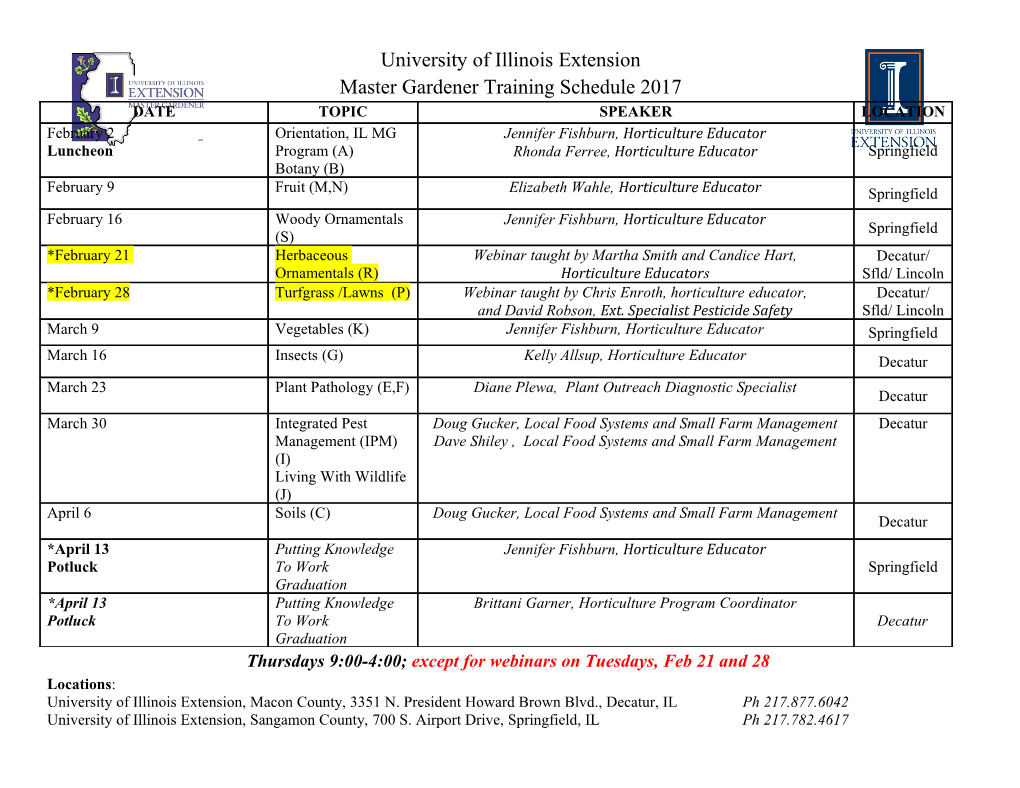
<p> Hydrologic Cycle—NOTES</p><p>Slide 2 – Hydrologic Cycle The hydrologic cycle begins with the evaporation of water from the surface of the ocean. As moist air rises into the atmosphere, it cools and water vapor condenses to form clouds. Moisture is transported around the surface of the earth until it returns to the surface as precipitation (rain, snow, etc.). Once the water reaches the ground a small portion of the water will evaporate back into the atmosphere, a small portion will infiltrate directly into ground water, and majority of the rainfall will runoff into streams, lakes, ponds, etc. Streams, rivers, and lakes are primary sources of ground water recharge (and discharge). Groundwater seeps its way into lakes, rivers, and streams, and is released back into the atmosphere through transpiration. The balance of water remaining on the earth's surface is called runoff, which drains into lakes, rivers and streams and eventually carried back to the oceans, where the cycle begins again. Slide 3 – Water Budget The earth’s supply of water is stored in the oceans (97.2%) of the earth's water, land 2.799%, and the atmosphere holds less than .001%. Of the water stored on land 2.15% is frozen water (ice caps) and 0.65% is fresh water. Of the available freshwater 97.5%, or 0.633% of the total supply of water is stored in groundwater, and only 0.016% of the earth’s water budget is available for wildlife and human use. The annual precipitation for the earth is more than 30 times the atmosphere's total capacity to hold water. This indicates there is a rapid recycling of water occurring between the earth's surface and the atmosphere. Slide 4 – Hydrologic Cycle - The Hydrologic Cycle. Illustration by Tom Schultz In the hydrologic cycle, approximately 80% of all evaporation is from the oceans, with the remaining 20% coming from inland water, soils and vegetation. Winds transport the evaporated water globally, influencing the humidity of the air throughout the world. For example, a typical hot and humid summer day in the Midwestern United States is caused by winds blowing tropical oceanic air northward from the Gulf of Mexico. Another example is how humid it feels in Las Vegas, which has a base summer humidity level of 12%, after a summer thunder shower as the rain quickly evaporates back into the atmosphere. Water vapor is a gas. Humidity is the amount of water vapor in the area. The dew point is the temperature at which the air becomes saturated and forms clouds. Fog is a cloud that touches the ground. Water is transferred from the surface to the atmosphere through a process called evapotranspiration. Evapotranspiration (ET) is the sum of evaporation from water bodies, moisture escaping from vegetation via plant transpiration and from soil. Vegetation type and land use can significantly affect evapotranspiration rates within a watershed. Plants absorb soil water through their roots. Transpiration is the release or evaporation of that water into the atmosphere from the leaves and stems of plants. Transpiration accounts for approximately 10% of all evaporating water. Absorption of water by a plants root system can originate from deep in the soil. For example, some desert plants have roots that extend 20 meters into the ground. Changes in climatic conditions and lower and raising the water table will effect distribution and structure of plant communities. For example, sagebrush has an open root system. This means they are constantly absorbing water. If the water table rises and/or if an area is flooded for any length of time the sagebrush will drown. The opposite effect can also occur. If precipitation decreases and/or if the water table is lowered new plant species will invade the area. Perfect example is the Owens Valley, CA. Long term dewatering of the area has resulted in Mojave Desert plant communities moving further north up into the Owens Valley, which under normal conditions would not have occurred. Condensation is the alteration of water from its gas form (water vapor) into liquid water. Condensation occurs in the atmosphere (e.g., bathroom shower walls/windows, ice water class) when warm air rises, cools, the surrounding air mass becomes saturated and looses its capacity