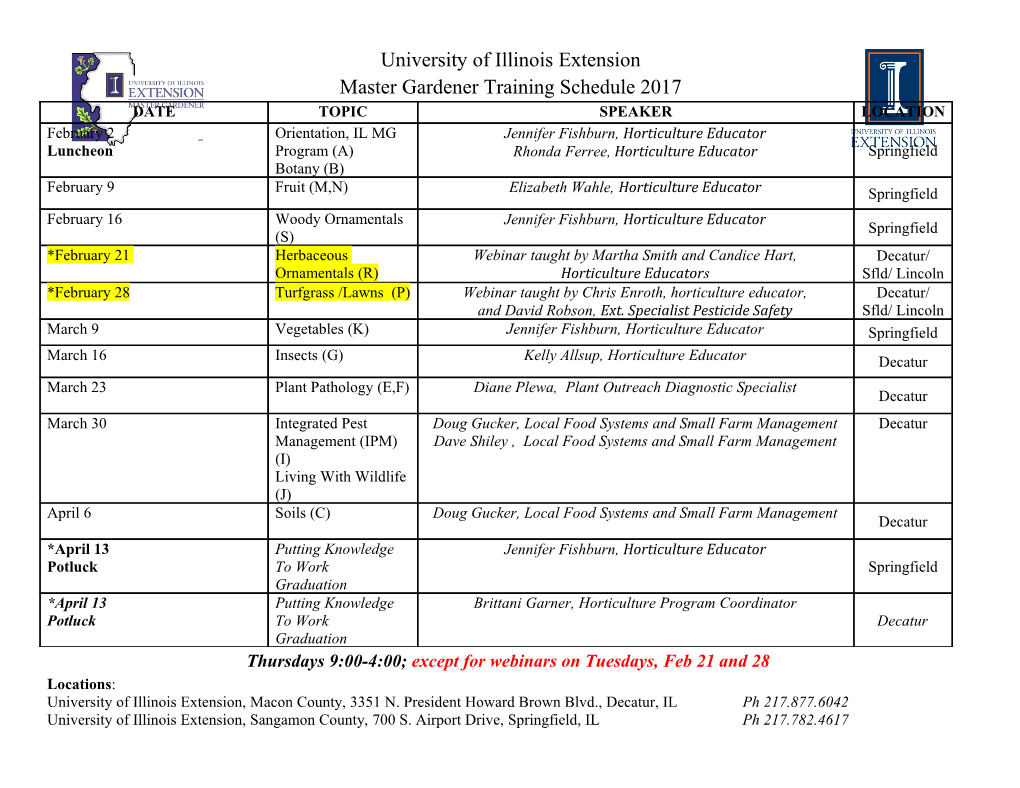
<p> INVASION OF NORTHEASTERN FORESTS BY INTRODUCED SPECIES: SPATIALLY-EXPLICIT FEEDBACKS BETWEEN POPULATION DYNAMICS AND ECOSYSTEM PROCESSES Results from Prior NSF Support DEB 9815390: Feedbacks between calcium cycling and canopy tree dynamics in a north temperate forest. PIs: C. Canham, G. Lovett, G. Likens, N. van Breemen, and A. Finzi; Amount: $449,980; Dates 4/99 – 3/02. DEB 0087214: Models as tools for synthesis of the effects of hurricanes on temperate and tropical forests. PIs: C. Canham, N. Brokaw, N. Fetcher, B. Haines, and J. Thompson; Amount: $299,901; Dates 10/2000 – 9/2003. DEB 0516066: Collaborative Research: Natural Disturbance and Human Land-Use as Determinants of Tree Community Dynamics in a Subtropical Wet Forest. PIs: C. Canham and M. Uriarte; Amount $145,933; Dates 9/205 – 8/08. Five NSF grants over the past 15 years have provided the core support for research on the development of spatially-explicit, individual-based models of forest dynamics. The models encapsulate an emerging neighborhood theory of forest dynamics (Canham and Uriarte, in press) The 3 awards active during the past 5 years are listed above. The original model (SORTIE) was developed for mixed deciduous-conifer forests of southern New England, but studies by Canham and colleagues have expanded research to temperate coniferous forests of British Columbia, boreal forests across Canada, temperate forests of New Zealand, urban forests in Kentucky, and tropical forests of Puerto Rico, with studies of processes ranging from feedbacks between tree species and soil nutrient dynamics to the effects of hurricane disturbance. A hallmark of all of the research is a clear linkage between field research and modeling, and the development and use of novel approaches to data analysis based on likelihood methods and information theory. During this period, Canham and colleagues have completely re-engineered the software to produce a new, open-source platform (SORTIE-ND; www.sortie-nd.org) for spatially-explicit simulation of forest dynamics using neighborhood theory and models. The core research supported by the 5 NSF grants has produced over 46 publications (including papers in press), including 38 on which C. Canham is an author or co-author. The peer-reviewed journal publications have appeared in Canadian Journal of Forest Research (7), Ecological Applications (5), Ecological Modelling (2), Ecological Monographs (3), Ecology (3), Forest Ecology and Management (4), Journal of Ecology (4), Oecologia (2), Oikos (3), and 7 other journals. The individual publications are identified with an asterisk in the References section. Nine students have completed PhD’s working on research with SORTIE: John Casperson, Adrien Finzi, Jim Hill, Richard Kobe, and Eric Ribbens (Univ. of Connecticut); Jaclyn Schnurr and Chris Tripler (Idaho State Univ.); Michael Papaik (Univ. of Massachusetts); and Feike Dijkstra at the Agricultural University in Wageningen (Netherlands). Current graduate students working with Canham on research related to SORTIE-ND include Dominique Gravel (PhD student, University of Quebec at Montreal) and Laurel Williams (MS student, University of Vermont). Many of our research assistants have subsequently entered PhD programs in ecology, including Coelie Hoover (Univ. of Georgia), Erika Latty (Cornell), Kristi Silber (Rutgers), Swee May Tang (Michigan State), and Christina Tonitto (Princeton). Two NSF Minority Postdoctoral fellows have been mentored by Canham while working on these projects (Chris Tripler and Maria Uriarte), and a Fullbright postdoc from Spain (Lorena Gomez) is currently working in Canham’s lab on research directly related to the work outlined in this proposal.</p><p>1 Introduction While much of the research on invasive plant species has focused on population and community dynamics, invasive plants can have profound impacts on ecosystem processes (e.g. Vitousek and Walker 1989, Gordon 1998, Ehrenfeld and Scott 2001, Ehrenfeld 2003). The initial establishment of an introduced species will take place in the context of the conditions created by the existing plant community and local soil conditions (including soil faunal and microbial communities). Once a new exotic species becomes established, there is the potential for distinctive transformation of ecosystem processes by the invader, with potential implications for both the further rate of invasion by the exotic species and the regeneration of native species. A transformation of ecosystem properties to conditions more favorable to invasive conspecifics is a potential mechanistic explanation for the “lag phase” (Hobbs and Humphries 1995, Sax and Brown 2000) common between establishment of exotic species and actual invasion outbreaks. A fundamental tenet of invasion ecology is that introduced species typically invade areas opened by disturbance (Elton 1958, Burke and Grime 1996, review in Grime 2001), although recent studies also recognize that invasions themselves can feedback to alter subsequent disturbance regimes (Mack and D’Antonio 1998). Indeed, the majority of plants currently listed as invasive in the northeastern U.S. are ruderals, and usually become established in large openings (Torrey Herbarium 1996). The exotic plant species currently capable of invading closed canopy forests in the northeastern U.S. are largely herbs, shrubs and vines, and have high dispersal rates (e.g. Japanese barberry, Berberis thunbergii) (Torrey Herbarium 1996, Hunter and Mattice 2002). Exotic tree species in the region, in contrast, are still primarily restricted to the margins of intact forests, particularly disturbed urban and suburban forests (Anderson 1999, Knapp and Canham 2000, Webb et al. 2000). Two of the most common exotic tree species in the region, Norway maple (Acer platanoides) and the tree of heaven (Ailanthus altissima), are invasive dominants primarily in stands which had experienced some form of disturbance at the time of initial invasion (Webb and Kaunzinger 1993, Anderson 1999, Martin 1999, Knapp and Canham 2000). However, the unusual life-history traits of these two trees suggest they are capable of invading intact forests as well: Norway maple’s high tolerance of shade (Kloeppel and Abrams 1995) and the tree of heaven’s extraordinarily high growth rates in small canopy gaps (Knapp and Canham 2000) make them potentially potent invaders of native forests. There is an important distinction between invasion of forests by understory species (shrubs, herbs, and vines) versus canopy tree species. There is mounting evidence that both the biomass and diversity of the understories of northeastern forests are generally far from saturated, and have, in fact, been depleted in many sites by past land-use practices (Foster 2002, Flinn and Vellend 2005). Thus, successful establishment of exotic species of shrubs and herbs may or may not lead to reductions in the abundance and diversity of native understory species. The canopies of northeastern forests, regardless of past land-use, are generally much closer to “saturation” (where density and biomass at stand maturity are effectively a zero-sum game). Thus, any measurable invasion by introduced tree species can be expected to come directly at the expense of the abundance of native tree species. This will have direct consequences for the ecological processes, ecosystem services, and economic benefits of those forests. This raises a critical set of questions: are intact, native forests resistant to invasion except following large disturbance events? Alternatively, have