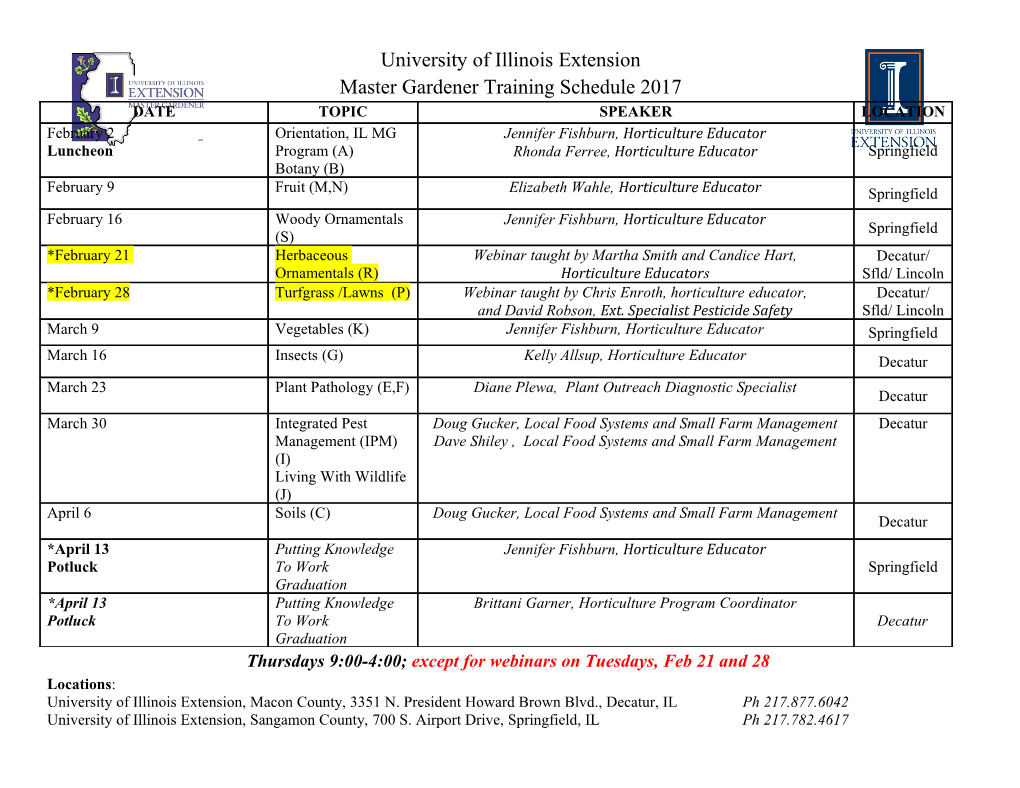
<p>Details of Materials and Methods [Jerbi et al.] (provided as online supplementary material)</p><p>Patient and electrodes implantation</p><p>The patient (female, 39 years old) was a candidate for surgery for drug-resistant partial epilepsy. Magnetic Resonance Imaging (MRI) showed no abnormality. As the location of the epileptic focus could not be identified using non-invasive methods, the patient underwent intracerebral EEG recordings by means of stereotactically implanted multilead depth electrodes (iEEG) (for a detailed description of this methodology, see (Kahane, Minotti et al. 2004)). The presurgical evaluation revealed that the epileptogenic area was in the left anteromedial temporal area. The patient gave informed consent prior to participating in the experiment.</p><p>The patient was implanted with semi-rigid electrodes (0.8 mm diameter), each consisting of a linear array of 10 to 15 recording sites (inter-site spacing of 3.5 mm) (Dixi, Besançon, France). The electrodes were inserted perpendicular to the saggital plane (see Figure 1, main text), therefore recording from both medial and lateral cortical areas, including sulcal cortex. All electrode contacts were identified on each individual stereotactic scheme and then anatomically localized using the proportional atlas of Talairach and Tournoux (Talairach and Tournoux 1988). In addition, computer-assisted matching of a postimplantation computerized tomography (CT) scan with a preimplantation 3-D MRI provided a direct visualization of the electrode contacts with respect to the brain anatomy of each patient (Activis, Lyon, France).</p><p>Recordings</p><p>Experimental data were recorded extraoperatively according to our routine procedure (Kahane, Minotti et al. 2004). Intracerebral recordings were conducted using an audiovideo EEG monitoring system (Micromed, Treviso, Italy) that simultaneously recorded 128 iEEG channels sampled at 1024 Hz (0.1–250 Hz bandwidth) during the experimental paradigm. All signals were re-referenced to their nearest neighbor on the same electrode, 3.5 mm away prior to analysis (bipolar montage). In other words, we do not use a common reference scheme. Recording sites showing pathological activities were excluded from the analysis, and among the remaining sites both common reference and bipolar data were systematically inspected, both raw and high-pass filtered (above 15 Hz). Eye movements were recorded with electro- oculography (EOG) sampled at 1024 Hz and fully synchronized with EEG data acquisition. </p><p>Experimental Paradigm</p><p>The full experiment consisted of three tasks, the present study reports only on the first condition (Temporal pole artifacts were found similarly in the other two conditions). In the first condition (STORY), participants were shown one-by-one, 80 short one-line sentences in french (e.g. ‘THE KING LEFT THE CASTLE BY NIGHT’, after translation). The displayed sentences had between 7 and 13 words. However, in 20 % of trials (16 trials), the sentence was from a completely unrelated story, taken from a sports magazine (‘HIS CLAY-COURT GAME IS REALLY EXCELLENT’). The subject was required to indicate (button press) after each sentence whether it belonged to the main story or not. The presentation parameters were the same for each trial (Figure 1): First, a fixation cross appeared for 2 s instructing the participant to shift her gaze to the left side of the screen. Next, a sentence is displayed and the participant reads it at her own pace until her gaze reaches a fixation cross on the right side of the screen. The participant then pressed a button to display the response panel, asking whether the sentence belonged to the main story (‘left index button press’) or not (‘right index button press’). A further button press launched the next trial. Note the experimental design made it possible to calibrate eye-movements in each trial, since participants were instructed to look at a left and a right fixation cross.