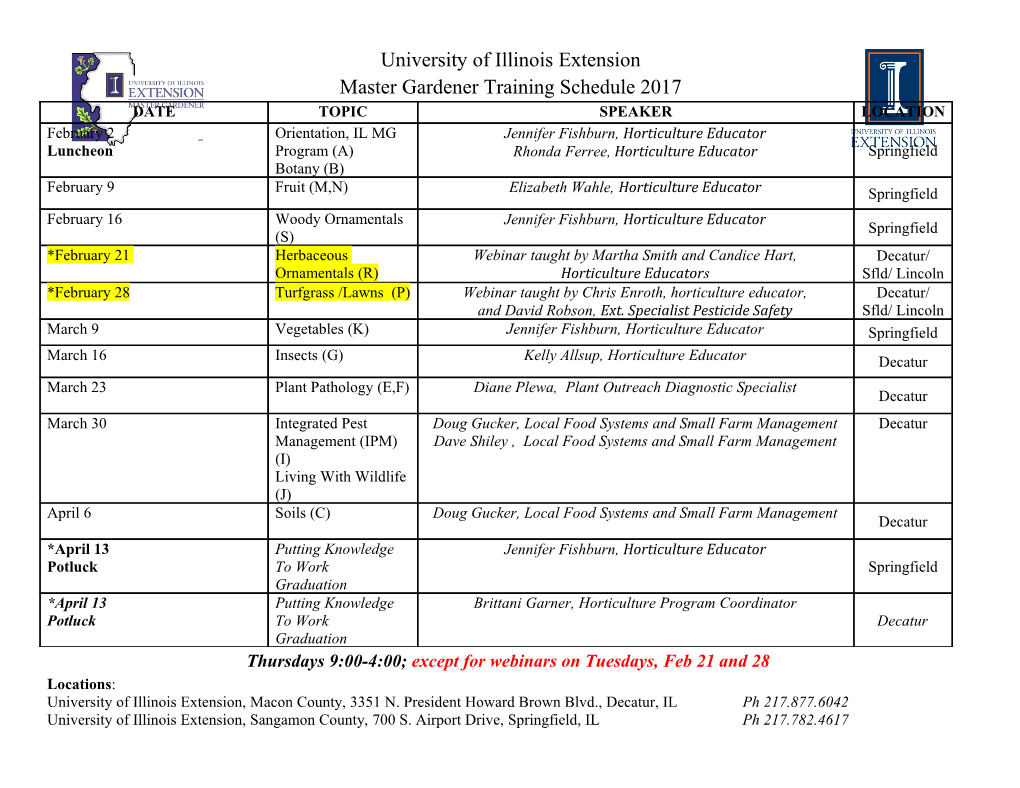
<p>Supplemental Material</p><p>Reduced capacity of alternative sigmas to melt promoters ensures stringent promoter recognition </p><p>Byoung-Mo Koo, Virgil A. Rhodius, Gen Nonaka, Pieter L. deHaseth, and Carol A. Gross</p><p>Supplemental Methods</p><p>Plasmid construction Derivatives of plasmid pSAKT28 and pSAKT32 encoding 28 or 32 variants were used for expression of 28 or 32 to determine their activities in vivo (Koo et al. 2009a; Koo et al. 2009b). All 28 or 32 variants were constructed by site-directed mutagenesis. Derivatives of pQF50K carrying promoter fragments cloned in the BglII-XbaI sites upstream of lacZ were used for measuring promoter strength and activities of ’s (Wang and deHaseth 2003). Promoter mutant derivatives were constructed using PCR by amplifying the promoter regions using mutagenic primers that encompassed either the BglII or XbaI site. Derivatives of the pET21a vector (Novagen) encoding 28, 32 and their variants were used to overexpress proteins for purification. The vectors were constructed using PCR by amplifying from the pSAKT28 and pSAKT32 series using primers to create flanking NdeI and HindIII sites and inserted as NdeI-HindIII fragments into pET21a</p><p>NdeI-HindIII vector. The resultant s contain an N-terminal His6-tag and PreScission cleavage site (Patikoglou et al. 2007). . -galactosidase assay The assay systems for in vivo promoter expression studies were constructed by transforming derivatives of pSAKT and pQF50K into appropriate strains sequentially using electroporation (for 32) or heat-shock (for 28). Overnight cultures in LB media with 100 g/ml ampicillin and 50 g/ml kanamycin (and 0.1% L-(+)-arabinose for CAG57101) grown at 30°C were diluted 1:100 and grown for 2hr at 30˚C and either induced with 1 mM IPTG (experimental) or not (control). Cell growth was stopped after 2hr for 28 and 3hr for 32 and -galactosidase assays were performed as described previously (Miller 1972). -galactosidase activity (in Miller Units) was determined using</p><p>1 the following equation: Units = 1000 x [(OD420 – 1.75 x OD550) / (incubation time in minutes x volume of the culture used in the assay in ml x OD600 of the culture used in the assay)] </p><p>Overproduction and purification of s s were overproduced from BL21/pLysS strain harboring pET21a derivatives. Overnight cultures were diluted 1:100 into fresh media containing 100 g/ml ampicillin and 30 g/ml chloramphenicol, and grown aerobically at 30 °C. When the cultures reached 28 32 OD600 of 0.4, expression of or was induced by addition of IPTG to a final concentration of 1 mM and growth continued at 30 °C with aeration for 2 hr. 500 ml of each culture was then harvested by centrifugation and stored at - 80 °C until purification. For purification of 28,the cell pellets were resuspended in buffer A1 (20 mM HEPES, pH 8.0, 300 mM KCl, 1 mM -mercaptoethanol, 5 mM Imidazole, 1 mM AEBSF). The cells were disrupted by