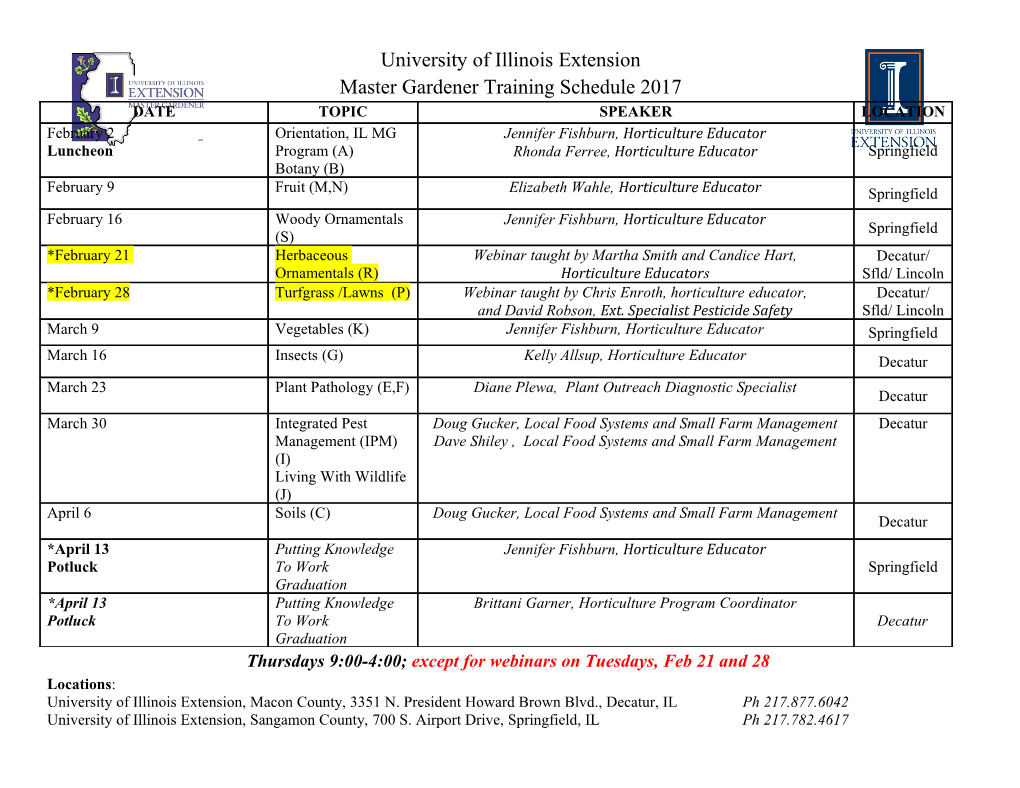
<p> Cosmetic Care and Nanotechnologies: Extending Sensory Perception to the Nanometer Level</p><p>Frédéric Leroy, Gustavo Luengo, Philippe Hallégot L’Oréal Recherche</p><p>Introduction</p><p>From the early stages of human development man has had two basic needs, among others, to fulfill: a need to protect oneself and a need to communicate to others. The former focalized on the use of products to impress or enhance certain feelings, while the later, more advanced, saw cosmetics as a way to protect the original biological surfaces from the external agents.</p><p>FIGURE 1 Figure 1. In the development of Cosmetics, special care must be taken to understand the final interaction of the product with the human substrate.</p><p>But what is a cosmetic?, in general terms it refers to any article to be applied to the various external parts of the human body for cleaning them, perfuming them, changing their appearance and/or correcting body odors and/or keeping them in good condition. </p><p>The success of a cosmetic product is based, not only on its properties, but also on the way it interacts with the biological substrate. This is one of the challenges of the cosmetic scientist: to develop chemicals that eventually will interact with hair or skin in such a way that the main needs are fulfilled: a durable cosmetic effect, a protective action and obviously harmlessness. The heterogeneous structure of hair and skin is not helpful in achieving these goals, taking into account its innate variability of morphology due to its biological origin. For example, the hair surface is known to be hydrophobic but turns to hydrophilic in presence of water. Behind this macroscopic evidence there exists a molecular mechanism that is still poorly understood the control of which could lead to significant applications. The shape of hair is obviously controlled by the chemical composition of the proteins making the fiber, and their spatial organization. Their precise knowledge and the description of the molecular mechanisms which controls the shape could lead to the discovery of new kinds of actives for perming or straightening hair.</p><p>One of the major challenges of the cosmetic scientist is consequently to understand the molecular or supra-molecular structure of the substrates he works on and how they govern their macroscopic properties. And more precisely, he has to study, analyze and understand the relationships between a chemical reaction or interaction at the molecular level, and a physical property at the macroscopic level to conceive and develop new cosmetic ingredients. This challenge is further complicated by the enormous complexity of the biological materials he works on, and the great variety of transformations he wants to achieve on them.</p><p>The cosmetic substrate: skin and hair and their modification The main substrates of interest in cosmetic science are hair and the outermost layer of skin called the stratum corneum. Both are composed of dead cells mainly filled with a specific type of protein called keratin. This protein is characterized by a high content of cystine, an aminoacid that has the capacity to crosslink the protein by intermolecular disulfide linkages. The structure and assembly of these proteins (and other components like lipids) in the cells and the cohesion of the cells give hair and stratum corneum their specific properties, mechanical and tribological among others.</p><p>Skin Skin is the largest organ of the human body and its main role is protection. The structure of skin includes three compartment: epidermis, dermis and subcutaneous tissue (see Figure 2). The epidermis structure is made up of several cell layers: basal, spinous, granular and horny layer or stratum corneum, which is the outermost part of the skin. From the basal layer, The cells migrate upwards, progressively flatten, loose their nucleus and become filled with keratin filaments (keratinization) embedded in the cell proteins remnants. The cells of the horny layer are referred to as corneocytes (see Figure 3), and can be seen to be roughly hexagonal in shape overlapping at the edges with neighboring cells. The actual arrangement of the keratin is still discussed ( helix like hair vs. -pleated sheet), although recent results using X-ray diffraction are more consistent with a model of self-folded keratin sheets. </p><p>FIGURE 2 Figure 2. Scheme representing the complex structure of skin with its different layers: dermis, epidermis and finally horny layer or stratum corneum.