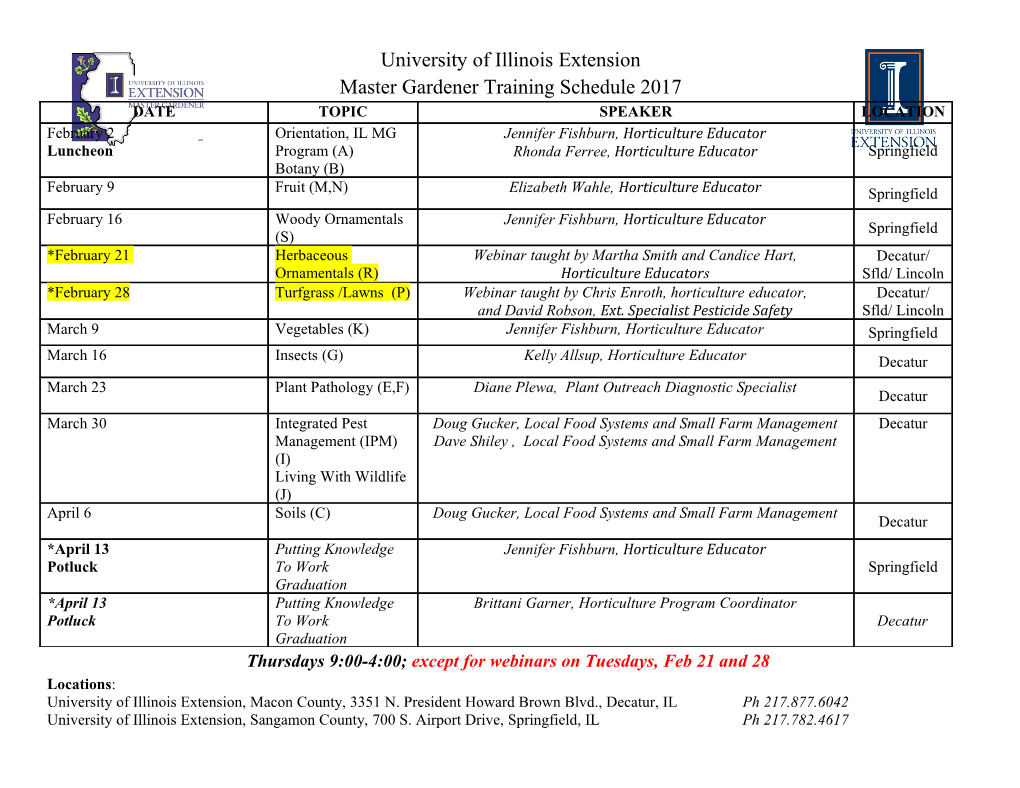
<p>Massively parallel aligned nanofibers-based</p><p> nanogenerator deposited via in situ,</p><p> oriented poled near-field electrospinning</p><p>Yiin-Kuen Fuha,*, Shao-Yu Chena, Jia-Cheng Yeb a Department of Mechanical Engineering, National Central University, Jhongli City, Taoyuan County, Taiwan, R.O.C b Graduate Institute of Energy Engineering, National Central University, Jhongli City, Taoyuan County, Taiwan, R.O.C</p><p>MATERIALS AND METHODS 1. Polymer solution The polymer solutions were made from polyvinylidene fluoride (PVDF) powders (Mw = 534 000, Sigma-Aldrich, USA), Dimethylformamide (DMF) (Tedia, USA), acetone (Tedia, USA), and Capstone® FS-66 (Sigma-Aldrich, USA). Prior to generation of the solutions, all reagents were not further purified or modified. It is noted that Capstone® FS-66 is an anionic phosphate fluorosurfactant that was provided without solvents.</p><p>2. PVDF solution preparation The PVDF solutions with DMF/acetone solvents were prepared using the following steps: a. 1.6 g PVDF powder was dispersed in 4 g acetone for 30 minutes using a magnetic stirrer. b. The complete PVDF solution was prepared by mixing 6 g DMF with the PVDF/acetone solution. Simultaneously, 0.4 g Capstone® FS-66 was added to the mixture and stirred for a minimum of one hour to reach sufficient homogeneity. c. This surfactant is magnetically stirred for 60 minutes to maintain a minimal foaming. All solutions were prepared and stored at room temperature in a well-ventilated laboratory environment. All containers were sealed with Parafilm to minimize evaporation. Specific content of the solutions is listed in Table S1 below. The concentration of all substances is calculated in weight percentage (wt %) of the solvent. Table S1. Experimental data of PVDF solution. PVDF concentration Solvent ratio Fluorosurfactant (DMF : acetone) concentration 16 wt % 6 : 4 3 wt %</p><p>3. Preparation of direct-write PVDF nanofibers on plastic substrate The in-situ poled PVDF nanogenerator has dimensions of 6 cm × 2cm ×1.5mm (length × width × thickness) that suspended multiple fibers across eleven copper contact electrodes made of 55 μm-thick copper foils, was applied on the top surface of the plastic polyvinyl chloride (PVC) substrate for piezoelectricity measurements.</p><p>NFES IN-SITU POLING PROCESS AND CHARACTERIZATION Figure S1. Near-field electrospinning (NFES) is capable of combining direct-write, mechanical stretching, and in situ electrical poling to precisely deposit piezoelectric nanogenerators onto a flexible or rigid substrate such as plastic or silicon wafers (redrawn after [13])</p><p>The in situ mechanical stretching and electrical poling during direct-write technique by means of near-field electrospinning (NFES) can be schematically illustrated in Figure S1 such that the strong electric fields (greater than 106 V/m) and stretching forces from the electrospinning process naturally align dipoles in the nanofiber crystal. It is experimentally validated by FTIR that the nonpolar α-phase (random orientation of dipoles) is transformed into polar β-phase, resulting the aligned polarity of the electrospun nanofiber. Figure S2.FTIR spectra of the NFES electrospun nanofibers (needle-to-collector distance is 1 mm with applied voltages 1.2 kV) and conventional electrospinning with whipping instability.