factors such as slow dispersal and slow juvenile growth rates obscured what will be an inexorable invasion of native forests by gap- phase and shade tolerant exotic tree species such as Norway maple and tree of heaven, with understories of exotic, shade-tolerant shrubs and herbaceous species? As the exotic species gain</p><p>2 a foothold, will they initiate a sequence of transformations of ecosystem processes that accelerate or retard the rate of additional invasions? These questions are far from academic, since the answers will determine the nature of the long-term threat to native forests and will aid in the development of management to meet the threat. More fundamentally, it raises the question of whether the assessment that invasive species are typically r-selected (e.g. Rejmanek 1996, Reichard and Hamilton 1997, Grotkopp et al. 2002) has been biased by the focus on species that are the most rapid invaders following introduction to a landscape, missing the longer-term but more profound implications of transformation of ecosystem processes due to invasion of northeastern forests by shade tolerant, late-successional plant species. Linking Field Research and Models to Assess Plant Invasion and Ecosystem Transformation Advances in computing power in the past 20 years have led to a proliferation of spatially- explicit, individual-based models of population and ecosystem dynamics (Judson 1994, Dunning et al. 1995, Mooij and DeAngelis 1999). Spatially-explicit models of forest dynamics such as ZELIG (Urban et al. 1989) and SORTIE (Pacala et al. 1996) shift the focus from the dynamics of discrete patches or gaps (sensu Watt 1947, Levin and Paine 1974) to interactions among individual organisms, and allow a more detailed treatment of environmental heterogeneity at a variety of spatial scales. In effect, the individual-based models encapsulate an emerging theory of “neighborhood” dynamics, in which fine-scale spatial interactions regulate the demography of component tree species (Pacala et al. 1996, Law and Dieckmann 2000). The spatial distribution of component species, in turn, regulates spatial variation in a whole host of community and ecosystem properties, with feedbacks on population dynamics. Studies of neighborhood dynamics appear to be a particularly powerful vehicle for the integration of population dynamics and ecosystem processes. Attempts to “link species and ecosystems” have been a pervasive theme in ecology in the past decade (Jones and Lawton 1994). Canopy tree species exert strong control over many forest ecosystem processes, which in turn, have feedbacks that influence canopy tree dynamics (Canham and Pacala 1994, Rothe and Binkley 2001). Studies of forest ecosystem processes have traditionally used plot designs that average across variation in local neighborhood composition and structure. It is very difficult using this approach, however, to predict changes in ecosystem processes as canopy composition changes. This is particularly true when the spatial interactions of different species are non- additive, and the properties of a plot cannot be predicted from simple data on the relative abundance of species within the plot. The emerging theory of neighborhood dynamics provides an alternative approach that incorporates the mechanistic, spatially-explicit interactions between species dynamics and ecosystem processes, including heterogeneity in the physical environment. Spatially-explicit models then provide the tool for synthesizing these processes into spatial and temporal predictions of system dynamics (Gratzer et al. 2004). The shift to spatially-explicit, individual-based models has been facilitated by new methods of analysis of field data (i.e. Ribbens et al. 1994, Kobe et al. 1995, Canham et al. 2001), in which measurements of environmental factors (e.g. light), critical demographic rates (e.g. recruitment), and ecosystem processes (e.g. nitrogen mineralization) are analyzed in terms of the spatial distributions of neighboring trees and physical environmental factors. The analyses are based on likelihood methods and information theory (Edwards 1992, Hilborn and Mangel 1997, Burnham and Anderson 2002, Canham and Uriarte, in press), and allow a tight linkage between the models and parameterization of the models from field data. They also allow quantification of uncertainty in both parameter values and model predictions (Pacala et al. 1996).</p><p>3 To date, assessments of the potential for an exotic species to become invasive have generally used retrospective analyses of past invasions (e.g. Reichard and Hamilton 1997, Grotkopp et al. 2002). As suggested above, these studies may underestimate the long-term invasiveness of exotic shade tolerant and gap phase species in forests. In more general terms, predictions of the invasiveness of an introduced species must be made in the context of both specific environments and the traits of the native species with which the exotics must compete and interact (Williamson and Fitter 1996). We suggest that mechanistic models of forest dynamics, based on neighborhood theory, offer a promising approach to the assessment of invasion potential by introduced plant species. For the past 15 years, the PIs and colleagues have worked on the development of a spatially-explicit, mechanistic model of the dynamics of forests of southern New England [SORTIE, and now SORTIE-ND (www.sortie-nd.org)]. We focus the research proposed here on the transition oak – northern hardwood forests of southern New England to take advantage of the substantial previous field research and modeling of the dynamics of the 9 dominant native tree species, and because of the gradients of soil resource availability present in that region (e.g. Canham et al. 1994, Pacala et al. 1994, Ribbens et al. 1994, Kobe et al. 1995). Our research during the past 7 years has focused on feedbacks between tree population dynamics and forest ecosystem processes, and the role of fine-scale variation in soil nutrient availability for niche partitioning and the dynamics of tree species (e.g. Finzi et al. 1998a,b, Bigelow and Canham 2002). Our recent research clearly demonstrates that saplings of all of the major native tree species respond to fertilization with either calcium, nitrogen, or both (Finzi and Canham 2000, Bigelow and Canham 2002, Tripler et al. 2002, Bigelow and Canham submitted). Variation in soil moisture availability is also important, but only at the extremes of a topographic gradient (Casperson et al. 1999). Our research has revealed striking heterogeneity in those soils, as a function of both variation in the mineral content of the glacial till (vanBreemen et al. 1997) and the effects of native tree species in the immediate neighborhood (e.g. Canham and Pacala 1994, Finzi et al. 1998a,b, Dijkstra 2003, Dijkstra and Smits 2002). Recent results suggest a very strong degree of niche partitioning of the native tree species along gradients of nitrogen (N) and calcium (Ca) availability (Bigelow and Canham 2002, Bigelow and Canham, submitted). As an example, in sites with higher levels of Ca in the parent material, sugar maple abundance increases dramatically, in response to (1) a suite of mechanisms by which sugar maple trees enhance the levels of exchangeable (available) Ca in surface soils (Dijkstra 2003, Dijkstra and Smits 2002), and (2) higher growth rates and survival rates of seedlings and saplings, particularly under low light (Kobe et al. 1995, Bigelow and Canham, submitted). In effect, soil Ca levels determine the shade tolerance of sugar maple. As Ca levels increase (in part because of feedbacks from the presence of adult sugar maples), sugar maple becomes more competitive with beech, and eventually displaces beech (vanBreemen et al. 1997). During the past 2 years, under USDA funding, we have completed the field studies needed to parameterize the basic demographic processes of seed dispersal, seedling recruitment, and juvenile growth and survival for the 2 introduced tree species at our research sites – Norway maple (Acer platanoides) and tree of heaven (Ailanthus altissima). That research will allow us to use SORTIE-ND to do a first approximation of the dynamics of establishment and spread of the two exotic tree species within the context of the existing assemblages of native tree species (but without incorporation of ecosystem transformations). In effect, those simulations represent the simplest scenario, in which there are no ecosystem effects of the introduced species, and where light is the only resource limiting growth of juveniles of the two exotic tree species.