</p><p>FIGURE 3 Figure 3. Scanning Electron micrograph (SEM) micrograph showing the corneocytes that form the surface of skin and its outermost layer: the stratum corneum.</p><p>The stratum corneum acts as a mechanical, thermal and chemical barrier against the external environment and is without doubt, the first target of skin-care products. "Indeed the raison d'être of the epidermis is to make the stratum corneum; this is its specific biological mission".</p><p>It is remarkably impressive that the 15 µm -thick layer of stratum corneum is able to prevent the whole human body against water loss and the external physical and chemical aggressions. The barrier effect and the cohesiveness of the stratum corneum is due to the highly structured organization of the corneocytes and the intercellular matrix. In effect, from the simplest structural model widely accepted, the matrix is mainly composed of a lamellar phase of various lipids organized in bilayers that act as a glue keeping the cells together and providing a barrier effect. The lipids are mainly composed of ceramides, cholesterol, fatty acids, and cholesterol esters. It is interesting to note that they do not contain phospholipids which are commonly found other biological membranes. Ceramides, that constitute ~40% of all stratum corneum lipids, are a type of surfactants with a polar head (hydroxyl terminal groups); and a double non-polar saturated tail. They would naturally seem to be ideally suited to form highly ordered and impermeable membranes, and they are believed to play a fundamental role in the bilayer and lamellar structure of the lipids in the intercellular matrix as it is covalently bound to the corneocytes and has a long saturated chain that may extend more than one bilayer distance and therefore act as a link to keep two membranes tightly associated. Hair Despite hair fibre is not a living biological material, it’s even a more complex material than living skin. In fact, it can be considered as a nano-composite material with a highly organized structure that confers its unique physical properties. Hair is a roughly cylindrical fibre (~50- 100 µm in diameter). The overall number of hairs covering a normal human head is comprised between 120,000-150,000, which leads to an important surface area (typically ~6 m2 for 20 centimeter long hair). This is a large surface to cleanse when shampooing for example. (see Figure 4).</p><p>FIGURE 4 Figure 4. SEM of a section of human hair fiber. The diameter of the fiber is ~80µm. The image of the transversal cut shows the complex structure of this nanocomposite, the cortex and the cuticle are clearly visible. Note the typical scaled structure of the cuticle surface.</p><p>Hair structure is complex and can be divided from the center into three layers: the medulla, the cortex and the cuticle (see Figure 5).</p><p>FIGURE 5 Figure 5. Scheme of the hair fiber structure, from the -helical protein, to the microfibril, the macrofibril and the cortical cells. The structure of the cuticle is also shown.</p><p>The medulla is in the center and is composed of honeycomb-like cells with empty spaces aligned along the longitudinal axis of the hair. The cortex is the main part of the hair. It is formed of elongated cortical cells (~100µm in length and ~1-6 µm in diameter) aligned along the axis of the fiber and filled with partially crystallized keratin. This peculiar structure of the cortex gives the hair its unique mechanical properties. Keratin has the ability to crystallize in the form of an -helical structure, basic element of a 4-strand rope arranged in a coiled- coil configuration. Serine, glycine, n-acetyl serine and cystine are the main aminoacids and the chains are stabilised by many inter- and intra- molecular interactions: electrostatic, van der Waals, hydrogen and covalent (disulfide bonds). These units form hexagonal crystals called microfibrils (~7.5 nm in diameter) or intermediate filaments (IF). Moreover, these IF are embedded in an amorphous protein (matrix), as a semi- crystalline sub-structure of the cells called macrofibrils (~100-400 nm in diameter). These imbricate crystalline structures, strongly anisotropic, convert the hair in a true bio- nanocomposite, giving it its unique mechanical properties: high rupture stress and elongation, high elastic modulus, rapid and total recovery even at high deformation.