to hold water vapor. The excess water vapor condenses to form cloud droplets. In the hydrologic cycle, the transport of moisture is visible as clouds. Clouds consist of ice crystals and/or very small water droplets. Clouds are propelled from one place to another by global hemispheric gyres, the jet stream, and earth surface-based circulations like land and sea breezes. A typical 1 kilometer thick cloud contains approximately one millimeter of rainfall. The amount of moisture in the atmosphere is usually 10-50 times greater by volume. Groundwater is a summation of all the water penetrating the earth's surface. As water penetrates the soil surface it travels through the zone of aeration or unsaturated zone. The gaps or pore space in the zone of aeration is filled mostly with air. Below the zone of aeration is the zone of saturation. In the zone of saturation the gaps and fractures are filled with water. The top of the saturated zone is called the water table. As the quantity of groundwater water increases or decreases, the water table rises or falls accordingly. When the area below the soil surface is saturated flooding occurs. Lakes and streams are expressions of where ground water is exposed at the land surface. The amount of water that can be held in the soil is called porosity. The rate at which water flows through the soil is its permeability. Different soil types hold and absorb water at different rates. As surface permeability decreases there is an increase amount of water remaining on the surface. Increased surface water results in increased rate of runoff creating a greater potential for flooding. As water seeps/infiltrates into the soil it may encounter an impermeable layer (i.e., clay, rock), or aquifers, and then travels laterally. An unconfined aquifer is where the water table is exposed to the earth's atmosphere through the zone of aeration. A confined aquifer occurs between zones or layers of impermeable strata. A free flowing artesian system occurs where the water table is above the well or spring and pressure force the water to be free flowing. Groundwater returns to the surface water bodies (lakes, streams, etc.) through these aquifers. In cases where the water table intersects the land surface, springs may occur. Springs are discharges of ground water to the land surface. Groundwater flow rates are in centimeters per day, meters per year, or centimeters per year. Surface runoff is movement of water, from rain, snowmelt, or other sources, flowing over the land surface. A watershed is a land area producing runoff flowing to a common point. Water is an excellent naturally occurring solvent. Its capable of scrubbing the atmosphere clean of particulate matter and aerosols, and absorbing soil contaminants (trace metals, petroleum, pesticides, fertilizers, etc.). Slide 5 – Hyporheic Zone The hyporheic zone is a region beneath and lateral/adjacent to the stream bed, where there is mixing of shallow groundwater and surface water. The flow dynamics, or hyporheic flow is important for surface water/groundwater interactions, as well as fish spawning, and benthic macroinvertebrate distribution and community structure. The mechanism of hyporheic flow can be triggered by stream water percolation, or the groundwater upwelling seepage beneath the stream bed and alongside the stream banks. Slide 6 – Hyporheic Zone (cont.) Water bodies are described as being lotic (flowing, moving) or lentic (standing or slow moving). In a lotic (flowing) stream system there are 2 types of flow – Rheic flow – or surface flow – i.e., visible free running water, and hyporheic percolating flow of water through the streams sides and bottom substrate (i.e., sand, gravel, cobbles, etc). Therefore, streams can be viewed as having 3 dimensional flow dynamics (along the path of the stream, down into the substrate, and into the sides/banks). The Hyporheic Zone is the area below the stream bed where water flows/percolates in the interstitial space between the sand, gravel, cobbles, etc. Hyporheic exchange between surface water and groundwater is unique because the stream water entering the subsurface (hyporheic zone) can mix with groundwater and eventually reenter the stream (rheic) at some point downstream. As water moves from the surface