sonication and the insoluble fraction was pelleted by centrifugation at 5,000 X g for 10 min and resuspended in 10 ml of buffer A2D (20 mM HEPES, pH 8.0, 300 mM KCl, 1 mM -mercaptoethanol, 5 mM Imidazole, 6 M guanidine hydrochloride). The 28 proteins were purified from the supernatant fraction by metal affinity chromatography using Talon metal affinity resin (Clontech) in denaturing condition but proteins were eluted in native condition by omitting guanidine hydrochloride. The N- terminal His6-tag was removed using PreScission protease (GE Healthcare) during dialysis into buffer Q (10 mM Tris, pH 7.5, 50mM NaCl, 1mM DTT) at 4 °C for 16 hr. The sample was further purified by a second, subtractive metal affinity chromatography step to remove uncleaved His6-’s and the His6-tag, followed by ion exchange chromatography (HiTrap Q Sepharose; GE Healthcare). As a final step, protein fractions were further purified by gel filtration chromatography (Superdex 75; GE Healthcare) in order to remove residual contaminants and free 28 fractions were taken. For purification of 32, the cell pellets resuspended in buffer A1a (20 mM HEPES, pH 8.0, 300 mM KCl, 1 mM -mercaptoethanol, 5 mM Imidazole, 0.66% Isoleucine, 0.83% Phenylalnine, 1 mM AEBSF). The cells were disrupted by sonication and the lysates</p><p>32 cleared of cell debris by centrifugation at 100,000 X g for 90 min. N-terminal His6- was purified from the soluble fraction by metal affinity chromatography using Talon metal affinity resin (Clontech). The N-terminal His6-tag was then removed using PreScission protease (GE Healthcare) during dialysis into buffer Q (10 mM Tris, pH 7.5, 50mM NaCl, 1mM DTT) at 4 °C for 16hr. The sample was further purified by a second, subtractive metal affinity chromatography step to remove uncleaved His6-s and the His6-tag, followed by ion exchange chromatography (HiTrap Q Sepharose; GE Healthcare). As a</p><p>2 final step, DnaK bound 32 was removed by gel filtration chromatography (Superdex 75; GE Healthcare) and free 32 fractions were taken. Purified proteins were concentrated in buffer B (20 mM Tris, pH7.5, 200 mM KCl, 1 mM</p><p>DTT, 0.01% Triton X-100, 50% glycerol). Purity of all His6-tag free s were >95% judged by SDS-PAGE and native-PAGE. Protein concentration was determined by the Bicinchoninic acid protein assay (Pierce).</p><p>In vitro transcription Single round run-off transcription was performed at 30 °C. Linear DNA templates were generated by amplification of promoter region of pQF50K derivatives with following primers; forward primer, IT-F, 5’- ACTCTCAGTACAATCTGCTCTGATG -3’ and reverse primers, IT-R-L, 5’- AAAGCGCCATTCGCCATTCAGGCTG -3’ and IT-R-S, 5’- GCTATTACG CCAGCTGGCGAA AGG -3’. Primers IT-F and IT-R-L were used to generate control templates bearing wt groE promoter (420 bp: 220 nt upstream and 200 nt downstream of the transcription start site) from pQF50K derivatives. Short templates (355 bp: 220 nt upstream and 135 nt downstream of the