</p><p>The cuticle forms the outer surface of hair. It has an overlapping scale-like structure protecting the cortex. A normal hair has about 6-8 overlapping cells in close contact. In addition and thanks to earlier electron microscopy studies, we know that the cuticle is divided in three layers; the innermost endocuticle, the exoxuticle and the outermost epicuticle. The endocuticle, composed of cytoplasmatic and nuclear remnants has a low cystine content (~3%) and is supposed to be the weakest component of the cuticle structure. The exocuticule is composed of cystine-rich proteins component; the part of the layer closer to the exocuticle is referred to as the A-layer. Its high cystine content suggests that the exocuticle is a highly crosslinked material, therefore producing a more compact material which could explain its high mechanical resistance and behavior as a water barrier. The final structure of the outermost layer of the cuticle is of special interest: the surface properties of hair depend on the physico-chemical properties of this layer. The most recent findings have shown that the epicuticle membrane of hair fibers contain highly crosslinked protein (~75%) and fatty acids (25~%). Among these adsorbed fatty acids, the 18- methyleicosanoic acid (18-MEA) appears to be covalently grafted via thioester linkages to the protein and is considered to play an important role in the physico-chemical and tribological properties of hair. Based on these observations a model has emerged to explain the fine molecular structure of the hair surface. Further measurements need to be done to evaluate the accuracy of this representation (see Figure 6), as well as the extent or ordering of this suspected monolayer; the degree of coverage on the scale structure, its dynamics in the presence of surfactants or various treatments, etc.</p><p>FIGURE 6 Figure 6. Scheme representing the diverse possible scenarios that could take place at the outermost layer of the hair surface. A) Covalently linked lipids like the 18-MEA disorderly attached to the protein. B) The lipids are homogeneously ordered at the surface of the protein. And C) a mixed model is proposed and therefore an heterogeneous coverage can be expected.</p><p>Considering the complexity of the chemical and the physical structure of the different elements constituting hair or skin, it seems extremely difficult to achieve the goal described before: to link a chemical reaction at the molecular level to the modification of a macroscopic physical property. In fact, recent advances in the field of nanotechnologies and nanoanalytical instruments allow the cosmetic scientist to look at these materials with a previously unbelievable spatial resolution and precision. For example, the availability of Atomic Force Microscopy (AFM) and other related techniques leads to measurement of topographical, mechanical and tribological characteristics of hair or skin at the almost molecular level, allowing us to better understand the contribution of the different structural elements of the substrates to their macroscopic physical properties. Moreover, the recent development of very high resolution and high sensitivity micro-analytical tools, such as Secondary Ion Mass Spectrometers (SIMS) and nano-SIMS, gives us the opportunity to do a chemical analysis at the same spatial resolution than AFM and therefore to correlate chemical and physical information both obtained at a near-molecular level.</p><p>ATOMIC FORCE MICROSCOPY</p><p>AFM is based on the interaction of a sharp point (tip) attached to the end of a cantilever with the surface of interest (Figure 7). The interaction is measured by the cantilever bending that is itself monitored by the displacement of a laser beam reflected on the back of the cantilever to a XY detector. The lateral movement of the tip or the surface is accomplished using a piezo- electric element which provides scanning capabilities.</p><p>FIGURE 7 Figure 7: Basic AFM scheme. The tip’s small size is perfectly suited to characterized human hair.</p><p>AFM gives true topographic information without any special constraint (no vacuum, no metallic coating), contrary to the electron microscopes. In addition, measurements can be done in many close-to-real conditions (water or any other chemical condition), highly appreciated by the cosmetic scientist. As the measurement of the topographic height profile is straightforward and quantitative, experiments based on the quantification of the scale step height, and surface roughness give insight into the hair structure and its sensitivity to external parameters (physical or chemical). If we look closer at hair surface structure, the scales of the cuticle overlap determining a saw-tooth