into groundwater and back again streams are said to be gaining or losing. In a gaining stream reach the ground water level is above the level of the base of the stream . Groundwater discharges into the stream from bank/streamside seeps or direct upwelling through the streambed. This also is true for lentic systems (lakes, wetlands, ponds, etc.) receiving groundwater discharge. For example, in arid regions and during dry periods, half or more of the total stream flow can come from groundwater discharge called “baseflow.” In losing stream reaches there is a net loss of water into groundwater through the hyporheic zone and stream banks. In losing stream reaches the water table in the stream is above the water level in the aquifer . For lentic systems water from lake, wetland, etc. recharges the groundwater. An example of a losing systems is groundwater drawn down (i.e., cone of depression) from a drinking water well and water from a lotic and/or lentic system filling the evacuated space. Water in the hyporheic zone moves at a much slower rate than water in the stream channel. This is a result of shear stress in the interstitial pore space. The exchange of water through the hyporheic zone increases the residence time of water within a stream system. The smaller the interstitial pore spaces (fine grained sediment) the longer the residence time and the exchange of water through the hyporheic zone. Hyporheic flow paths can be further divided into short (centimeter scale) to long (meter scale). Short hyporheic flow paths are a result of the composition of the streambed (grainsize), and/or streambed roughness features (sediment morphological features). Sediment morphological features are sand waves, mega-ripples, and ripples. Streambed composition is defined by substrate size, or grainsize – mud, silt, sand, pebbles, gravel, cobble, boulders, bedrock. Long hyporheic flow paths (meter to several meter scale) are influenced by larger stream geomorphic features that exist within the stream reach, such as pool–riffle reach sequences, stream meanders, point bars, boulder, and debris (e.g., tree stumps, logs, shrubs, etc.). Biogeochemical Influences- Filtering, Biological Community All chemical elements found in the earth’s biological organisms are part of biogeochemical cycles. A biogeochemical cycle is a circuit or pathway that defines the fate and transport of elements (organic, inorganic) through the biotic (bio) and abiotic (geo) parts of an ecosystem. It can also be described as how an ecosystems function, or ecological function. These global cycles (hydrologic cycle, sedimentary cycle, nitrogen cycle, sulfur cycle, carbon cycle, oxygen cycle, phosphorus cycle, hydrogen cycle) include the circulation of elements and nutrients on which life and the earth's climatic condition depend. Using atmospheric and oceanic circulation patterns, climatic conditions influence the spatial distribution and community structure of biological life forms by regulating the flow of essential elements (e.g., oxygen) through the earth’s ecosystems. All chemical elements occurring in organisms are part of the biogeochemical cycles. In addition to being a part of living organisms, these chemical elements also cycle through abiotic factors of ecosystems such as water (hydrosphere), land (lithosphere), and the air (atmosphere). The hyporheic zone can be considered the boundary layer, or transition zone, or mixing zone between surface and groundwater. Therefore, hyporheic water in this transition zone is a mixture of surface water and groundwater. Geochemically, groundwater in comparison to surface stream water, on average, is lower in dissolved oxygen and enriched in inorganic solutes (salts). The hyporheic zone is a very active region for the biogeochemical transition of nutrients and other dissolved solutes. The hyporheic zone is where much of the water purification occurs by microbial processing of organic material, and by the uptake of riparian vegetation. Microbial activity may take up and/or modify incoming nitrogen compounds through nitrification and denitrification processes as part of their primary productivity. Biochemical oxygen demand (BOD) is a measure of the quantity of oxygen used by these micro-organisms in the aerobic oxidation