D.B. Wester. 1993. Potential forage species for deer in the southern mixed prairie. Journal of Range Management 46:70-75. (pdf)</p><p>Society for Range Management. 1989. A glossary of terms used in range management. Society for Range Management. Denver, CO. 3rd ed. p 9.</p><p>Taylor, C.R., Brooks, T.D., and N.E. Garza. 1993. Effects of short duration and high- intensity low-frequency grazing systems on forage production and composition. Journal of Range Management 46:118-121. (pdf)</p><p>Key Areas</p><p>Key areas are sampling sites deliberately selected, based mainly on professional judgement, in rangeland inventory or monitoring programs to be representative of the entire management unit. Data from key areas are interpreted as an indicative guide of average responses to land use throughout the management unit.</p><p>As a type of selected sampling, the capacity of a key area to represent the entire area depends on the experience and skill of observers selecting the location. In general, key areas should represent common range sites of the management unit, and address the objectives of the monitoring program. For example, when monitoring utilization, key areas should provide important sources of forage, but not be too close nor too distant from water points since neither of those locations will be a typical indication of grazing patterns over the entire area.</p><p>References and Further Reading</p><p>Bureau of Land Management. 1996. Sampling vegetation attributes. Interagency Technical Reference, BLM/RS/ST-96/002+1730. pp 2-3.</p><p>Holechek, J.L., Pieper, R.D., and C.H. Herbel. 1995. Range management principles and practices. Prentice Hall, Englewood Cliffs, NJ. 2nd ed. pp 204-205.</p><p>Nevada Range Studies Task Group. 1984. Nevada rangeland monitoring handbook. Soil Conservation Service, Forest Service, Bureau of Land Management, University of Nevada Reno, Agricultural Research Service and Range Consultants. September 1984. pp 2-3, 9-10. 146</p><p>Smith, E.L., and G.B. Ruyle. 1991. Considerations when monitoring rangeland vegetation. G.B. Ruyle. (ed). Some methods for monitoring rangelands and other natural area vegetation. University of Arizona, College of Agriculture, Extension Report 9043. pp 2-3.</p><p>Key Species</p><p>Rangeland inventory or monitoring programs usually concentrate on sampling only a few important species or species groups that serve as indicators of status and/or trend for the entire vegetation. Key species should be selected to satisfy program objectives, such as rare species or important forage plants. This approach permits more efficient sampling and interpretation, because measuring all species is time consuming and often provides greater detail than required.</p><p>Because most inventory and monitoring programs have typically evaluated the impact of livestock grazing on rangelands, important forage plants have traditionally been selected as key species. Consequently, when the key species concept is commonly applied to determine utilization levels, selection of plants could vary according to dietary preferences associated with the type of animal using the rangeland and time of sampling. In this situation, the utilization of key species is extrapolated to judge the proper use of other species at the site. Therefore, key species should generally be abundant, productive and palatable components of the vegetation, and moderately sensitive to grazing.</p><p>However, key species can be selected to meet any management objective. For example, key species may be chosen in relation to their role in watershed protection, or as threatened or endangered species requiring special attention. Furthermore, if the management goal is to improve low range condition, key species may not be abundant in the existing vegetation, but should still possess a seed source and be known as an indicator of improving range condition.</p><p>References and Further Reading</p><p>Bureau of Land Management. 1996. Sampling vegetation attributes. Interagency Technical Reference, BLM/RS/ST-96/002+1730. pp 4-5.</p><p>Holechek, J.L., Pieper, R.D., and C.H. Herbel. 1995. Range management principles and practices. Prentice Hall, Englewood Cliffs, NJ. 2nd ed. pp 204-205.</p><p>Nevada Range Studies Task Group. 1984. Nevada rangeland monitoring handbook. Soil Conservation Service, Forest Service, Bureau of Land Management, University of Nevada Reno, Agricultural Research Service and Range Consultants. September 1984. pp 2-3, 10.</p><p>Roberts, B.R., and D.P.J. Opperman. 1974. Veld management recommendations, a reassessment of key species and proper use factors. Proceedings of the Grassland Society of Southern Africa 9:149-155. 