transcription start site) were amplified from pQF50K derivatives with IT-F and IT-R-S and used for comparing transcription from wt or mutant promoters. RNAP core enzyme was purified as described in previous study (Sharp et al. 1999). Holoenzyme was reconstituted by incubation of core RNAP and a 3-fold excess of 32 or a 5-fold excess of 28 on ice for 30 min in 32 binding buffer (20 mM Tris pH8.0, 100 mM</p><p>KCl, 10 mM MgCl2, 0.1 mM EDTA, 1 mM DTT, 100 g/ml BSA, 5% glycerol and 0.05% Tween-20) or 28 binding buffer (20 mM Tris pH8.0, 100 mM K-acetate, 10 mM Mg- acetate, 0.1 mM EDTA, 1 mM DTT, 100 g/ml BSA, 5% glycerol and 0.05% Tween-20). Holoenzyme was diluted in binding buffer and pre-incubated at 30 °C for 5 min. After incubation of holoenzyme and DNA template at 30 °C for another 5 min, transcription was initiated by addition transcription mix and incubation at 30 °C for 6 min. The reaction mixture (9 l) contained 50 nM holoenzyme, 5 nM template DNA (2.5 nM of long template and 2.5 nM of short template), 200 M ATP, 200 M GTP, 200 M UTP, 10 M CTP, 100 nM [-32P]CTP (3000 Ci/mmol), and 100 g/ml heparin in binding buffer. Reactions were stopped by the addition of 7 l of gel loading solution containing 20 mM EDTA, 80% deionized formamide, 0.1% of bromophenol blue and xylene cyanol FF, and 35-nucleotide 32P end-labeled oligomer. After incubation at 90 °C for 2 min, 9 l of each sample was subjected to electrophoresis in a 6% acrylamide/7M urea/TBE gel. The transcripts were visualized and analyzed using Molecular Dynamics Storm 560 Phosphor-Imager scanning system and ImageQuant 5.2 densitometry software. Sizes of</p><p>3 transcripts from long and short template are 200 and 135 nucleotides respectively.</p><p>Promoter activity determined by expression of green fluorescence protein The 32 promoter library was constructed as previously described (Rhodius et al. 2006). Briefly, 50 32 promoters were cloned as XhoI-BamHI fragments into the green fluorescent protein (GFP) reporter plasmid, pUA66 (Zaslaver et al. 2004) upstream of the gene gfpmut2 (Cormack et al. 1996). The promoter fragments (from -65 to +20 with respect to the predicted transcription start point validated in our previous work (Nonaka et al. 2006) were generated using PCR by amplifying from genomic DNA with the upstream and downstream primers to create flanking XhoI and BamHI sites, respectively. Reporter strains were constructed by transforming derivatives of pSAKT32 (Table S1) and promoter library plasmids into CAG57101 sequentially using electroporation. Promoter assays were performed by direct inoculation of Luria broth supplemented with appropriate antibiotics from fresh transfomants. 