profile characterized by a step-height. Most studies reflected a wide distribution of cuticle-step heights. Nowadays, cosmetic ingredients interact with the hair surface at a very small level. The common presence of a nanometric thin film can change enormously the macroscopic sensorial perception and therefore is essential to determine its presence and its morphological distribution. </p><p>To illustrate this, Figure 8 shows a high resolution image of the hair surface after a cosmetic treatment. Scales are clearly visible, as well as rests of an active, in this case a conditioning polymer. Note that the real profile is easily measured following the indicated line. Similarly Figure 9 shows the real surface morphology of a corneocyte.</p><p>FIGURE 8 Figure 8: Example of the types of image obtained by AFM on hair surface. Upper: 3D topographic image of the cuticle; Lower: height profile through a line parallel to the axis of the fibre.</p><p>FIGURE 9 Figure 9: Topographic images of a corneocyte. Upper left; low resolution image of the whole cell; Upper right: detail of the cell surface; Lower: height profile through the marked line.</p><p>At the macroscopic level, the friction of hair is asymmetric due to the orientation of scales. If sliding is done from root to tip (touch) or when looking into fibre-fibre interaction, the role of the sub-micron structures is believed to be dominant. Only with the advent of AFM techniques, we can have access to the local friction and progress towards the understanding of both, the hair surface and the molecular origin of macroscopic friction. The latter is, among others, a key factor when studying the effects produced by hair products such as hair-setting, hair-styling, conditioners and shampoos.</p><p>For example, the outermost layer of hair is composed of lipids. Some of them are covalently linked and although their role is not fully understood it is believed that its presence helps preserve the integrity of the fibre. Figure 10 shows the fine details of friction contrast recently observed in our laboratories by this method on human hair fibres in which the covalently linked fatty acid 18-methylicosanoic (18-MEA) has been removed using hydroxylamine. Topographic images showed that no morphological changes were done to the fibre. In spite of that, the friction pattern changed, became more erratic and formed sub- micrometers domains suggesting that the lipid layer is not covering the hair surface homogeneously any more. This is important because certain cosmetic treatments are known to eliminate those fatty acids. Shampoos and conditioners, can be better formulated to compensate for this loss</p><p>FIGURE 10 Figure 10: Friction images of the surface of a cuticle cell; Upper: image of the virgin fibre; Lower: friction image of the same area after removal of covalently bound lipids. Brighter area correspond to higher friction force where the lipid monolayer has been removed. Knowing the rigidity of the cantilever, its deflection when the tip is interaction with the substrate can be converted to a force. AFM can then be used as a nano-force transducer. Force spectroscopy allows the continuous measurement of the interaction of the tip with the cosmetic substrate. In essence, the scanner brings the sample and the tip together while monitoring the force induced on the cantilever that holds the tip. The system records both the approaching and retracting curve as force applied versus distance travelled by the piezo. The retracting or unloading curve is usually the one that holds information regarding the strength of the interaction; a minimum in the retracting curve (pull-off force) is associated to the adhesion. Depending on the load applied on the surface (loading force) the tip may penetrate the substrate (nano-indentation). The degree of penetration can give information about the Young modulus of the material. This method allows obtaining mechanical information about the hair or skin constituents: cortex, cuticle, etc. and therefore localize the effect of cosmetic treatments at the core of its structure.</p><p>Figure 11 shows a contact image of one hair section showing the different layers that form the cuticle. Several indentations can be observed that show the high positional capabilities. After this type of experiments values of modulus and hardness were extracted allowing for a comparison among the different components.</p><p>FIGURE 11 Figure 11: Topographic image of a hair cross section after several indentations at various forces.