</p><p>4 A Neighborhood Framework for the Study of Ecosystem Processes Forests are ideal candidates for neighborhood analyses (Canham and Uriarte, in press). Canopy trees provide the bulk of both the physical and biological structure - living and dead, above and belowground - in forest ecosystems. The dominant ecosystem fluxes (e.g. throughfall, litterfall, nutrient uptake, leaching losses) are primarily vertical rather than horizontal, and the identities of canopy trees overhead and nearby have distinctive effects on a whole host of ecosystem processes at the “neighborhood” scale [0-50 meters] (Binkley and Giardina 1998, Finzi et al. 1998a,b). It is relatively easy to map the distributions of forest trees in large plots (Condit 1998), and readily obtainable measures of plant size such as DBH (diameter of the stem at 1.35 m height) can be used in allometric equations to predict above and belowground biomass (Jenkins et al. 2003) and the physical dimensions of tree crowns (e.g. Canham et al. 1994). Consider the state of some ecosystem property y at a point p (i.e. soil nutrient availability, nutrient return via leaf litterfall, growth of a plant at that location, etc.). For practical reasons, y(p) is measured in a sample drawn from a location of finite dimensions (p’). Perhaps the most basic neighborhood model is a simple additive function of the general form (Canham and Uriarte, in press): n y( p ) g( p ) f (xi ) (1) i1</p><p> for i = 1..n “agents” (xi, typically trees) within some maximum distance (r), and where g(p’) is some function of the physical and biological attributes of location p’. Each agent (xi) has a vector of attributes; for a tree this would typically include species, DBH, and location relative to location p’, and may also include environmental conditions at the location of each tree. In principle, r should be chosen to be large enough that all agents that have measurable impact on the process at location p’ are included. The neighborhood function may take more complicated algebraic forms to reflect non-additive effects of the “agents” (i.e. plants in the neighborhood) on the ecosystem process of interest. A key component of f (xi) is the characterization of the “footprint” of the agent: i.e. how does the effect of a neighboring tree on the ecosystem property at location p change with the distance of the tree from p. To date, neighborhood models have largely been used in studies of population dynamics and resource competition among sessile organisms (particularly plants). We believe that there is much wider potential application of the methods for any ecosystem process where the spatial configuration of component organisms plays a central role. Studies of the spatial distribution of leaf litterfall (Ferrari and Sugita 1996, Staelens et al. 2003) represent one obvious example. The approach is likely to be seen as brute phenomenology by investigators who seek mechanistic explanation of ecosystem processes at much more reductionist levels. For studies of forest ecosystem dynamics, however, we would suggest that there is already overwhelming empirical evidence that tree species differ significantly in their effects on a whole host of ecosystem processes, and a growing literature on the exact mechanisms that underlie these differences. We argue that there is a critical need for research on how to integrate the net effects of the myriad mechanisms by which both introduced and native species alter ecosystem processes, and by which they interact with each other. There have been a number of attempts to link models of tree population dynamics with models of forest ecosystem dynamics, but other than the pioneering work with LINKAGES (Post and Pastor 1996), none to our knowledge have yet explicitly incorporated a neighborhood approach. Neighborhood models will be critical where there are non-additive effects of mixtures of different species (Finzi and Canham 1998, Gartner and Cardon 2004). In our framework, non-</p><p>5 additive effects are accommodated using higher-order (nonlinear) terms in Eqn. 1. If there are strong nonlinearities, non-spatial models will give biased predictions of the effects of the mixtures, as a result of Jensen’s inequality (Duursma and Robinson 2003, Pastor et al. 2000). For example, both antagonistic and synergistic effects of species mixtures are common in studies of leaf litter decomposition (Gartner and Cardon 2004). Recent studies also suggest that the size and identity of neighboring plants can alter the traits of an individual (e.g., litter quality, plant architecture or water use efficiency) that determine the ecosystem effects of that individual (Eviner 2004). There has been some recent progress in predicting at least the direction of the effect (antagonistic vs. synergistic) of different mixtures of species from the traits of component species (Wardle et al. 1998, Eviner and Chapin 2003), but the wide range of empirical results found in recent studies suggests that a great deal more field research will be needed to predict a priori the magnitude and direction (antagonistic vs. synergistic) of non-additive effects. The challenge for a neighborhood approach is to use the results of recent experiments and process studies to construct relatively simple models that capture the mechanisms that underlie these non-additive effects of both native and introduced species, without resorting to the brute force (and extremely large number of resulting parameters) required to describe any potential non- additive effects among all possible subsets of species (Finzi and Canham 1998). Objectives Our overarching goal is to develop an integrated, spatially-explicit framework using neighborhood theory to explore and model the relationships between plant invasions and ecosystem processes in northeastern forests. We will focus our research on a suite of four exotic plant species that are currently invading northeastern forests: garlic mustard (Alliaria petiolata), Japanese barberry (Berberis thunbergii), tree of heaven (Ailanthus altissima) and Norway maple (Acer platanoides). The list of potential invaders is obviously much longer, but these four species offer a range of life histories, growth forms, and potential ecosystem effects. We will employ experimental and observational methods in the field, and spatially-explicit designs and new statistical tools based on likelihood methods for our modeling. The proposed research has 3 components. 