150 l cultures were grown in covered 96-well U-bottom tissue culture plates overnight at 32 °C with shaking at 400 rpm. The cultures were then diluted 1:100 into fresh assay 96-well plates (Costar #3915, black with clear flat bottom covered with lid, Corning) containing Luria broth supplemented with appropriate antibiotics and 1 mM IPTG and incubated in a multimode microplate reader- incubator-shaker Varioskan spectrofluorometer (Thermo Electron Corporation) at 32 °C with shaking at 480 rpm, 6 mm orbital diameter. Repeated OD600 and GFP fluorescence measurements were performed every 30 mins. Fluorescence (relative fluorescence units or RFU) was determined by excitation at 481 nm and emission measured at 507 nm. </p><p>32 -dependent promoter activity was determined as follows: First, at each OD600 measurement the GFP-dependent fluorescence for CAG57101 cells expressing 32 and promoter::gfp construct was background-corrected by subtracting the fluorescence readings of CAG57101 cells expressing the same 32 variant but with a promoterless gfp construct. Next, the background-corrected values were plotted against OD600 and the slope of the linear portions of the differential rate plot was determined as promoter activity. Note that the rrnB P1 promoter is highly 70-dependent and the xerD promoter gave almost no activity; these promoters were excluded from further data analysis.</p><p>Electrophoretic Mobility Shift Assay All equipment used in this assay was pre-chilled at 4 ºC. PAGE-purified synthetic oligonucleotides were used for preparing double strand and fork junction probes. The sequences of non-template strand are (-</p><p>4 32 41)CCCCCTTGAAGGGGCGAAGCCTCATCCCCATTTCTCTGGTCA(+1) of promoter 28 (PgroE) and (-39)CAGCAATAAAGTTTCCCCCCTCCTTGCCGATAACGAGATC(+1) of promoter (Ptar). Templates with variable length or bearing base substitutions as indicated in Fig. 6 were modified from these two sequences. 32P-labeled 10 nM annealed DNA probe and 25 nM holoenzyme were mixed in 10 l of binding buffer (20 mM Tris pH8.0, 100 mM KCl, 10 mM MgCl2, 0.1 mM EDTA, 1mM DTT, 100 g/ml BSA, 5% glycerol, 0.05% Tween-20 and 10g/ml poly(dI-dC)) and incubated at 4 °C for 10 min. For heparin challenge, 2 l of 600 g/ml heparin was added and the incubation was continued for an additional 5 min. The electrophoresis was performed in a pre- chilled 5% acrylamide/TBE gel at 4 ºC. Labeled probes were visualized and analyzed using Molecular Dynamics Storm 560 Phosphor-Imager scanning system and ImageQuant 5.2 densitometry software.</p><p>5 Supplemental Table S1. Strains and plasmids used in this study</p><p>Strains/plasmids Relevant genotype Source/reference</p><p>Strains</p><p>E. coli</p><p>DH5 fhuA2 (argF-lacZ)U169 phoA glnV44 Φ80 Invitrogen (lacZ)M15 gyrA96 recA1 relA1 endA1 thi-1 hsdR17</p><p>- - BL21(DE3)pLysS dcm ompT hsdS(rB mB ) gal (DE3), pLysS, Invitrogen CmR</p><p>CAG22239 MG1655 lacX74 Lab collection</p><p>CAG57101 MG1655 lacX74, rpoH::aadA, (Koo et al. 2009a)</p><p>R R PBAD_groESL::cat Cm , Sp</p><p>CAG57115 MG1655 lacX74, fliA::aadA, flgM::cat (Koo et al. 2009b) CmR, SpR</p><p>Plasmids pBK131 32-I54N in pSAKT32, ApR (Koo et al. 2009a) pBK161 32-R2.3CCR in pBK131, ApR This study pBK162 32-R2.3CCR, K130A in pBK131, ApR This study pBK163 