</p><p>A latest, very original and unique, application of AFM is the monitoring of the interaction forces between a surface and a tip previously functionalized. Several methods have been developed to chemically modify AFM tips. For example by thiolisation; tips that have been coated by a thin layer of gold can react with thiol-terminated long chain fatty acids. This allows for a monolayer to be grafted on the tip surface exposing the chemical groups present at the other extreme of the chain (Figure 12).</p><p>FIGURE 12 Figure 12: Schematic representation of a functionalized AFM tip.</p><p>By this methods, it is possible to obtain tips that expose hydrophilic or hydrophobic chemical groups like CH3, COOH, NH2; etc. Tips become more like chemical probes measuring the interaction forces at the nano-scale (van-de Waals, electrostatic interactions). If measurements are carried out in water, it is possible to follow the influence of pH, for example. For example, we measured adhesion forces using this procedure by tapping randomly on the surface of hair in water using an hydrophobic tip(CH3). As expected, forces were much higher on the surface of natural hair instead of bleached hair. In effect, Hydrophobic forces are predominant due probably to the covalently liked lipid layer that covers natural hair. A powerful application of this type of measurements is the capacity to obtain adhesion maps; by measuring at each point the exhibited adhesion. An example is also shown in Figure 13, representing the adhesion forces between an AFM tip functionalised with CH3 groups and a hair fibre partially covered by a polymer. Brighter colors represent higher adhesion helping us determine the presence of a small thin film where a polymer is adsorbed.</p><p>FIGURE 13 Figure 13: Cartography of adhesion forces between a CH3 functionalized tip and a hair fibre partially covered with a polymer. Bright regions correspond to higher forces where the polymer is adsorbed.</p><p>Secondary Ion Mass Spectroscopy</p><p>But, to fully understand how the properties of hair (or skin), it is necessary to complete the physical information given by instruments such as AFM with a chemical information at the same level, i.e. at the nanometer scale. Secondary Ion Mass Spectroscopy is the tool that can provide us with this information.</p><p>In SIMS (Figure 14) a primary ion beam is scanned onto the sample surface, which is consequently sputter-eroded. Constitutive elements of the eroded volume (generally a few atomic layers) are collected and subsequently identified by mass spectrometry. Image distribution of any isotope from any element can be acquired with a spatial resolution of about 100nm when using conventional instruments. With use of nano-SIMS, spatial resolution has been dramatically improved down to less than 50 nm, together with an improved detection limit. Furthermore, the instrument allows true simultaneous acquisition of up to five different masses exactly at the same location on the sample. For the first time, accurate high-resolution quantitative imaging of isotope ratios can be conducted.</p><p>FIGURE 14 Fig. 14: Principle of SIMS : SIMS reveals atomic mass sample composition.</p><p>The simultaneous quantification of different isotopes and/or elements allows the rapid and precise evaluation of the composition of hair (figure 15). One of the most obvious applications is the precise localization in hair of sulfur-rich proteins by comparing the images obtained at the mass corresponding to 32S, and those corresponding to a typical signal of proteins (12C14N). The images clearly indicate that the sulfur-rich proteins are preferentially located in the exocuticle and are non-uniformly located in the cortex. On the opposite, the medulla is exclusively composed of proteins with a low sulfur content (figures 15, 16).</p><p>FIGURE 15 Figure 15: Simultaneous isotope mapping of 32S (with magnified image of the cuticle on the right), 12C, 13C, 12C14N and 13C14N on one hair transverse cut.</p><p>FIGURE 16 Figure 16: Simultaneous mapping of proteins (12C14N, in green) and 32S (red) showing the specific location of sulfur rich proteins. Exocuticle is particularly rich in sulfur, when medulla only contains low sulfur proteins.