1. Development of predictive, spatially-explicit neighborhood regression models of the effects of introduced plant species on ecosystem processes and tree population dynamics. There are two components to this part of the proposed research: (a) field studies and analyses to develop neighborhood models of key ecosystem properties, including the availability of the two soil resources (N and Ca) that previous studies have shown influence juvenile growth and survival of native trees, and (b) field studies and analyses to develop neighborhood models of the effects of proximity to the introduced species on the growth and survival of both the native and exotic tree species. Our previous research has shown that growth and survival of native tree species at our study sites are strongly influenced by the availability of both nitrogen and calcium. The spatially-explicit approach used in our neighborhood models will allow us to predict spatial variation in these two key resources as a function of the distribution and abundance of both native and exotic species (and underlying variation in soil parent material). We will explicitly test whether the effects of invasive exotic trees in native communities are lower or higher than expected in isolation (e.g. in monocultures). If these non-additive effects exist, then resistance to invasion in a given environment will vary depending on both the identity of the invader and the spatial configurations of the native species. </p><p>6 A key question that will be addressed by our research is whether there are threshold local densities of the exotic species above which the ecosystem effects of the invaders create positive or negative feedback loops that either accelerate or retard the subsequent spread of the invasive species. One source of a threshold would be if the effects of the exotic species on resource availability were a nonlinear function of their local abundance, with the most dramatic effects occurring as clusters of exotic species develop. This is likely to be the case with extremely low understory light levels forming under clusters of Norway maples. This is also the pattern we see in the effects of native species such as sugar maple on soil nitrogen and calcium availability (e.g. Finzi and Canham 1998), where the distinctive species-specific patterns are most pronounced when the species are distributed in clusters. These dynamics could give rise to an effective “lag phase” during which the exotic species reach critical density and spatial distribution, followed by accelerated spread and significant effects on the regeneration of native tree species. Research on Norway maple in its native habitat in Europe suggests that this species is similar to sugar maple in having high fluxes of both N and Ca in leaf litterfall (Norden 1994a,b). To our knowledge there have been no studies of the effects of tree of heaven on soil nutrient dynamics. We hypothesize that tree of heaven, whose leaves and bark have been shown to be rich in secondary compounds (Heisey 1990, 1996), will produce low-quality litter and potentially allelopathic litterfall and root exudates able to inhibit soil biota biomass and activity, and therefore depress the rates of nutrient cycling. As a consequence of a negative feedback, litter quality will be lower and further drive down microbial abundance, eventually depressing the fertility of the system. Norway maple, in contrast, usually grows in fertile soils and appears to be similar to sugar maple in having high fluxes of both N and Ca in leaf litterfall (Norden 1994a,b). We expect it to produce high quality litter that stimulates soil biota and nitrogen cycling, generating a positive feedback on soil fertility and nutrient availability. These types of cascading effects have been scarcely explored in invasives ecology, although their study appears crucial to understand how exotic species can contribute to changes in ecosystem processes. 2. Analysis of the effects of canopy disturbance on invasion and ecosystem transformation. Surveys document a strong correlation between disturbance and most plant invasions, yet the specific ways in which disturbance may intact with ecosystem transformation by exotic species are poorly studied. In the early stages of invasion by an exotic, both its abundance and residence time are dwarfed by native species. Hence, the demographic advantage and legacy effects of native species in intact forests may overwhelm the potential for exotic species to transform ecosystem processes, even for those exotics which have strong ecosystem effects. Disturbances, particularly large-scale ones, have the potential to allow exotics to establish in numbers sufficient to alter processes - through erosion, changes in nutrient status, soil microbial changes, etc. - in ways that may reduce subsequent resistance to invasion. We will use both experimental studies of natural disturbances (canopy gaps) and comparative studies of the effects of human-induced disturbance (logging) to explore 3 specific questions: a) Are canopy disturbances necessary for the exotic species to build sufficient numbers to significantly alter ecosystem conditions and processes? b) What specific effects of a disturbance are critical for establishment of the exotics (i.e. increased light, nutrient availability, seedbed substrate availability)? c) Is there a minimum disturbance size or severity required for establishment of a given exotic species? 3. Integration and Synthesis The results of the field research for the first two objectives will provide the functions and </p><p>7 parameter estimates needed to explicitly link the effects of the four exotic plant species on ecosystem processes to the population dynamics of both the native and exotic tree species, using the neighborhood modeling framework provided by SORTIE-ND. For reasons outlined later, we do not propose to make population dynamic predictions for the exotic herb and shrub species (but will rather use different scenarios of spread of those two species as input to the model). Integrating our results in SORTIE-ND will allow us to provide quantitative predictions of the potential course of invasion by the two exotic tree species, and the implications of that invasion for the abundance and diversity of the native tree species, as well as for changes in a suite of ecosystem state variables. It will also allow us to determine whether modifications of ecosystem processes by the exotic species create positive or negative feedbacks that either accelerate or retard the subsequent spread and dominance of the invasive species by altering resource conditions in the understory. Simulation of both natural disturbance (i.e. windthrow) and anthropogenic disturbance (i.e. logging, and mortality of native species due to exotic pests and pathogens) will allow us to evaluate the degree to which invasion by the introduced species is influenced by canopy disturbance. These advances in our understanding of the introduced species will also help shed light on the pressing issue of the ecosystem conditions that increase community invasibility (Hobbs and Humphries 1995, Davis et al. 2000), and will provide useful insights into the mechanisms regulating the invasion of native forests by introduced species. The Cast of Characters: Transformation of Northeastern Forests by Four Introduced Plant Species Garlic Mustard (Alliaria petiolata) Garlic mustard is one of the few invasive herbs that can dominate the ground layer of northeastern forests, growing in early spring and late fall when many native species are dormant (Nuzzo 2000). Although maximum growth rates of garlic mustard have been documented at 50% of full sunlight (Nuzzo 2000), garlic mustard