32-F104Y, H107W in pBK131, ApR This study pBK164 32-F104Y, H107W, K130A in pBK131, ApR This study pSAKT28 28 in pSAKT32, ApR (Koo et al. 2009b) pBK205 28-D81A in pSAKT28, ApR (Koo et al. 2009b) pBK206 28-R84A in pSAKT28, ApR (Koo et al. 2009b) pBK207 28-R91A in pSAKT28, ApR (Koo et al. 2009b) pBK221 28-Q73W in pSAKT28, ApR This study pBK222 28-Q73W, D81A in pSAKT28, ApR This study pBK223 28-Q73W, R84A in pSAKT28, ApR This study pBK224 28-Q73W, R91A in pSAKT28, ApR This study pBK300 32 in pET21a*, ApR (Koo et al. 2009a) pBK301 32-I54N in pET21a*, ApR (Koo et al. 2009a) pBK311 28 in pET21a*, ApR (Koo et al. 2009b) pBK312 28 –D81A in pET21a*, ApR (Koo et al. 2009b)</p><p>6 pBK313 28 –R84A in pET21a*, ApR (Koo et al. 2009b) pBK314 28 –R91A in pET21a*, ApR (Koo et al. 2009b) pBK321 28 –Q73W in pET21a*, ApR This study pBK322 28 –Q73W, D81A in pET21a*, ApR This study pBK323 28 –Q73W, R84A in pET21a*, ApR This study pBK324 28 –Q73W, R91A in pET21a*, ApR This study pBK343 32-I54N,F104Y, H107W in pET21a*, ApR This study pQF50K Vector, pMB1 ori, 1600 ori, promoterless lacZ, (Farinha and Kropinski 1990) KmR pQF50K_groE PgroE (-44 to -9, WT) in pQF50K, KmR (Wang and deHaseth 2003) pQF50K_groE_T-35G PgroE (T-35G) in pQF50K, KmR (Wang and deHaseth 2003) pQF50K_groE_A-33C PgroE (A-33C) in pQF50K, KmR (Wang and deHaseth 2003) pQF50K_groE_C-14A PgroE (C-14A) in pQF50K, KmR (Wang and deHaseth 2003) pQF50K_groE_T-11G PgroE (T-11G) in pQF50K, KmR (Wang and deHaseth 2003) pQF50K_groE_T-9C PgroE (T-9C) in pQF50K, KmR (Wang and deHaseth 2003) pBK501 PgroE (C-16A,C-15A) in pQF50K, KmR (Koo et al. 2009a) pBK506 PgroE (C-14A,C-13A) in pQF50K, KmR This study pBK521 PgroE (13bp spacer) in pQF50K, KmR This study pBK522 PgroE (15bp spacer) in pQF50K, KmR This study pBK601 Ptar (-44 to +10, WT) in pQF50K, KmR (Koo et al. 2009b) pBK608 Ptar (C-12A) in pQF50K, KmR (Koo et al. 2009b) pBK611 Ptar (G-11A) in pQF50K, KmR (Koo et al. 2009b) pBK614 Ptar (A-10C) in pQF50K, KmR (Koo et al. 2009b) pBK618 Ptar (G-14T, C-13T) in pQF50K, KmR (Koo et al. 2009b) pBK621 Ptar (9bp spacer) in pQF50K, KmR This study pBK622 Ptar (1bp spacer) in pQF50K, KmR This study pBK623 Ptar (12bp spacer) in pQF50K, KmR This study pBK624 Ptar (13bp spacer) in pQF50K, KmR This study pUA66 Vector, pSC101 ori, GFP reporter plasmid (Zaslaver et al. 2004) carrying GFPmut2, KmR pUA H1 PdnaK P1 (-65 to +20) in pUA66, KmR This study, (Nonaka et al. 2006) pUA H2 PdnaK P2 (-65 to +20) in pUA66, KmR This study, (Nonaka et al. 2006) pUA H3 PileS (-65 to +20) in pUA66, KmR This study, (Nonaka et al. 2006)</p><p>7 pUA H4 PhepA (-65 to +20) in pUA66, KmR This study, (Nonaka et al. 2006) pUA H5 PyadF (-65 to +20) in pUA66, KmR This study, (Nonaka et al. 2006) pUA H6 PyafD (-65 to +20) in pUA66, KmR This study, (Nonaka et al. 2006) pUA H7 PribE (-65 to +20) in pUA66, KmR This study, (Nonaka et al. 2006) pUA H8 PclpP (-65 to +20) in pUA66, KmR This study, (Nonaka et al. 2006) pUA H9 Plon (-65 to +20) in