147</p><p>Smith, A.D. 1965. Determining common use grazing capacities by application of the key species concept. Journal of Range Management 18:196-201.</p><p>Residual Biomass</p><p>Residual biomass refers to the weight of vegetation remaining after grazing is concluded. Residual biomass has important rangeland management ramifications because it acts as the source of future plant growth and as soil protection. For these reasons, residual biomass is a useful goal upon which to interpret utilization and base decisions concerning stocking rates. Residual biomass can be directly sampled using any of the methods to determine biomass.</p><p>References and Further Reading</p><p>(Note: pdf files require Adobe Acrobat (free) to view)</p><p>Bartolome, J.W., M.C. Stroud, and H.F. Heady. 1980. Influence of natural mulch on forage production on differing California annual range sites. Journal of Range Management 33:4-8. (pdf)</p><p>Bement, R.E. 1969. A stocking rate guide for beef production on blue-grama range. Journal of Range Management 22:83-86. (pdf)</p><p>Heady, H.F., and R.D. Child. 1994. Rangeland ecology and management. Westview Press, Boulder, CO. pp. 175-179.</p><p>McClaran, M.P., and D.N. Cole. 1993. Packstock in wilderness: Use, impacts, monitoring, and management. General Technical Report INT-301. Intermountain Research Station, United States Department of Agriculture, Forest Service. Ogden, UT. pp. 10-12.</p><p>Methods to Determine Utilization</p><p>Many methods have been developed to estimate utilization, but few have proven suitable for all situations. Depending on the objectives of the inventory or monitoring program, sampling may concentrate on describing utilization for several key species, or it may aim to describe grazing impacts on the entire forage resource.</p><p>The following sections describe several widely adopted approaches to estimate utilization.</p><p> Grazed and Ungrazed Comparisons Grazed Class Method Grazed Plant Method Height-Weight Method Utilization Zones 148</p><p>References and Further Reading</p><p>Bonham, C.D. 1989. Measurements for terrestrial vegetation. John Wiley & Sons, New York, NY. pp. 290-311.</p><p>Bureau of Land Management. 1996. Utilization studies and residual measurements. Interagency Technical Reference, BLM/RS/ST-96/004+1730. pp. 23-126.</p><p>Cook, C.W., and J. Stubbendieck. (eds.). 1986. Range research: Basic problems and techniques. Society for Range Management, Denver, CO. pp. 121-132.</p><p>Risser, P.G. 1984. Methods for inventory and monitoring of vegetation, litter, and soil surface condition. In: National Research Council/National Academy of Sciences. Developing strategies for rangeland management. Westview Press. pp. 667-673.</p><p>Grazed and Ungrazed Comparisons</p><p>Utilization can be estimated by comparing biomass from paired grazed and ungrazed sample units, with the difference representing the amount of forage consumed (or otherwise destroyed) during the grazing period. Data is collected at the end of the grazing period, by adopting an appropriate method to determine biomass.</p><p>Areas can be protected from grazing by either small or larger exclosures that restricted to key areas of the management unit. Small temporary cages are easily moved at the end of each grazing period to eliminate long term effects of cages. Larger permanent exclosures with special fences are useful to separate the effects of livestock and wildlife, but interpretations suffer from the confounding effects of extended periods of time with protection from grazing.</p><p>The advantage of the simple approach of grazed and ungrazed comparisons is that it overcomes a common shortcoming of many other methods to determine utilization, which depend on observer experience to imagine the biomass that has been removed. Several other factors, however, reduce the accuracy and precision of this method. First, different growth rates between grazed and ungrazed plants can compromise the legitimacy of protected plots as indicators of potential forage production. In addition, cages can modify the micro-environment to enhance plant growth within small caged plots. Finally, sample size is usually limited by the expenses associated with constructing and locating cages.</p><p>In another approach, utilization can be determined by comparing the biomass of forage before and after grazing, without installing structures to protect areas from livestock. However, this technique is only suited to short grazing periods where plant regrowth after grazing is insignificant. Because of regrowth after grazing, this method of estimating utilization will typically underestimate the actual level of utilization.</p><p>References and Further Reading 149</p><p>(Note: pdf files require Adobe Acrobat (free) to view)</p><p>Bonham, C.D. 1989. Measurements for terrestrial vegetation. John Wiley & Sons, New York, NY. pp. 309-310.