thrives under light levels that vary from deep shade to full sunlight (Meekins and McCarthy 2000, Myers et al. 2005). The rapid spread of garlic mustard in North American woodlands in recent decades, with resultant declines in native biodiversity, has led to the investigation of the competitive mechanisms used by this species. Studies by Meekins and McCarthy (2000) compared the effects of light, nutrient addition and density on the establishment and growth of garlic mustard and concluded that while nutrient resource availability and competition for space were key factors in determining success in the initial invasion, subsequent growth and spread were primarily determined by light availability. Other factors that favor garlic mustard over native species are high seed production (Nuzzo 1999), low rates of herbivory by deer (Nuzzo 2000), and potentially allelopathic inhibition of the germination of native species (Prati and Bossdorf 2004). Japanese Barberry (Berberis thunbergii) Japanese barberry is a habitat generalist that grows well in full sunlight in fields and forest edges, but it is also a shade tolerant species that forms dense stands in closed canopy forests at light levels as low as 1% (Brunelle and Lapin 1996, Silander and Klepeis 1999). Reproduction is mainly sexual, but vegetative reproduction comes from both root sprouts and rooting branches. Barberry produces abundant seed which has germination rates as high as 90% (Rhoads and Block 2002). Barberry seed is dispersed by birds and small mammals. In the spring, Japanese barberry is one of the first shrubs to leaf out, and it is not browsed by deer, making it a dominant understory species in heavily browsed forests (Rhoads and Block 2002). Ironically, colonization of the forest understory by Japanese barberry may have benefits for some songbird species that </p><p>8 experience higher fecundity than those species nesting on the ground (Schmidt et al. 2005). Barberry suppresses the growth and biomass of native species, and rapid growth of stems and production of new stems helps to buffer Japanese barberry from environmental stress (Silander and Klepeis 1999, Ehrenfeld 1997). There is also evidence that barberry alters soil microbial communities, soil chemistry, and nutrient dynamics (e.g. Kourtev et al. 2002, 2003) Tree of Heaven (Ailanthus altissima) The tree of heaven exhibits many of the traits normally associated with a successful invasive species (Berger 1990, Bright 1995). It produces very large numbers of wind dispersed seeds (Hu 1979) which suffer much lower rates of predation by native small mammal seed predators than do seeds of native tree species (Ostfeld et al. 1997, Manson and Stiles 1998). It grows quickly in high light (Bazzaz 1979, Hu 1979, Feret 1985, Heisey 1990), and can reproduce asexually via root sprouts (Hu 1979). It is particularly common in urban forests (Newton 1986). An allelopathic compound (ailanthone) has been isolated from bark and foliage of A. altissima (Heisey 1990, 1996). Although there have been no demonstrations of effective phytotoxicity under natural conditions, there have been reports that bark extractions influenced the foraging behavior of termites, and may reduce colonization of trees by the insect (Grace 1997). Although tree of heaven is recognized as an important exotic weed by the Nature Conservancy (Hoshovsky 1999), early studies considered it shade-intolerant and unable to invade closed forests of native species (e.g. Feret 1985, Newton 1986, Cronk and Fuller 1995). There is mounting evidence to challenge this traditional view, prompted in part by documentation of the abundance of the species in intact forests (e.g. Kowarik 1995). Knapp and Canham (2000) examined the ability of tree of heaven to colonize small canopy gaps in an oldgrowth forest in New York, and concluded that while the species clearly requires canopy openings, its rapid growth rates would allow it to outcompete all native gap-phase species. Indeed, tree of heaven’s ability to reach mature size during a single period of release in a treefall gap would make it an effective competitor with even the most shade tolerant species in these forests, since the native shade tolerant species require several periods of release in successive gaps in order to reach canopy size (Canham 1985, 1990). Thus, while tree of heaven appears to be dependent on disturbance, even very small-scale disturbance associated with the normal gap- phase dynamics in forests may be sufficient to allow significant rates of invasion. Norway Maple (Acer platanoides) Studies have shown striking effects on the structure and species richness of the native understory community when Norway maple becomes established in the canopy (e.g. Wyckoff and Webb 1996, Martin 1999). Invasion by Norway maple has economic implications as well, threatening the abundance of valuable timber species such as sugar maple (Acer saccharum), red oak (Quercus rubra) and black cherry (Prunus serotina). It has a shade tolerance comparable to American beech (Fagus grandifolia) (Kloeppel and Abrams 1995), prolific seed production, rapid growth, widespread horticultural use, and tolerance of a broad range of environmental stresses. It is one of the most common yard and street trees nationally, and the most popular species at nurseries, ensuring a widespread and plentiful seed source (Santamour and McArdle 1982, Bassuk 1990, Nowak and Rowntree 1990). It is found across a broad climatic spectrum in its native range and it grows “optimally” over more than half of the U.S. (Nowak and Rowntree 1990). Eradication efforts are difficult given its resprouting ability and often super abundant advance regeneration (Webb et al. 2000). There is surprisingly little published about the population dynamics and ecology of </p><p>9 Norway maple, even in its native habitat in Europe (Andrzejczyk and Brzeziecki 1995, Niinemets 1998). Consequently, uncertainty surrounds the invasive ability of A. platanoides in North America, and little action has been taken to control Norway maple’s spread domestically. Some experts feel that it may only dominate in sites located in an urban/suburban matrix where its seed is plentiful and its pollution tolerance confers a competitive advantage (Mehrhoff pers. comm.). Other researchers suggest it can invade natural areas (Webb and Kaunzinger 1993, James and McFadden 1993, Martin 1999, Webb et al. 2000). The limited research conducted to date, including our own preliminary results, has clearly demonstrated its ability to become abundant and even dominate not only open, disturbed areas (Anderson 1999) but intact forests as well (Webb and Kaunzinger 1993, Martin 1999, Webb et al. 2000). Our recent studies provide the most conclusive evidence to date that Norway maple can establish from seed in closed- canopy stands (Martin et al. submitted). Research Plan Study Sites The proposed research will be conducted in and around the 3,000 ha Great Mountain Forest (GMF) in northwestern Connecticut. GMF is located on Canaan Mountain, at elevations of 300-500 m. Soils are typically acidic, well-drained, sandy loams derived from glacial till over mica-schist bedrock. For the past 15 years we have used GMF as the principal field site for the development of SORTIE and SORTIE-ND. Our study sites at GMF are in second-growth (~80 - 130 yr. old) stands with a history of logging but no history of agriculture. Forest structure is relatively simple, with no major native shrub or subcanopy tree species. The most common species in the understory is Dennstaedtia punctilobula (hayscented fern). The