</p><p>Figure S2 shows FTIR spectra of both NFES and conventional electrospinning samples. It is observed that FTIR spectra of NFES samples exhibit the α-phase related bands at 614, 760,795 and 975 cm-1 and the β-phase related bands at 840 and 1278 cm-1, respectively. Prior data [1] also showed the similar trend such that the α-phase- related bands locate at 614, 765, 795 and 975 cm-1,the β-phase-related bands at 840 and 1278 cm-1 andγ-phase at 1225 cm-1, respectively In comparison, the conventional electrospinning fibres share the similar characteristics in α-, β- and γ-phase-related bands. However, the NFES nanofibers exhibit weaker and broad diffraction peaks, which may be attributed to the small size of the crystallites and the formation mechanism of β-phase can be found in [2]. Both electrospinning processes show the effect of Columbic force promoted β-phase conformational changes to straighter TTTT conformation In particular, the NFES samples show a prominent β-phase related band around 840, indicating the piezoelectric polarity polar β phase through aligning in-situ dipoles inside the crystal of electrospun NFs.[13]</p><p>VALIDATION OF PVDF SPINNABILITY FOR CONTINUOUS AND LARGE- AREA DEPOSITION Figure S3. Plots showing the spinnability and dependence of NFES nanofiber diameters on various processing parameters (a) A 16 wt% PVDF and needle-to- collector distance 1 mm. (b) Needle-to-collector distance. Other parameters are maintained as the followings: 16 wt% PVDF applied voltage 1 kV</p><p>Figure S3 shows the spinnability and dependence of NFES nanofiber diameters on various processing parameters. Though the diameter of nanofibers can be adjusted through applied voltage and needle-tocollector distance, however, the continuous spinnability in large area cannot (shown as red circle). In order to achieve the continuous and large-area deposition, the NFES spinning window is experimentally identified for PVDF solution (shown as green box), which is relatively narrow compared to other commonly used solution such as PEO [12]. </p><p>1. Piezoelectric PVDF and non-piezoelectric polyethylene oxide nanofibers Figure S4. (a) Voltage output of a PVDF nanogenerator subject to continuous stretch and release. (b)Voltage output of electrospun PEO nanofibers subject to continuous stretch and release </p><p>Figure S4 shows the comparison of the electrical outputs generated from piezoelectric PVDF and non-piezoelectric poly(ethylene oxide) (PEO) nanofibers, respectively. Only noise with no visible peaks is observed in the output voltage (Figure S4b), indicating the strikingly different behaviors between PEO nanofibers and PVDF nanofibers (Fig. S4a). Therefore, the possibility of any artifact such as residual charges, friction, or contact potential as the cause of the observed electric outputs can be excluded for the PVDF nanofibers.