</p><p>Another problem which can be elucidated with nano-SIMS is the precise localization of exogenous molecules in the substrate. Active ingredients need to be bio-disponible to be efficient, i.e. they must reach at the precise location where they will have an effect. The nano- SIMS not only gives a high resolution in the location of these molecules, but also allows us to follow the penetration of molecules without any specific signature (color, specific spectroscopic feature, …) by tagging them with a specific isotope, radioactive or stable. The high sensitivity of nano-SIMS makes possible to follow very small variations of the isotopic ratio and thus to detect and quantify these molecules with a high accuracy. On figure 17, an organic molecule tagged with 13C has been applied to hair. The profile of the 13C/12C ratio across the hair obviously indicates that this molecule accumulates in the cuticle, but does not penetrate the cortex of the fibre.</p><p>FIGURE 17 Figure 17: Evolution of the ratio of 13C/12C and 13C14N/12C14N signals along a line scan across a transverse section of a hair treated with 13C-tagged molecule. (a) 32S image of the cut; (b) Isotope ratios along the scan; (c) Magnified view of the 13C14N/12C14N image in false color</p><p>The major problem to which the cosmetic chemist is confronted is the exact localization of the chemical reactions occurring between the active molecules he develops and the substrate he wants to transform. A better knowledge of hair or skin properties also needs a better understanding of the structural compartments involved in the definition of the physical properties. For example, permanent waving of hair is obtained through a two-step process: first a mechanical relaxation leading to a deformation of hair, then a stabilization of the new shape. First step is achieved by reducing the disulfide bonds, thus lowering the density of cross-links in the proteinic material which allows the hair to mechanically relax; the second step consists in the fixation of the proteins new configuration by creating new cross-links via the oxidation of thiol groups in new disulfide bridges (figure 18). Unfortunately, all the disulfide bonds do not have the same contribution to the mechanical properties of hair, and consequently to its shape. We know especially that many of these linkages are intra-molecular linkages which have a negligible contribution to these properties. The best reducing agent for perming hair will be the molecule which specifically acts on the disulfides bonds stabilizing the protein structure, which are incompletely known. Moreover, the mechanical relaxation of the hair will be better if the reduction process is homogeneous throughout the whole fibre. Nano-SIMS is useful for following the reduction process in the hair. This is done by reducing the hair, then blocking the newly formed thiol groups with 14C-iodoacetic acid. Observation of the isotopic ratio 14C/12C on hair cross-sections gives an accurate image of the reduced regions. On figure 19, we can see that ammonium thioglycolate (the most common reducer) leads to a very homogeneous reduction throughout the fibre, when tri-hydroxymethyl phosphine, another kind of reducer, only reduces the disulfide bonds located in the outermost part of the cortex. Thus, it is easy to understand why the phosphine is an excellent reducer, but an ineffective perming agent.</p><p>FIGURE 18 Figure 18 : Schematic principle of the two-step process of hair permanent waving. 1: Reduction of disulfide bridges and mechanical relaxation; 2: oxidation of thiols to disulfide linkages to fix the new protein conformation.</p><p>FIGURE 19 Figure 19 . Elemental mapping of 12C14N and 14C14N in hair reduced with ammonium thioglycolate (upper images) or tri-hydroxymethyl phosphine (lower images) then blocked with 14C-Iodoacetic acid.</p><p>Nano-SIMS technical performances don’t permit today to identify and characterize the chemical modifications at the level of molecules. Its spatial resolution (35 nm) however is enough to localize these modifications in the different structural compartments of hair. This provides a dramatic improvement to our knowledge of the reactivity and the chemical transformations of our substrates. SKINCHIP®</p><p>Nanotechnologies and micro-machining coming from the electronic industry offer the cosmetic scientist other opportunities for creating unique sensors for fast and accurate measurement cosmetic properties of hair and skin in vivo, or exploring with a new view our materials. An example is the SkinChip®, aimed to get images of skin surface.