forest is in the transition zone between oak-dominated forest of the central Appalachians and the northern hardwoods of New England. Our previous research has focused on the nine dominant native tree species in the region: Tsuga canadensis (eastern hemlock), Fagus grandifolia (beech), Acer saccharum (sugar maple), Acer rubrum (red maple), Betula alleghaniensis (yellow birch), Prunus serotina (black cherry), Quercus rubra (red oak), Fraxinus americana (white ash), and Pinus strobus (white pine). Both garlic mustard and Japanese barberry are increasingly common in forests of the region, particularly in stands nearest populated areas. While neither Norway maple nor tree of heaven are currently abundant within the interior of Great Mountain Forest, both species are common in stands adjacent to roads and nearby villages. Development of Neighborhood Models to Link Ecosystem Properties, Disturbance, and Population Dynamics Basic Approach: Equation 1 (page 5) provides the starting point for our general approach to the development of neighborhood regression models of the effects of the distribution and abundance of native and exotic plant species on both key ecosystem processes and plant population dynamics (Objective #1). The models will also incorporate the effects of disturbance, to address Objective #2. In essence, our approach is a form of spatial regression, in which the state of a dependent variable (i.e. exchangeable Ca concentration, growth of a plant) at a given location is a function of both (a) physical and biological properties (i.e. mineralogy of the parent material) at that specific location, and (b) the spatial distribution of the key neighborhood “agents” (primarily trees, in our case) around that location. The functions (models) developed by our approach can then be used to “scale-up” to predictions of the spatial variation in the value of the dependent variable through the input of a map of the distribution of the agents (i.e. trees) and a map of the spatial distribution of the local physical and biological properties in (a) that are </p><p>10 determined to have a significant effect on the dependent variable of interest. Note that this is exactly how the functions (models) developed from our field research will be incorporated into SORTIE-ND (described in more detail, below) We propose to incorporate canopy disturbance in the analyses in two ways. First, there is ample evidence that there are important biological legacies left behind after the death of a neighboring canopy tree, due to both the physical structure of coarse woody debris, and residual but transient effects of the presence of the live tree on soil properties in the neighborhood. Since the rooting location, species, and approximate size (DBH) and time since death can be determined in the field for trees that have died in the recent past (within 10-20 years), both live and dead trees can be included in the models as “agents” in f (xi). Second, nearby disturbances can alter the physical and biological properties of the specific sample location [i.e. g(p’) in Eqn. 1] in measurable ways (i.e. the effects of canopy gaps on soil moisture and temperature). In effect, our analyses will allow explicit quantification of both the spatial “footprint” of a canopy disturbance (as in Canham et al. 1990 for effects of gaps on understory light levels), and the change in that footprint over time since the death of the canopy tree. Thus, our approach provides a clear, quantitative pathway by which canopy disturbance and the spatial and temporal dynamics of forest composition and structure can be linked to spatially-explicit and temporally dynamic predictions of ecosystem properties. As those ecosystem properties change, they can feed back to alter the future dynamics of the species. Hypothesis testing in our framework is accomplished through a model comparison approach (Johnson and Omland 2004, Canham and Uriarte in press). For example, to test whether there is sufficient evidence to conclude that there are non-additive effects of the mixtures of species within a neighborhood, we compare the strength of evidence - using likelihood and model comparison metrics such as Akaike’s Information Criterion (AIC) – for simple additive models versus more complex models that incorporate non-additive terms. One of the PIs (Canham) has extensive experience in the analytical/statistical methods used in the development of spatially-explicit, “neighborhood” models, for processes ranging from landscape-scale models of lake chemistry (Canham et al. 2004, Maranger et al. 2006) to a wide range of population dynamic processes, including seed and seedling dispersion (LePage et al. 2000, Uriarte et al. 2005, Greene et al. 2004), seed predation and small mammal distribution (Schnurr et al. 2004), and neighborhood models of tree competition (Canham et al. 2004, Uriarte et al. 2004a,b, Canham et al. in press). The proposed research will represent the first formal use of these methods to link tree population dynamics to forest ecosystem properties. Sampling Design: The development of the neighborhood models of both ecosystem properties and demographic rates (growth and survival) uses a common sampling protocol. Stands are chosen that represent a broad range of conditions in terms of (1) physical environments, particularly the gradient in parent material mineralogy that our previous work has shown to be so important to both nutrient cycling and tree population dynamics in these forests, (2) canopy composition and structure, including variation in the abundance of all 4 of the introduced plant species, and (3) canopy disturbance, particularly variation in position within and among canopy gaps of a range of sizes. Since our analytical methods are a form of regression, it is not critical that we sample randomly across the landscape - we are not trying to make inferences about average conditions across any given landscape. Rather, we are trying to develop predictive, mechanistic models of the neighborhood processes that control spatial and temporal variation in the dependent variables. Thus, the most critical requirement of the sampling is to distribute broadly across sample space. In practical terms, since our neighborhood</p><p>11 models use spatial information on the locations of canopy trees, the sampling is done in mapped stands. We have already established ~ 10 ha of mapped stands from previous work at our study sites, including 6 mapped stands (each ~ 1 – 1.5 ha in size) in locations that vary in the abundance of the four introduced species. We will need to establish additional mapped stands to expand the range of conditions to include areas with varying levels of canopy disturbance. We will use a combination of both naturally occurring gaps (particularly to sample across a range of time since disturbance) and experimentally created gaps (using felling of trees) to sample across a sufficient range of disturbance conditions. An “observation” in our analyses consists of a measurement of a given dependent variable (i.e. N mineralization) at a single location in one of the stands. We will distribute sampling within stands so that observations are a minimum of 15 m apart from one another to reduce concerns about spatial autocorrelation, although the parameter estimates from our likelihood-based regressions are generally very robust in this regard. Perhaps the most critical requirement for all of our field sampling is for relatively large sample sizes. Our spatial non- linear regression models require use of optimization routines (using simulated annealing, with