</p><p>Bureau of Land Management. 1996. Utilization studies and residual measurements. Interagency Technical Reference, BLM/RS/ST-96/004+1730. pp. 70-75.</p><p>Cook, C.W., and L.A. Stoddart. 1953. The quandary of utilization and preference. Journal of Range Management 6:329-331. (pdf)</p><p>Frischnecht, N.C., P.W. Conrad, and P.E. Hansen. 1970. Improved folding utilization cages. Journal of Range Management 23:215-218.(pdf)</p><p>Heady, H.F. 1957. Effect of cages on yield and composition in the California annual type. Journal of Range Management 10:175-177.(pdf)</p><p>Hinnant, R.T., and M.M. Kothmann. 1982. Durable livestock exclosure for herbage production and utilization sampling. Journal of Range Management 35:127-128.(pdf)</p><p>Owensby, C.E. 1969. Effect of cages on herbage yield in true prairie vegetation. Journal of Range Management 22:131-132.(pdf)</p><p>Schulz, T.T., and W.C. Leininger. 1990. Differences in riparian vegetation structure between grazed areas and exclosures. Journal of Range Management 43:295-299.(pdf)</p><p>Grazed and Ungrazed Comparisons</p><p>Utilization can be estimated by comparing biomass from paired grazed and ungrazed sample units, with the difference representing the amount of forage consumed (or otherwise destroyed) during the grazing period. Data is collected at the end of the grazing period, by adopting an appropriate method to determine biomass.</p><p>Areas can be protected from grazing by either small or larger exclosures that restricted to key areas of the management unit. Small temporary cages are easily moved at the end of each grazing period to eliminate long term effects of cages. Larger permanent exclosures with special fences are useful to separate the effects of livestock and wildlife, but interpretations suffer from the confounding effects of extended periods of time with protection from grazing.</p><p>The advantage of the simple approach of grazed and ungrazed comparisons is that it overcomes a common shortcoming of many other methods to determine utilization, which depend on observer experience to imagine the biomass that has been removed. Several other factors, however, reduce the accuracy and precision of this method. First, different growth rates between grazed and ungrazed plants can compromise the legitimacy of protected plots as indicators of potential forage production. In addition, 150 cages can modify the micro-environment to enhance plant growth within small caged plots. Finally, sample size is usually limited by the expenses associated with constructing and locating cages.</p><p>In another approach, utilization can be determined by comparing the biomass of forage before and after grazing, without installing structures to protect areas from livestock. However, this technique is only suited to short grazing periods where plant regrowth after grazing is insignificant. Because of regrowth after grazing, this method of estimating utilization will typically underestimate the actual level of utilization.</p><p>References and Further Reading</p><p>(Note: pdf files require Adobe Acrobat (free) to view)</p><p>Bonham, C.D. 1989. Measurements for terrestrial vegetation. John Wiley & Sons, New York, NY. pp. 309-310.</p><p>Bureau of Land Management. 1996. Utilization studies and residual measurements. Interagency Technical Reference, BLM/RS/ST-96/004+1730. pp. 70-75.</p><p>Cook, C.W., and L.A. Stoddart. 1953. The quandary of utilization and preference. Journal of Range Management 6:329-331. (pdf)</p><p>Frischnecht, N.C., P.W. Conrad, and P.E. Hansen. 1970. Improved folding utilization cages. Journal of Range Management 23:215-218.(pdf)</p><p>Heady, H.F. 1957. Effect of cages on yield and composition in the California annual type. Journal of Range Management 10:175-177.(pdf)</p><p>Hinnant, R.T., and M.M. Kothmann. 1982. Durable livestock exclosure for herbage production and utilization sampling. Journal of Range Management 35:127-128.(pdf)</p><p>Owensby, C.E. 1969. Effect of cages on herbage yield in true prairie vegetation. Journal of Range Management 22:131-132.(pdf)</p><p>Schulz, T.T., and W.C. Leininger. 1990. Differences in riparian vegetation structure between grazed areas and exclosures. Journal of Range Management 43:295-299.(pdf)</p><p>Grazed Class Method</p><p>The grazed class method has been widely used by land management agencies to determine utilization of key species. This method uses data that is collected along pace transects where the plant closest to the toe at each sampling point is assigned to one of about 6 classes of utilization (e.g., 0%, 10%, 30%, 50%, 70%, 90%). Once the data is collected, utilization is estimated by multiplying the class value by the number of times it 151 was recorded, adding the results for each class, and calculating an average by dividing by the total number of observations in the transect.