pUA66, KmR This study, (Nonaka et al. 2006) pUA H10 PhtpG P1 (-65 to +20) in pUA66, KmR This study, (Nonaka et al. 2006) pUA H11 PhtpG P2 (-65 to +20) in pUA66, KmR This study, (Nonaka et al. 2006) pUA H12 PybeD (-65 to +20) in pUA66, KmR This study, (Nonaka et al. 2006) pUA H13 PybeZ (-65 to +20) in pUA66, KmR This study, (Nonaka et al. 2006) pUA H14 PglnS (-65 to +20) in pUA66, KmR This study, (Nonaka et al. 2006) pUA H15 PyceJ (-65 to +20) in pUA66, KmR This study, (Nonaka et al. 2006) pUA H16 PyceP (-65 to +20) in pUA66, KmR This study, (Nonaka et al. 2006) pUA H17 PPhoP (-65 to +20) in pUA66, KmR This study, (Nonaka et al. 2006) pUA H18 PtopA (-65 to +20) in pUA66, KmR This study, (Nonaka et al. 2006) pUA H19 PyciS (-65 to +20) in pUA66, KmR This study, (Nonaka et al. 2006) pUA H20 PycjX (-65 to +20) in pUA66, KmR This study, (Nonaka et al. 2006) pUA H21 Pmlc (-65 to +20) in pUA66, KmR This study, (Nonaka et al. 2006) pUA H22 PydhQ (-65 to +20) in pUA66, KmR This study, (Nonaka et al. 2006) pUA H23 PgapA (-65 to +20) in pUA66, KmR This study, (Nonaka et al. 2006) pUA H24 PsdaA (-65 to +20) in pUA66, KmR This study, (Nonaka et al. 2006) pUA H25 PhtpX (-65 to +20) in pUA66, KmR This study, (Nonaka et al. 2006) pUA H26 PnarP (-65 to +20) in pUA66, KmR This study, (Nonaka et al. 2006) pUA H27 PclpB (-65 to +20) in pUA66, KmR This study, (Nonaka et al. 2006) pUA H28 PgrpE (-65 to +20) in pUA66, KmR This study, (Nonaka et al. 2006) pUA H29 PyfjN (-65 to +20) in pUA66, KmR This study, (Nonaka et al. 2006) pUA H30 PygaD (-65 to +20) in pUA66, KmR This study, (Nonaka et al. 2006) pUA H31 PygbF (-65 to +20) in pUA66, KmR This study, (Nonaka et al. 2006) pUA H32 PxerD (-65 to +20) in pUA66, KmR This study, (Nonaka et al. 2006) pUA H33 PyggV (-65 to +20) in pUA66, KmR This study, (Nonaka et al. 2006) pUA H34 PrpoD (-65 to +20) in pUA66, KmR This study, (Nonaka et al. 2006) pUA H35 PftsJ (-65 to +20) in pUA66, KmR This study, (Nonaka et al. 2006) pUA H36 PyhdN (-65 to +20) in pUA66, KmR This study, (Nonaka et al. 2006) pUA H37 PprlC (-65 to +20) in pUA66, KmR This study, (Nonaka et al. 2006) pUA H38 PmutM (-65 to +20) in pUA66, KmR This study, (Nonaka et al. 2006)</p><p>8 pUA H39 PibpA (-65 to +20) in pUA66, KmR This study, (Nonaka et al. 2006) pUA H40 PhslV (-65 to +20) in pUA66, KmR This study, (Nonaka et al. 2006) pUA H41 PyrfG (-65 to +20) in pUA66, KmR This study, (Nonaka et al. 2006) pUA H42 PyhgH (-65 to +20) in pUA66, KmR This study, (Nonaka et al. 2006) pUA H43 PfxsA (-65 to +20) in pUA66, KmR This study, (Nonaka et al. 2006) pUA H44 PgroES (-65 to +20) in pUA66, KmR This study, (Nonaka et al. 2006) pUA H45 PmutL (-65 to +20) in pUA66, KmR This study, (Nonaka et al. 2006) pUA H46 PmiaA (-65 to +20) in pUA66, KmR This study, (Nonaka et al. 2006) pUA H47 Phfq (-65 to +20) in pUA66, KmR This study, (Nonaka et al. 2006) pUA H48 PholC (-65 to +20) in pUA66, KmR This study, (Nonaka et al. 2006) pUA H49 PcreA (-65 to +20) in pUA66, KmR