</p><p>Stimuli were shown in white on a black computer screen, located 70 cm in front of the participant. The experiment was performed using Presentation® software (Version 0.70, www.neurobs.com). The visual angle between peripheral left and right fixation crosses was 14° (-7° to 7°). Data analysis</p><p>Intracerebral EEG data were analyzed with respect to saccade onsets (‘s’, as shown in figure 1, main text). All saccade onsets were identified from the EOG trace recorded during sentence reading. Identification was offline and semi-automatic: a custom Matlab (The Mathworks, Inc.) program provided first-pass automatic detection of saccade onsets; then, the same program allowed for every detected saccade the visualization of the EOG signal with the detected onset, and manual correction of the marker latencies if needed. </p><p>Intracerebral EEG data were analyzed in the time-frequency (TF) domain and averaged across saccade onsets: a) iEEG data were split into epochs extending from 1 s before the marker to 1 s after; b) for each epoch showing no sign of pathological activity or eye blinks, the bipolar derivations computed between adjacent electrode contacts were analyzed in the TF domain by convolution with complex Gaussian Morlet’s wavelets (Tallon-Baudry, Bertrand et al. 1997). This convolution provided for each trial a TF power map P (t , f) = w (t , f) * s (t) 2, where w (t , f) was for each time t and frequency f a complex 2 2 –1/2 Morlet’s wavelet w (t , f) = A*exp (–t / 2t ) * exp (2ift), with A = (t) and t= 1 / (2f) and f a function of the frequency f: f = f / 7. The investigated frequency range was 1-250 Hz. For the sake of statistical analysis, the TF maps were computed for a set of [50 ms x 6 Hz] time-frequency bins.</p><p>We detected TF regions with systematic power increases time-locked to saccade onset. This amounts to comparing statistically at each frequency the TF power measured at any moment in time with the energy measured during a predefined baseline interval relative to the marker of interest. One test was performed for each time-frequency bin (latency = t, frequency = f) with the null-hypothesis H 0 being that the power measured at frequency f and latency t relative to the marker is not different from the mean energy measured during the baseline at the same frequency. For each TF bin, we used a Wilcoxon test (a nonparametric equivalent of a paired t-test) which compared across epochs the power measured for each TF bin to that obtained for the baseline at the same frequency. The baseline was set to +75 ms to +125 ms relative to saccade onset, i.e. during the subsequent fixation. P values were corrected for multiple comparisons using the False Discovery Rate procedure (Genovese, Lazar et al. 2002). The time-frequency and statistical analysis were performed with a software package for electrophysiological analysis (ELAN- Pack) developed at INSERM U821 (Brain Dynamics and Cognition lab, Lyon, France). </p><p>REFERENCES</p><p>Genovese CR, Lazar NA and Nichols T (2002). "Thresholding of statistical maps in functional neuroimaging using the false discovery rate." Neuroimage 15(4): 870-8.</p><p>Kahane P, Minotti L, Hoffmann D, Lachaux J and Ryvlin P (2004). Invasive EEG in the definition of the seizure onset zone: depth electrodes. Handbook of Clinical Neurophysiology. Pre-surgical assessment of the epilepsies with clinical neurophysiology and functional neuroimaging. F. Rosenow and H. O. Lüders, Elsevier Science.</p><p>Talairach J and Tournoux P (1988). Co-planar stereotaxic atlas of the human brain. 3-dimensional proportional system: an approach to cerebral imaging. New York, Thieme.</p><p>Tallon-Baudry C, Bertrand O, Delpuech C and Permier J (1997). "Oscillatory gamma-band (30-70 Hz) activity induced by a visual search task in humans." J Neurosci 17(2): 722-34.</p>
Details
-
File Typepdf
-
Upload Time-
-
Content LanguagesEnglish
-
Upload UserAnonymous/Not logged-in
-
File Pages2 Page
-
File Size-