</p><p>The grazed class method recognizes the difficulties in assigning an exact utilization level to each plant. Ranking data into broad classes is also a relatively rapid procedure because observers are not required to spend as much time contemplating utilization levels to the closest percent! In fact, rapid evaluation is the key to success for this approach because a large sample is less sensitive to the occasional incorrect ranking. However, the method is still subject to personal bias, and sampling accuracy and precision will benefit if time is devoted to training observers. Photographic manuals that illustrate the typical appearance of plants at various levels of utilization have been developed to guide utilization rankings for key species of southern Arizona.</p><p>References and Further Reading</p><p>(Note: pdf files require Adobe Acrobat (free) to view)</p><p>Bureau of Land Management. 1996. Utilization studies and residual measurements. Interagency Technical Reference, BLM/RS/ST-96/004+1730. pp. 81-85.</p><p>Schmutz, E.M. 1978. Estimating range use with grazed-class photo guides. University of Arizona Cooperative Extension Service and Agricultural Station. Bulletin, A-73. pp. 1- 16.</p><p>Schmutz, E.M. 1983. Browsed-class method of estimating shrub utilization. Journal of Range Management 36:632-637. (pdf)</p><p>Schmutz, E.M., G.A. Holt, and C.C. Michaels. 1963. Grazed-class method of estimating forage utilization. Journal of Range Management 16:54-60. (pdf)</p><p>Sundt, P.L., and M.P. McClaran. 1993. The binomial use monitoring (BUM) method for desert shrubs. Rangelands 15:224-227.</p><p>Grazed Plant Method</p><p>The grazed plant method was developed by Roach in southern Arizona during the early 1950's to determine utilization. This method involves initially developing a regression relationship between the proportion of plants grazed (irrespective of the degree of grazing) and utilization levels, either by visiting sites with different grazing intensities or by repeatedly sampling the same site as grazing progresses through the year. The relationship can concentrate on key species or include all forage species, depending on the objectives of the inventory or monitoring program. Another method to determine utilization must be adopted to provide the data used to describe the relationship between the proportion of plants grazed and utilization levels. 152</p><p>Field sampling is a rapid procedure following the establishment of the regression relationship. Data is collected from pace transects, by classifying the plant closest to the toe at each sampling point as grazed or ungrazed. Once the proportion of grazed plants is calculated, utilization levels are estimated by referring to the regression relationship.</p><p>The effectiveness of the grazed plant method depends on grazing patterns of livestock within the management unit. In situations where plants are grazed only once, a value of R2>0.90 for the regression relationship indicates that the proportion of grazed plants is a strong prediction of utilization. However, further increases in utilization cannot be detected once all plants have been grazed, even if only lightly. The method is also insensitive when livestock tend to regraze the same plant, rather than uniformly grazing all vegetation. The regression relationship is also specific to the population where the data is collected, and caution must be taken when attempting to apply it to other years, sites, or different types of livestock.</p><p>References and Further Reading</p><p>Bonham, C.D. 1989. Measurements for terrestrial vegetation. John Wiley & Sons, New York, NY. pp. 298-299.</p><p>Cook, C.W., and J. Stubbendieck. (eds.). 1986. Range research: Basic problems and techniques. Society for Range Management, Denver, CO. pp. 127-129.</p><p>Gierisch, R.K. 1967. An adaption of the grazed plant method for estimating utilization of thurber fescue. Journal of Range Management 20:108-111.</p><p>Hurd, R.M., and N.A. Kissinger. 1953. Estimating utilization of Idaho fescue (Festuca idahoensis) on cattle range by percent of plants grazed. Rocky Mountain Forest and Range Experiment Station. Paper No. 12. pp. 1-5.</p><p>Roach, M.E. 1950. Estimating perennial grass utilization on semi-desert cattle range by percentage of ungrazed plants. Journal of Range Management 3:182-185.</p><p>Springfield, H.W. 1961. The grazed plant method for judging the utilization of crested wheatgrass. Journal of Forestry 59:666-670.