This study, (Nonaka et al. 2006) pUA H50 PrrnB P1 (-65 to +20) in pUA66, KmR This study, (Nonaka et al. 2006)</p><p>*: thrombin cleavage site is modified to PreScission protease cleavage site</p><p>9 Supplemental Figures Figure S1</p><p>Figure S1. Comparison of in vitro transcription by either wt and Q73W28 . Single round run-off transcription assays were performed as described in Supplemental methods; each experiment was repeated a minimum of three times and representative gels of each transcription reactions are shown. A. Transcription of the wt tar promoter by 28 variants with mutations that eliminate promoter recognition determinants constructed either in wt or Q73W28. T; transcript from tar promoter driven by different 28 variants. E; end-labeled 35 nucleotide oligomer (serves as a loading control). B. Comparison of effect of wt andQ73W28 on transcription from non-optimal tar promoters in vitro. The upper band in each lane is from the wt tar promoter (internal control); the lower band originates either from the wt tar promoter or the mutant to be tested.</p><p>10 Figure S2.</p><p>Figure S2. Promoter activities driven by either wt or YW32 were determined by the expression of GFP from natural 32 promoters. Assay was performed as described in Supplemental methods. Promoter activity was calculated from the slope of the differential plot of OD600 vs GFP fluorescence (RFU), see Supplemental methods. Activities of rrnB P1 and xerD promoters are not shown in this graph as mentioned in Supplemental methods. All values are averages of three independent experiments; error bars indicate one standard deviation.</p><p>11 Figure S3.</p><p>Figure S3. 32 and 28 variants do not bind DNA probes bearing their anti-10 promoter sequence. Electrophoretic mobility shift assay (EMSA) was performed essentially as described in Supplemental methods but using DNA probes bearing the anti -10 promoter</p><p>32 28 sequence for (A) or (B). The sequences of non-template strand are (-</p><p>41)CCCCCTTGAAGGGGCGAAGCCTCATGGGGTAAACTCTGGTCA(+1) of anti-10 groE promoter and (-39)CAGCAATAAAGTTTCCCCCCTCCTTCGGCTATTCGAGATC(+1) of anti-10 tar promoter (anti-10 sequences are underlined). Binding assays are shown in groups of 3 (core RNA polymerase; wt holoenyzme; holoenzyme with enhanced melting to the following templates: long and short duplex template without heparin; long and short duplex template with heparin; long and short fork junction template with heparin. Representative gels are shown. Almost no binding is observed with any template; the weak bands that indicate binding are also present in the core RNA polymerase control indicating that these bands represent nonspecific binding to DNA. A. Binding of RNAP containing wt or YW32 to duplex or fork anti -10 groE promoter DNA. B. Binding of RNAP containing wt or Q73W28 to duplex or fork anti-10 tar promoter DNA. </p><p>12 Figure S4.