</p><p>The SkinChip is based on an integrated electronic device, the Touchip®, initially produced by ST Microelectronics for sensing fingerprint in a few seconds. The sensor is composed of an micro-array of 360 x 256 capacitive micro-sensors. Each sensor-cell contains an active capacitive feedback circuit whose effective capacitance is modulated by contact with the skin close to the surface of the sensor. When the skin is in close contact with the sensor surface (fingerprint ridge) the feedback capacitance is maximized, but becomes minimized when the skin is less close from the sensor surface (fingerprint valley) (Fig. 20).</p><p>FIGURE 20 Figure 20: (a) detailed view of the ST Microelectronics micro-sensor; (b) Schematic principle of the sensor</p><p>L’Oréal derived the SkinChip® from this sensor for the visualization of skin micro-relief and the measurement of several topographic and physico-chemical parameters. By using specifically developed image analysis software, different characteristic parameters of the skin surface can be analysed. The morphology is easily assessed by detecting the density and the main orientations of the lines describing the micro-relief. Differences in the topography of the skin at various locations on the body are clearly seen (Figure 21). The variation of the critical parameters of the skin micro-relief (especially the main orientations of the micro-wrinkles) with respect to the age is easily assessed (Figure 22).</p><p>FIGURE 21 Fig.21: Aspects of the skin surface on different locations revealed by the non-optical imaging device. (a) Volar forearm; (b) Cheek of middle-aged male; (c) Crow pad wrinkles; (d) Lip.</p><p>FIGURE 22 Fig.22: (a) Main directions of the micro-relief primary lines of the ventral forearm of a young man; (b) Typical plot of the index of occurrence vs. the angle (relative to the line perpendicular to the forearm axis).</p><p>Beyond the topographic observation of the skin, the analysis of the grey levels of the SkinChip® image gives information on the hydration of the skin. Incorporation of water in the stratum corneum induces a change in the conductivity of the skin in contact with the sensor, thus modifying its response. For example, the objectivation of the effect of a moisturizing cream on skin surface is easily detected with the SkinChip® (Figure 23).</p><p>FIGURE 23 Fig.23: Image of dry skin (volar forearm). Upper : untreated zone; Lower : zone treated with a moisturizing skin-care cream. Skinchip® is thus a unique tool giving in a few seconds information on skin previously obtained in minutes or hours with multiple systems much more complex to operate.</p><p>Conclusion</p><p>Skin and more especially hair are complex biological nano-materials. Their study will be greatly improved by the use of nanotechnology-based instruments which allow the cosmetic scientist to probe with high resolution and sensitivity their chemical , physical and cosmetic properties</p><p>Acknowledgements</p><p>We Wish to thank Prof. C. LECHENE, Department of Medicine, Brigham and Women's Hospital and Harvard Medical School, Boston, USA and Dr. H.-N. MIGEON, Laboratoire d'Analyse des Matériaux, Centre de Recherche Public - Gabriel Lippmann, Luxembourg for realizing the nano-SIMS observations. Thanks also to Dr B. QUERLEUX, L’Oréal Recherche for developing the SkinChip® softwares.</p><p>References</p><p>Zviak C (1986) The Science of hair care. Marcel Dekker, New York Robbins CR (2002) Chemical and physical behavior of human hair, 4th ed. Springer, New York Negri A, Peet DJ, Rivett DE (1996) Text. Res. J. 87:608 Wertz PW, Downing DT (1989) Stratum corneum: biological and biochemical considerations In: Swarbrick J, Guy RH (eds) Transdermal drug delivery. Marcel Dekker, New York Binnig G, Gerber CH, Stoll E, Albrecht TR, Quate CF (1987) Europhys. Lett. 3:1281 Adams MJ, Briscoe BJ, Wee TK (1990) J. Phys. D Appl. Phys. 23:406 Luengo G, Leroy F (2004) The science of beauty on a small scale: nanotechnologies applied to cosmetic science In: Applied Scanning Probe Methods; Bhushan B, Fuchs H, Hosaka S (eds). Elsevier. Phillips TL, Horr TJ, Huson MG, Turner PS, Shanks RA (1995) Text. Res. J. 65(8): 445 O'Connor SD, Komisarek KL, Baldeschwieler JD (1995) J. Invest. Dermatol. 105(1): 96 Smith JR (1998) J. Microsc. 191: 3 Castaing R, Slodzian G (1962) Microanalyse par émission ionique secondaire. J. Microsc. 1:395 Slodzian G, Daigne B, Girard F, Boust F, Hillion F (1992) Scanning secondary ion analytical microscopy with parallel detection. Biol. Cell. 74:43 Hallégot P, Peteranderl R, Lechene C (2004) In-Situ Imaging Mass Spectrometry Analysis of Melanin Granules in the Human Hair Shaft. J. Invest. Dermatol. 122:00</p>
Details
-
File Typepdf
-
Upload Time-
-
Content LanguagesEnglish
-
Upload UserAnonymous/Not logged-in
-
File Pages10 Page
-
File Size-