</p><p>Height-Weight Method</p><p>Assessment of utilization by height-weight methods closely follows the approaches of indirect sampling to determine biomass. This technique involves initially establishing a regression relationship between the height and biomass of selected species. This relationship is developed by cutting ungrazed plants into equally spaced segments and weighing each portion. The regression relationship then allows utilization to be predicted by relating grazed plant height to the proportional reduction of plant biomass. 153</p><p>This approach is most successful to determine utilization of bunchgrasses, but has occasionally been applied to forbs and sedges. The regression relationship is rarely linear because a the greater proportion lies close to the ground. A major drawback of the method is that considerable effort must be devoted toward establishing the initial regression relationship and that relationship is likely to change between sites and from year to year. Furthermore, it is insensitive at low levels of utilization, particularly when livestock tend to take bites from only part of the plant rather than uniformly grazing the entire plant.</p><p>Similar indirect sampling methods based on dimension analysis are commonly used to determine utilization of browse species. Most approaches have focused on establishing relationships between the biomass of browse plants and their twig weight, twig length or twig diameter.</p><p>References and Further Reading</p><p>(Note: pdf files require Adobe Acrobat (free) to view)</p><p>Basile, J.V., and S.S. Hutchings. 1966. Twig diameter-length-weight relations of bittterbrush. Journal of Range Management 19:34-38. (pdf)</p><p>Bonham, C.D. 1989. Measurements for terrestrial vegetation. John Wiley & Sons, New York, NY. pp. 295-298, 302-305.</p><p>Bureau of Land Management. 1996. Utilization studies and residual measurements. Interagency Technical Reference, BLM/RS/ST-96/004+1730. pp. 89-102.</p><p>Cook, C.W., and J. Stubbendieck. (eds.). 1986. Range research: Basic problems and techniques. Society for Range Management, Denver, CO. pp. 123-125, 129.</p><p>Harshmann, E.P., and R. Forsman. 1978. Measuring fireweed utilization. Journal of Range Management 31:393-396. (pdf)</p><p>Heady, H.F. 1950. Studies on bluebunch wheatgrass in Montana and height-weight relationships of certain range grasses. Ecological Monographs 20:55-81.</p><p>Lommasson, T., and C. Jensen. 1943. Determining utilization of range grasses by height- weight tables. Journal of Forestry 41:589-593.</p><p>McDougald, N.K., and R.C. Platt. 1976. A method of determining utilization for wet mountain meadows on the summit allotment, Sequoia National Forest, California. Journal of Range Management 29:497-501. (pdf)</p><p>Mitchell, J.E., R. Elderkin, and J.K. Lewis. 1993. Seasonal height-weight dynamics of western wheatgrass. Journal of Range Management 46:147-151. (pdf) 154</p><p>Provenza, F.D.. and P.J. Urness. 1981. Diameter-length-weight relations for blackbrush ( Coleogyne ramosissima ) branches. Journal of Range Management 34:215-217. (pdf)</p><p>Utilization Zones</p><p>Creating maps that describe utilization zones within the management unit is an important step in many rangeland inventory or monitoring programs to provide valuable background information for further management. Utilization maps assist in the identification of key areas for sampling and management, the recognition of problems in livestock distribution, and in decisions concerning the location of additional range improvements.</p><p>Methods adopted to identify utilization zones should be rapid and accurate in order to ensure that a complete description is obtained for the entire management unit. Most techniques are based on a reconnaissance approach, whereby the whole area is visited to obtain a general impression of utilization levels and patterns. These patterns are directly transcribed onto baseline maps or aerial photographs while in the field. Utilization need not be determined with a high degree of precision because the primary aim of the map is to identify general patterns across the management unit. For example, rather than directly assessing utilization, classes could be defined from descriptions that compare the appearance of better forage species and less preferred species (Table 1). At a later stage, actual utilization levels might be estimated with more vigorous techniques.</p><p>Table 1. Suggested Classes for Mapping Utilization Zones </p><p>Use Description Degree of Class Use</p><p>None Very little or no use of key forage plants. 0-15%</p><p>Light Key forage plants lightly to moderately used. Practically no use 16-35% on low value forage plants.</p><p>Safe Key forage plants used to allowable intensity for the season the 36-65% season of grazing and sites involved. Some use of low value forage plants.