</p><p>Figure S4. Sequence logos denoting sequence conservation within Region 2.3 of Group 1, 2 s (A) and Group 3 s (B). Sequences of classified s were adopted from our previous review (Gruber and Gross 2003). 134 of Group 1, 2 s and 96 of Group 3 s are aligned. The aligned sequences were visualized using sequence logo (http://weblogo.berkeley.edu) (Crooks et al. 2004). Sequence logos of the position corresponding to that of E. coli 70 from 425th to 434th residue are shown.</p><p>13 Supplemental references</p><p>Cormack, B.P., Valdivia, R.H., and Falkow, S. 1996. FACS-optimized mutants of the green fluorescent protein (GFP). Gene 173: 33-38. Crooks, G.E., Hon, G., Chandonia, J.M., and Brenner, S.E. 2004. WebLogo: a sequence logo generator. Genome Res 14: 1188-1190. Farinha, M.A. and Kropinski, A.M. 1990. Construction of broad-host-range plasmid vectors for easy visible selection and analysis of promoters. J Bacteriol 172: 3496-3499. Gruber, T.M. and Gross, C.A. 2003. Multiple sigma subunits and the partitioning of bacterial transcription space. Annu Rev Microbiol 57: 441-466. Koo, B.M., Rhodius, V.A., Campbell, E.A., and Gross, C.A. 2009a. Dissection of recognition determinants of Escherichia coli sigma32 suggests a composite -10 region with an 'extended - 10' motif and a core -10 element. Mol Microbiol 72: 815 - 829. -. 2009b. Mutational analysis of Escherichia coli sigma 28 and its target promoters reveals recognition of a composite -10 region, comprised of an 'extended -10 motif' and a core-10 element. Mol Microbiol 72: 830 - 843. Miller, J.H. 1972. Experiments in Molecular Genetics. Cold Spring Harbor Laboratory Press, Cold Spring Harbor, NY. Nonaka, G., Blankschien, M., Herman, C., Gross, C.A., and Rhodius, V.A. 2006. Regulon and promoter analysis of the E. coli heat-shock factor, sigma32, reveals a multifaceted cellular response to heat stress. Genes Dev 20: 1776- 1789. Patikoglou, G.A., Westblade, L.F., Campbell, E.A., Lamour, V., Lane, W.J., and Darst, S.A. 2007. Crystal structure of the Escherichia coli regulator of sigma70, Rsd, in complex with sigma70 domain 4. J Mol Biol 372: 649-659. Rhodius, V.A., Suh, W.C., Nonaka, G., West, J., and Gross, C.A. 2006. Conserved and variable functions of the sigmaE stress response in related genomes. PLoS Biol 4: e2. Sharp, M.M., Chan, C.L., Lu, C.Z., Marr, M.T., Nechaev, S., Merritt, E.W., Severinov, K., Roberts, J.W., and Gross, C.A. 1999. The interface of sigma with core RNA polymerase is extensive, conserved, and functionally specialized. Genes Dev 13: 3015-3026. Wang, Y. and deHaseth, P.L. 2003. Sigma 32-dependent promoter activity in vivo: sequence determinants of the groE promoter. J Bacteriol 185: 5800-5806.</p><p>14 Zaslaver, A., Mayo, A.E., Rosenberg, R., Bashkin, P., Sberro, H., Tsalyuk, M., Surette, M.G., and Alon, U. 2004. Just-in-time transcription program in metabolic pathways. Nat Genet 36: 486-491.</p><p>15</p>
Details
-
File Typepdf
-
Upload Time-
-
Content LanguagesEnglish
-
Upload UserAnonymous/Not logged-in
-
File Pages15 Page
-
File Size-