of organic matter. Denitrification is the conversion of dissolved nitrate (NO3) into nitrogen gas (N2). The denitrification process generally occurs in anoxic sediments (sediments without oxygen). The spatial variations in streambed morphology and composition (sediment permeability) results in patchy streambed types (e.g. mud-sand, sand-gravel, gravel-cobble, etc.). These different patches will result in differing surface-subsurface hydrological exchange rates. Biogeochemical processes and benthic macroinvertebrate assemblages will vary among these patch types. This variation is a result of the flux in the exchange of surface-subsurface water, which determines the supply of organic matter and inorganic solutes. The flux in the exchange rate impacts the spatial distribution and composition of the benthic aquatic community. Coliform Bacteria (Fecal Coliform Bacteria) is naturally present in warm-blooded mammal (human, animal) and bird digestive tracts and feces. Coliform bacteria should not be found in well water or any other sources of drinking water. The presence of coliform bacteria in water is an indication of sewage or fecal contamination. Slide 7 – Ecological Function Natural riparian-wetland areas are characterized by the interactions of vegetation, soils, and hydrology. Riparian-wetland areas are functioning properly when adequate vegetation, landform, or large woody debris is present to dissipate stream energy associated with high waterflows, thereby reducing erosion and improving water quality. Changes in ecological function result from alterations (e.g., fire, invasive species, anthropogenic activity). Human Influences accelerate changes in ecosystem character. Over time, ecosystems develop spatial patterns characteristic of human disturbance and alterations. It is necessary than to recognize the different geographic patterns and how they respond to alterations and resource management activities. Differences between crop field water dynamics and a riparian buffer water dynamics. Arrow widths represent comparable amounts of water. Illustration by Tom Schultz. www.buffer.forestry.iastate.edu/Photogallery/... Slide 9 – Proper Functioning Condition Proper Function Condition (PFC) is a methodology for assessing the physical functioning of riparian and wetland areas. The term PFC is used to describe both the assessment process, and a defined, on-the-ground condition of a riparian-wetland area. In either case, PFC defines a minimum or starting point. Function is determined by assessing the physical functioning of riparian-wetland areas through consideration of hydrology, vegetation, and soil/landform attributes, and how well the physical processes are functioning. PFC is a state of resiliency that will allow a riparian wetland system to hold together during a 25 to 30 year flow event, sustaining that system's ability to produce values related to both physical and biological attributes. Reference: http://www.mountainvisions.com/Aurora/pfc.html PFC isn’t: The sole methodology for assessing the health of the aquatic or terrestrial components of a riparian-wetland area. PFC isn’t: A replacement for inventory or monitoring protocols designed to yield information on the "biology" of the plants and animals dependent on the riparian-wetland area. PFC can: Provide information on whether a riparian-wetland area is physically functioning in a manner which will allow the maintenance or recovery of desired values, e.g., fish habitat, birds, or forage, over time. Riparian areas are designated as vegetated (i.e., green) zones/areas along lakes, wetlands, rivers, streams, creeks. Flowing water features such as rivers, streams, and creeks (also referred to as lotic habitat areas). Wetland areas are associated with standing water features such as bogs, marshes, wet meadows, and estuaries (also referred to as lentic habitat areas). Functional - Riparian and wetland riparian areas are functioning properly when adequate vegetation, landform, or large woody debris is present to dissipate stream energy associated with high water flows. Also, providing habitat for terrestrial and aquatic organisms. Functional at Risk - areas are functioning properly, but an existing soil, water, or vegetation attribute makes them susceptible