</p><p>Heavy Key forage plants closely cropped. Low value forage plants 66-80% generally being grazed. Some trampling damage may be evident.</p><p>Severe Key forage plants destroyed. Low value forage plants carrying >80% the grazing load and are closely cropped. Trampling damage may be evident.</p><p>Source: Anderson and Currier (1978), p 88. 155</p><p>Mapping is easiest to conduct at the end of the grazing season, when utilization is greatest. In other cases, surveys earlier in the grazing season may indicate early grazing distribution problems. Patterns of wildlife use may also be described by mapping utilization before livestock are released into the management unit. Utilization zones are likely to remain relatively constant from year to year, so remapping is usually necessary only following changes in management such as stocking rates or season of grazing.</p><p>References and Further Reading</p><p>(Note: pdf files require Adobe Acrobat (free) to view)</p><p>Anderson, E.W., and W.F. Currier. 1973. Evaluating zones of utilization. Journal of Range Management 26:87-91. (pdf)</p><p>Bureau of Land Management. 1996. Utilization studies and residual measurements. Interagency Technical Reference, BLM/RS/ST-96/004+1730. pp. 23-24.</p><p>Nevada Range Studies Task Group. 1984. Nevada rangeland monitoring handbook. Soil Conservation Service, Forest Service, Bureau of Land Management, University of Nevada Reno, Agricultural Research Service and Range Consultants. September 1984. pp. 4-5,19-20.</p><p>Comparison Areas</p><p>Comparison areas, also known as reference areas or benchmark areas, are additional sampling locations established in rangeland inventory or monitoring programs to isolate the effects of land use from other natural events (especially weather factors) influencing vegetation responses. Therefore, comparison areas are usually chosen at localities with minimal management impacts, such as livestock exclosures or sites distant from water points. In other situations, fenceline contrasts reveal useful cues to separate the effects of land use from weather conditions.</p><p>Credible interpretations of land use impacts depend upon the selection of comparison areas that are representative of corresponding key areas or critical areas, partially realized by restricting sampling locations to the same range site. Problems of variability within a range site could be overcome by including multiple comparison areas, although this is seldom practical within the scope of time and budgetary constraints associated with most land evaluation programs.</p><p>References and Further Reading</p><p>Bureau of Land Management. 1996. Sampling vegetation attributes. Interagency Technical Reference, BLM/RS/ST-96/002+1730. p 6. 156</p><p>Smith, E.L., and G.B. Ruyle. 1991. Considerations when monitoring rangeland vegetation. G.B. Ruyle. (ed). Some methods for monitoring rangelands and other natural area vegetation. University of Arizona, College of Agriculture, Extension Report 9043. pp 2-3.</p><p>Wilson, A.D. 1984. Points of reference in the assessment of change in vegetation and land condition. Australian Rangeland Journal 6:69-74.</p><p>Critical Areas</p><p>Critical areas are sampling locations deliberately chosen in rangeland inventory or monitoring programs because of unique values or special concerns such as riparian zones, restricted habitats for threatened or endangered species, or range sites highly susceptible to erosion.</p><p>Unlike key areas, vegetation responses observed in critical areas are not interpreted to reflect management impacts over the entire management unit. Instead, critical areas are evaluated separately to ensure the fulfillment of specific goals that are associated with resource conservation.</p><p>References and Further Reading</p><p>Bureau of Land Management. 1996. Sampling vegetation attributes. Interagency Technical Reference, BLM/RS/ST-96/002+1730. pp 2-3.</p><p>Smith, E.L., and G.B. Ruyle. 1991. Considerations When Monitoring Rangeland Vegetation. G.B. Ruyle. (ed). Some methods for monitoring rangelands and other natural area vegetation. University of Arizona, College of Agriculture, Extension Report 9043. pp 2-3.</p><p>Selection of Attributes</p><p>Rangeland inventory and monitoring programs have usually focused on describing vegetation attributes, although soil characteristics are assuming greater importance in recent proposals for evaluating the status of rangeland resources. Vegetation attributes are generally regarded as easier to quantify compared to soil properties, which often rely on subjective assessments. Information on weather conditions, stocking rates, utilization patterns, wildlife, wildfires, and multiple use activities are useful to supplement measured vegetation responses, and to aid in interpreting the results. Photographs also provide a valuable visual record of the overall status of rangeland resources.