either proprietary code written by C. Canham, or using a new library of R functions for simulated annealing developed by C. Canham and L. Murphy). Confidence in the convergence of the routines typically requires a minimum of 10 observations per parameter in the model. Since we generally start with “full” models that require at least 1-2 parameters per species of “agent” (tree), even basic additive models start with typically 15 or more parameters (although the model comparison process typically results in simplification to models with fewer parameters). Thus, we plan to sample at least 250 locations. At each location, we will measure the suite of ecosystem processes and properties described in the next section, and conduct an experiment with transplanted seedlings (described in the second section below). Field Methods – Neighborhood Models of Ecosystem Properties: Our previous studies demonstrate that either or both N and Ca (along with light) limit growth and survival of juveniles of the native tree species at our study sites (Tripler et al. 2002, Finzi and Canham 1998, Bigelow and Canham 2002, Bigelow and Canham submitted). Our previous studies also document strong effects of the native tree species on the dynamics of both N and Ca (e.g. Finzi et al. 1998a,b, papers by F. Dijkstra and colleagues in Reference section). However, those studies have simply demonstrated that the native species both respond to and have an effect on variation in the availability of those two soil nutrients. We have not yet done the field research needed to develop neighborhood models of the effects of the distribution of the native species on those resources, let alone incorporate the effects of the introduced plant species. Thus, the primary focus for our neighborhood analyses of ecosystem processes will be on the dynamics of N and Ca, specifically on the dynamics of both mineralization and inorganic pool sizes for those two nutrients, in a neighborhood context. Net N and Ca mineralization will be measured with in situ mid-summer incubations, using our standard methods (Finzi et al. 1998a, Dijkstra 2003). We use standard KCl extractions for N and BaCl extractions for Ca (Finzi et al. 1998b, Dijkstra 2003). In order to provide more breadth in our assessment of the nature of the potential ecosystem transformations caused by the introduced species, we will also include measurements of total mid-summer soil C and N pools (using an Elantech CNS analyzer). All soil measurements will be separated into forest floor and upper mineral soil (0-15 cm) layers (Finzi et al. 1998a). Because parent material mineralogy is such an important driver of variation in Ca availability at our sites, we will use X-ray fluorescence on a sample of soil taken at 15-20 cm depth to quantify total inorganic soil pools of Ca (Finzi et al. 1998a). Finally, in order to test for </p><p>12 any coarse but distinctive effects of the introduced species on soil microbial communities, we will measure soil microbial biomass and respiration at each sample location, using chloroform fumigation-incubation methods (Jenkinson and Powlson 1976, Voroney and Paul 1984). Field Methods – Neighborhood Models of Interactions between Introduced and Native Species: The abundance of saplings of the native species varies along soil fertility gradients at our sites (Bigelow and Canham 2002), indicating that site and neighborhood-specific variation in growth and mortality begin to act on even small tree seedlings. Thus, we will use transplanted seedlings of 6 representative native tree species (beech, hemlock, sugar maple, white ash, red maple, and red oak) and the 2 introduced tree species (Norway maple and tree of heaven) to develop neighborhood models of the effects on seedling growth and mortality of (1) the distribution and abundance different native and introduced tree species, and (2) local ecosystem properties - particularly light levels (measured using fisheye photography), N and Ca availability, and parent material mineralogy. Five seedlings of each of the eight tree species will be planted in quadrats at ~ 200 of the 250 sample locations. Growth and survival of the seedlings will be followed for 2 years, and both average growth (as a continuous variable, measured on the seedlings that survive in a quadrat) and survival (as an ordinal response variable – the number of seedlings that survive) will be used as dependent variables in separate neighborhood models for each species. Seedlings will be germinated from either locally collected and stratified seeds, or from seeds obtained from regional, commercial seed sources if necessary. We have conducted field experiments with comparable numbers of seedlings before (i.e. Canham et al. 1999). Statistical Analyses – Specification of Alternate Regression Models, Parameter Estimation, and Model Comparison: There is an inevitable tension between the benefits of simplicity and parsimony versus the desire for mechanistic richness in our explanations of a phenomenon. Much of our motivation for the proposed research comes from a conviction that there is a need to balance the incredible richness of mechanistic detail that has emerged from the study of invasive species with a simple framework that integrates the myriad ways that native and introduced species interact to regulate ecosystem processes. Our neighborhood analyses face this tradeoff in microcosm. We will follow the general approach outlined in Canham and Uriarte (in press – preprint available at http://www.ecostudies.org/people_sci_canham.html) in the development of the functions (neighborhood models) used for the analysis of each of the dependent variables (whether an ecosystem process or a demographic rate). In brief, we will specify alternate models (functions) that represent alternate hypotheses about the nature of neighborhood controls on the dependent variable. The art of developing the alternate regression models is in finding functions that encapsulate known mechanisms, but in the most parsimonious way. Tests for the presence of non-additive effects of mixtures of native and invasive species, in particular, offer a significant challenge, for reasons outlined in Finzi and Canham (1998). This is simply because there are so many known mechanisms by which mixtures of species can interact in non-additive ways, and any given subset of species may interact in different ways. Once the alternate models are specified, maximum likelihood estimates of the parameters of the regression models will be determined using simulated annealing (a global optimization routine). We will use AIC to choose between alternate models, and will use standard metrics of goodness of fit to evaluate the best models. We will impose one additional constraint on the development of the neighborhood regression models. One of the hallmarks of previous research with SORTIE-ND has been the tight linkage between the empirical functions developed from field research and the actual functions used in the simulation model. We will adhere to that discipline here, and will take </p><p>13 computational efficiency into account in selecting the functional forms used in the neighborhood regression models, since they will be incorporated directly in SORTIE-ND. Our recent neighborhood models of competition between adult trees (e.g. Canham et al. 2004, Uriarte et al. 2004a,b, Canham et al. in press) provide an example: there are much more complex neighborhood models of tree competition in the literature, but they would be prohibitively slow to implement in a simulation model. Synthesis and Simulation