to degradation. The trend is an assessment of apparent direction of change (e.g., upward or downward) in conditions either towards or away from the site potential or site stability. Trend is determined by comparing the present condition of the stream reach with other reaches within the same systems (i.e., reference condition), with previous photos, trend studies, inventories, other documentation, or personal knowledge. The lack of historical information on the condition of a site may lead to a “trend not apparent” assessment. Nonfunctional - areas do not contain sufficient vegetation, landform, or large woody debris to dissipate stream energy associated with high flows. Slide 10 – Impacts In the world of environmental science there are some very basic concepts. One of those concepts is Vannote’s River Continuum Concept, but a concept even more important is water has a purpose. When a rain drop falls to the ground it does something. It’s taken up by plants (transpiration), soaks into the ground (through the Vadose Zone into the groundwater), and when the ground becomes saturated it runs off to join the hydrology of the system/watershed. During this process it can move particles (mechanical) and collect chemicals (chemical). When land is devoid of its natural vegetation the hydrodynamics change. This means when that raindrop falls to the ground it no longer has something to absorb to. As the raindrop becomes part of the overland flow, with no vegetation to alter its path, that raindrop(s) picks up speed (i.e., power). With the increased power the overland flow it is able to pickup larger particles and transport them into the stream (mechanical). Net result is increased sedimentation into the stream, degraded soils, potential intrusion areas for invasive plant species and aquatic species in the stream because of the degraded conditions, etc. So what has this all to do with drinking water. If you get your drinking water from ground water the increased surface water, or overland flow, decrease the amount of time available to percolate into the groundwater. Thus, decreasing water quantity, and subsequently lowering the water table. Potential effect is increasing chemical constituents because of the loss of dilution. Another issue is downcutting/erosion – in the next email I will show some examples – which can disconnect the stream from the groundwater. Increased sedimentation can impact the stream hyporheic/groundwater connection by filling in the pore spaces. If you get drinking water directly from the stream the increased flow can readily transport constituents of concern into your source water. Habitat and biological diversity in aquatic systems (streams, wetlands) are closely linked. An evaluation of habitat quality is critical to any assessment of ecological integrity. Habitat (stream and riparian) assessment is defined as the evaluation of the structures of the surrounding physical habitat influencing the quality of water resources and the condition of the resident aquatic community. A habitat assessment includes an evaluation of the variety and quality of the stream substrate, channel morphology, bank structure, and riparian vegetation. Rosgen (1985, 1994) presented a stream and river classification system founded on the premise that dynamically-stable stream channels have a morphologic structure capable of providing appropriate distribution of flow energy. Rosgen (1994), further identifies eight major variables effecting the stability of channel morphology: - Channel width - Channel depth - Channel slope - Channel roughness - Flow velocity - Discharge - Sediment load - Grainsize distribution Alteration of any of these characteristics from the norm will affect the streams capability to dissipate energy for that system. This will result in accelerated rates of channel erosion. Stream physical habitat structural components that function to dissipate flow energy are: Sinuosity Roughness of bed and bank materials Presence of point bars (slope is an important characteristic) Vegetative conditions of stream banks and the riparian zone Condition and accessibility of the floodplain</p>
Details
-
File Typepdf
-
Upload Time-
-
Content LanguagesEnglish
-
Upload UserAnonymous/Not logged-in
-
File Pages7 Page
-
File Size-