</p><p>No single vegetation attribute is best suited to describe the existing status or to detect trends in rangeland vegetation. Instead, attributes should be selected in light of the objectives of each inventory or monitoring program, the type of vegetation, and the availability of skilled observers. Ideally, attributes should involve rapid and simple 157 sampling methods, to ensure that accurate and precise data can be collected within the budgetary and time constraints associated with many rangeland evaluation plans.</p><p>Vegetation attributes commonly measured in rangeland inventory and monitoring programs include:</p><p> Biomass - is the primary objective of inventories conducted to determine carrying capacity, utilization patterns, or range condition. Despite its importance in management applications, few techniques are available to rapidly obtain accurate and precise biomass data for large areas, so that other attributes such as cover are usually recommended for large scale monitoring programs. It is also difficult to isolate fluctuations in biomass created by weather conditions and immediate grazing history from changes induced by active management. Cover - is widely adopted for both inventory and monitoring purposes because it is an attribute that provides rapid and repeatable data that is relevant to many practical interpretations regarding land use (eg., forage availability, wildlife habitat) and resource status (eg., erosion potential). Basal cover is generally considered a more stable measure than canopy cover to identify herbaceous vegetation change, since it is less influenced by weather fluctuations, time of year, and immediate grazing history. Density - may provide useful inventory information for large perennial species, particularly trees and shrubs. However, density is rarely measured in monitoring situations, because it can be very time consuming, and there are errors associated with the identification of individual plants. Frequency - is regularly used in monitoring programs, because it is a rapid technique that provides precise results. Although a sensitive index of changes in a species over time, additional data must be collected to reveal which other attribute caused the change. Frequency has less relevance in inventory applications, because it does not provide an absolute measure of species abundance. Species Composition - is commonly assessed to describe the character of vegetation during detailed inventory programs. However, accurate determination of species composition can be tedious, because all species must be sampled. Therefore, in long-term or broadscale monitoring situations, it can be more efficient to concentrate on a few key species or species groups, chosen to meet the objectives of the program. </p><p>In addition to deciding which vegetation attributes to measure, the planning of inventory or monitoring programs must also select which plant species to include in the sampling protocol.</p><p> Key Species Species Groups </p><p>References and Further Reading 158</p><p>Bonham, C.D. 1989. Measurements for terrestrial vegetation. John Wiley & Sons, New York, NY. pp 268-283.</p><p>Bureau of Land Management. 1996. Sampling vegetation attributes. Interagency Technical Reference, BLM/RS/ST-96/002+1730. pp 23-29.</p><p>Holechek, J.L., Pieper, R.D., and C.H. Herbel. 1995. Range management principles and practices. Prentice Hall, Englewood Cliffs, NJ. 2nd ed. pp 162-165.</p><p>McClaran, M.P., and D.N. Cole. 1993. Packstock in wilderness: Use, impacts, monitoring, and management. General Technical Report INT-301. Intermountain Research Station, United States Department of Agriculture, Forest Service. Ogden, UT. pp 11-13.</p><p>Pieper, R.D. 1984. A critique of "Methods for Inventory and Monitoring of Vegetation, Litter, and Soil Surface Condition". In: National Research Council/National Academy of Sciences. Developing strategies for rangeland management. Westview Press. pp 691- 701.</p><p>Risser, P.G. 1984. Methods for inventory and monitoring of vegetation, litter, and soil surface condition. In: National Research Council/National Academy of Sciences. Developing strategies for rangeland management. Westview Press. pp 647-690.</p><p>Smith, E.L., and G.B. Ruyle. 1991. Considerations when monitoring rangeland vegetation. G.B. Ruyle. (ed). Some methods for monitoring rangelands and other natural area vegetation. University of Arizona, College of Agriculture, Extension Report 9043. pp 1-2.