using SORTIE-ND The software for the original SORTIE model (Pacala et al. 1996) has been completely re-engineered in the past 3 years to produce an open-source, flexible simulation environment for spatially-explicit dynamics of forest ecosystems. Source code and manuals for both users and developers of the new model, SORTIE-ND (for “Neighborhood Dynamics”) are available on the web (www.sortie-nd.org). In summary, the model simulates the dynamics of two different types of “objects”: populations of individuals (typically trees, divided into distinct life-history stages that are defined by the user), and grids (of user-specified grid-cell size). The individuals are spatially-explicit. The grids are raster objects that can be either static (as in spatial variation in parent material mineralogy) or dynamic (as in the concentration of exchangeable Ca). The dynamics of both individuals and grids are governed by a user-defined set of behaviors (i.e. growth as a function of light, or Ca mineralization as a function of the distribution of tree species in the neighborhood). The primary motivation for re-engineering the model was to make it easy for new users to add new behaviors to the model. Any behavior (whether a demographic process or ecosystem process) can be either deterministic or stochastic. The most basic output from the model is a record of the spatial variation and temporal dynamics of the state variables (both populations of individuals and grids of ecosystem properties and processes). The neighborhood regression models developed under the field research proposed here will be used directly as a new set of behaviors in SORTIE-ND. Note that all of the core demographic functions for the dynamics of seedling recruitment and juvenile growth and survival (as a function of light) for both the 9 native species and the two introduced tree species have already been parameterized from previous research at our sites. The analyses done under the research proposed here will represent the first formal incorporation of soil nutrient dynamics in SORTIE. The new behaviors will allow us to do spatially-explicit simulations of the effects of invasion by the introduced species on both ecosystem processes and the performance of the native tree species. They will also provide our first formal application of neighborhood models to the prediction of linkages between tree population dynamics (whether of native or introduced species) and ecosystem properties and processes. Our modeling will treat the two introduced tree species as populations in the model, with full predictions of the dynamics of their invasion. Predictions of the long-term dynamics of invasion by the introduced shrub (Japanese barberry) and herb (garlic mustard) are beyond the scope of the research proposed here. Instead, our neighborhood regression models will treat the abundance (biomass) of both of those species as a feature of the immediate local environment at a sampling location (i.e. as an element of g(p) in Eqn. 1). For modeling in SORTIE-ND, we will then treat these two species as dynamic grids, but with dynamics that represent different fixed scenarios of change in abundance and spatial distribution over time (i.e. independent of feedbacks from ecosystem properties or the distribution of tree species). Wind is the dominant form of natural disturbance at our study sites. We have developed a wind disturbance submodel for SORTIE-ND (Papaik et al. 2005), based on empirically- derived, species-specific variation in susceptibility to windthrow (Canham et al. 2001). The </p><p>14 disturbance submodel will allow us to test invasion dynamics by the introduced species under different scenarios of wind disturbance severity. We have also developed an interface to allow specification of human disturbance via logging (Coates et al. 2003). That interface will also allow us to simulate disturbance in the form of selective loss of a single species dues to an introduced pest or pathogen (e.g. Jenkins et al. 2000). Project Schedule and Management Field work during the first field season will focus on setting up the seedling transplant study and mapping additional stands needed for the neighborhood models. Growth and survival from that experiment will be monitored for two years. Field work during the second year will add the analysis of all of the dependent variables used for the neighborhood models of ecosystem processes (e.g. N and Ca mineralization and pool sizes, total soil C and N pools, microbial biomass). The primary emphasis during the third year will be on development of the neighborhood regression models, and integration of those results in SORTIE-ND. All of the four investigators will participate in the neighborhood modeling and subsequent simulation with SORTIE-ND. One of the explicit goals of the project is to give the three new investigators (Myerson, Martin, Gomez) experience with both the likelihood methods and simulation modeling, using the approaches developed by Canham and colleagues over the past 15 years. Broader Impacts The proposed research will directly address the degree to which four important introduced species represent a threat to the biodiversity, functioning, and ecosystem services provided by northeastern forests. While much of the emphasis in invasive species research in this country has focused on ecosystems (particularly grasslands and wetlands) where rates of invasion by classically “weedy” species have been very rapid, the research proposed here focuses on the more gradual but potentially pervasive invasion of northeastern forests by shade tolerant plant species. Since mature forest canopies are essentially a zero-sum game, any significant invasion by the two introduced tree species will come at the expense of the abundance of native tree species. This will have direct consequences for the ecological processes, ecosystem services, and economic benefits of those forests. The likelihood methods used in the development of our neighborhood models are a powerful analytical tool for ecologists. Canham teaches an intensive, graduate and post-doctoral level course in the use of these methods in forest ecology with Dr. Maria Uriarte of Columbia University. The course has been taught each of the last 3 years (twice at IES, and once at the Universidad Austral de Chile in Valdivia, Chile) to a total of 60 students from 5 countries (roughly half graduate students, half with PhDs). Results from the research proposed here will be used to develop new examples and labs (tutorials) to use in the course, and to expand the focus of the course from population and community ecology to ecosystem science. The new course materials (lectures, labs, and readings) developed under the research proposed here will be made available on the course website (http://www.ecostudies.org/lmfe.html). The proposed research will also represent a significant expansion of the use of the SORTIE-ND simulation model. As with our likelihood methods, we will develop tutorials on modeling based on our approach, and will post them on the SORTIE-ND website (www.sortie-nd.org). The proposed research will provide direct training and research experience for a graduate student and undergraduate research assistants each summer. We will also recruit at least 1 student each summer in the IES Research Experience for Undergraduate (REU) program. That program has a strong emphasis (and a good track record) on minority recruitment.</p><p>15</p>
Details
-
File Typepdf
-
Upload Time-
-
Content LanguagesEnglish
-
Upload UserAnonymous/Not logged-in
-
File Pages15 Page
-
File Size-