</p><p>2. Polarity-oriented PVDF nanofibers Electrospun PVDF nanofibers are deposited in two types (A&B), mainly due to the polarity accumulated in the similar or opposite directions (Figure S5a,b, S6a,b). In type A deposition, the PVDF nanofiber arrays basically have the opposite polarity and were cancelling out one another such that the overall electric outputs were relatively small (Figure S5c.d). Red arrows illustrate the poling direction. Since the diameters of PVDF nanofibers are in the range of 0.9 μm -2.5μm, which may explained why the overall electric output is not zero. For the same-direction-poled alignment of type B, the enhancement of both output voltage and current is prominent, indicating the importance on polarity alignment during nanofibers deposition..</p><p>Figure S5. Type A electrospun NFs for opposite-poled alignment (a) Optical image of PVDF nanofibers deposited between two metal electrodes. Red arrows illustrate the poling direction. (b) Schematic electrode pattern and deposited method of Type A electrospun NFs (c)Voltage output subject to continuous stretch and release. (d) Current output subject to continuous stretch and release. NFES conditions are: voltage is 1.0 kV and needle-to-collector distance is 1 mm, flow rate is 0.005ml/hr.</p><p>Figure S6. Type B electrospun NFs for the same direction-poled alignment. (a) Optical image of PVDF nanofibers deposited between two metal electrodes. (b) Schematic electrode pattern and deposited method of Type A electrospun NFs.(c) Voltage output of the PVDF nanofibers subject to continuous stretch and release. (d) Current output of the PVDF nanofibers subject to continuous stretch and release. NFES conditions are: voltage is 1.0 kV and needle-to-collector distance is 1 mm ,flow rate is 0.005ml/hr. 3. Enhancement of electrical outputs Superposition of enhanced voltage and current outputs were demonstrated by making serial and parallel connections of nanogenerators as show in Fig. S7.</p><p>Figure S7. Two nanogenerators were superimposed to enhance the output voltages which (a) nanogenerator #1 and (b) nanogenerator #2 subject to continuous stretch and release. (c) Constructively, output voltages were basically added when two nanogenerators are in serial connection. Output currents of (d) nanogenerator #1 and (e) nanogenerator #2 subject to continuous stretch and release. (f) When two nanogenerators are in parallel connection, output currents add constructively. All measurement data are performed when the two nanogenerators operated in the same strain, strain rate, and frequency.</p><p>References and Notes</p><p>1. Yee W.A., Kotaki M., Liu Y., Lu X.: ‘Morphology, polymorphism behavior and molecular orientation of electrospun poly (vinylidene fluoride) fibers’, Polymer, 2007,48, pp. 512–521 2. Yiin-Kuen Fuh, Li-Chih Lien, Jason S.C. Jang. Micro & Nano Letters, 2012, Vol. 7, Iss. 4, pp. 376–379</p>
Details
-
File Typepdf
-
Upload Time-
-
Content LanguagesEnglish
-
Upload UserAnonymous/Not logged-in
-
File Pages9 Page
-
File Size-