</p><p>Time of Sampling</p><p>Season to season fluctuations in vegetation attributes represent the key consideration when deciding what time of year to conduct rangeland inventory or monitoring programs. To prevent seasonal differences from confounding interpretations, comparisons between inventory data from various sites or monitoring data from a single site over a number of years, should be confined to the same season so that key species are at a similar level of maturity. Also, it is possible to select vegetation attributes that are relatively insensitive to seasonal fluctuations, such as basal cover, to minimize the effects of sampling during different seasons.</p><p>The best time of the year to sample depends upon the objectives of the program and the selection of attributes to be measured. For example, regular monitoring to evaluate stocking rates by determining utilization levels are best conducted at the end of the grazing season. If species composition is identified as a monitored attribute, more accurate data collection is possible by coinciding field work with the period when plants are flowering and identifiable. 159</p><p>Other secondary factors may also control the most practical time of sampling. For example, sampling opportunities may be restricted by boggy soils limiting the accessibility to high elevation sites during spring, the availability of skilled labor, or conflicting workplace commitments.</p><p>References and Further Reading</p><p>Bureau of Land Management. 1996. Sampling vegetation attributes. Interagency Technical Reference, BLM/RS/ST-96/002+1730. pp 1-29.</p><p>McClaran, M.P., and D.N. Cole. 1993. Packstock in wilderness: Use, impacts, monitoring, and management. General Technical Report INT-301. Intermountain Research Station, United States Department of Agriculture, Forest Service. Ogden, UT. p 13.</p><p>Smith, E.L., and G.B. Ruyle. 1991. Considerations when monitoring rangeland vegetation. G.B. Ruyle. (ed). Some methods for monitoring rangelands and other natural area vegetation. University of Arizona, College of Agriculture, Extension Report 9043. p 3.</p><p>How Often to Sample</p><p>Data need only be collected on a single occasion for an inventory program, to document rangeland resources and to evaluate their current status. In contrast, monitoring must be conducted on a recurrent basis to detect changes in vegetation attributes. Although it may appear simpler to identify and interpret the causes of vegetation change under an annual sampling regime, such a protocol is rarely undertaken except when monitoring utilization. For example, vegetation changes large enough to assume practical significance often take more than one year to become evident, particularly after allowing for variability arising from weather, and sampling error factors. Consequently, the optimum interval between sampling depends on the longevity of key species. For example, long-lived perennial shrubs such as mesquite (Prosopis spp.) need not be monitored as frequently as populations of shorter-lived annuals or biennials such as Rothrock grama (Bouteloua rothrockii). In addition, frequent sampling is usually limited by time and budgetary constraints.</p><p>Therefore, with the exception of biomass data to evaluate utilization patterns or residual biomass levels, it is not unusual to return to sample sites only once every 3 to 5 years in a long-term monitoring program. More frequent monitoring may be desirable for newly developed schedules, to confirm that the selection of attributes, time of sampling, sampling methods and site selection are suited to the objectives of the program. In other situations, it may be plausible to a) measure a different subset of sites each year so that all sites are sampled at the end of 3 to 5 years, most years, b) measure all sites when changes are noted at a subset of sites, c) when altering stocking rates, or d) during extraordinary seasonal conditions. Photographs may also be considered as a quick and easy alternative 160 to provide additional information for sites in years when sampling of vegetation attributes is not undertaken.</p><p>References and Further Reading</p><p>McClaran, M.P., and D.N. Cole. 1993. Packstock in wilderness: Use, impacts, monitoring, and management. General Technical Report INT-301. Intermountain Research Station, United States Department of Agriculture, Forest Service. Ogden, UT. p 14.</p><p>Smith, E.L., and G.B. Ruyle. 1991. Considerations when monitoring rangeland vegetation. G.B. Ruyle. (ed). Some methods for monitoring rangelands and other natural area vegetation. University of Arizona, College of Agriculture, Extension Report 9043. p 3.</p>
Details
-
File Typepdf
-
Upload Time-
-
Content LanguagesEnglish
-
Upload UserAnonymous/Not logged-in
-
File Pages160 Page
-
File Size-